Abstract
Static charge present on a filter contributes substantial error to low-level mass measurements. The measurement and sources of static charge are not well understood and this article presents a fundamental method of static charge measurement. As predicted by other researchers, triboelectric charging was found to generate significant static charge on a filter when using 2007 Diesel particulate matter (PM) measurement protocols. Measurements made using our method indicate that PM filters are rapidly and effectively neutralized by 210 Polonuim ( 210 Po) sources. The neutralization of charge occurred exponentially with characteristic time constants (the time it takes for the charge on the filter to decay by 63%) of 0.4 and 0.7 s, depending on the strength of the source. The experimental neutralization time constants were consistent with theory. The charge remaining on highly charged 47 mm Teflon and TX40 filters after 5 s of neutralization with year old 210 Po sources biased the true filter mass by <1 μg when the filters were weighed with a 1 μg resolution Cahn microbalance.
INTRODUCTION
A frequently used measurement in aerosol science is the gravimetric analysis of filters to determine the suspended particulate matter (PM) mass concentration. Gravimetric analysis is often a reference method and is used to assess the performance of other mass measuring instruments. Thus, it is expected that gravimetric measurements be accurate over a wide range, however, it is known that static charge decreases accuracy of gravimetric analysis (CitationWeil 1990), especially when filter loadings are low. Evidence of the static charge influence comes from weighing a single filter before and after it is neutralized: the apparent mass will always be different. This difference and its subsequent bias on accuracy is difficult to quantify or predict. Our research is motivated by a recent 90% decrease in the US Diesel PM standard, from 0.1 to 0.01 g (hp-hr)–1 or equivalently, from 0.134 to 0.013 g kWh–1, suggesting that the percent error due to static could increase significantly if not properly controlled.
Static charge refers to an excess or deficiency of electrons and is present on a filter before and after PM sampling. During a filter weighing, static charge on the filter induces image charges in the conducting areas of the balance and the filter is electrostatically attracted to the image charges. Additionally, charge present on insulating areas of the balance may attract or repulse the filter, depending on the charge polarity. The balance interprets these attractions as mass, which adds to the overall mass of the filter.
To reduce the influence of static charge, the filter is often “neutralized” before it is weighed but there is no widespread agreement on how to best neutralize a filter or how to measure its charge. In this work, we examined neutralization methods that rely on radioactive alpha-particle sources. A 500 microCurie (μCi) 210Polonium (210Po) alpha-emitting source is used frequently for filter neutralization and this source has a half-life of 138 days (in SI units the strength is 1.85 × 107 becquerel, Bq). The neutralization potential of each source is based on the number of alpha particles emitted per unit time (the activity) and common practice is to replace the source on a yearly basis when its activity has been reduced to 80 μCi.
One neutralization method is to pass a radioactive source over a filter laying in a glass Petri dish. This method fails to address the charge located on the underside of the filter. CitationChase et al. (2005) discussed neutralization best practices, proposed filter charging mechanisms, and recommended a device (Electrostatic Discharge Platform (EDP)) to remove most of the charge. The EDP neutralizes both sides of a filter using multiple 210Po sources placed an appropriate distance apart. CitationChase and Schamp (2007) reported the optimum distance (3.4 cm) for most effective neutralization.
The length of neutralization necessary to reduce static charge to acceptable levels that is reported in the literature is inconsistent. CitationEngelbrecht et al. (1980) found that 47 mm Nuclepore filters were insufficiently neutralized after 30 s of exposure and attributed a bias of up to 150 μg to static charge. CitationAllen et al. (1999) reported that even after 30 s of exposure to 4 to 6 210Po sources, Teflon filters could not be weighed without measurement errors, in some cases, exceeding 20 μg. These results seem to contrast the work of CitationLawless and Rhodes (1999), who reported that neutralization with alpha particles removed filter static to below balance detectable levels within “a few seconds,” assuming that the neutralizers used were less than 6 months old. CitationVaughan et al. (1989) neutralized glass fiber and PVC filters for “a few seconds” and obtained precisions of 1.2 to 1.4 μg. CitationKhalek (2006) obtained precisions <2.5 μg when filters were neutralized on one side for 30 s. CitationFeeney et al. (1984) observed a time-dependant build up of charge on filters and found it necessary to neutralize with 210Po filters multiple times before neutralization was sufficient. CitationKoistinen et al. (1999) and Allen et al. (2001) achieved precisions of <2 μg with 210Po neutralization.
While some authors reported the length of neutralization, neither the filter's charge nor the strength nor age of the 210Po sources were explicitly reported in any of the references examined making it difficult to judge the authority of the results. If results show significant variability and static charge is suspected, the best practice is to increase the amount of 210Po, lengthen the neutralization time or to investigate possible charging during handling. This problem is similar to that of the electrical neutralization of aerosols (CitationLui and Pui 1974) but whereas the static charge on a particle can be directly calculated or reasonably estimated, the charge on a filter exists for entirely different reasons and cannot be accurately predicted. What is clear from this discussion is that a quantitative method of static charge detection would be useful.
Our work is particularly relevant to recent EPA regulations that require engine manufacturers to neutralize a filter until a “charge” of less than ±2 VDC remains on the filter, as measured by an electrostatic monitor (40 CFR 86.1312-2007c). The number provided by this instrument is not a measure of charge. CitationChase and Schamp (2007) discussed significant shortcomings found in EPA's method. The authors discuss how an electrostatic monitor measures surface potential. The probe-to-filter distance must be known exactly to make a quantitative interpretation of the charge on the filter. Additionally, where on the filter should one measure the potential? It is likely that the charge is non-uniformly distributed and thus an entire mapping of the filter's surface (top and bottom) would be necessary to obtain a complete picture of the charge. The inadequacy of the electrostatic monitor method is another motivator of our work.
The objective of this article is to present a device that can measure the static charge on a filter. The device uses a charge amplifier to measure the charge that the filter induces in a Faraday cage. A number of experiments were conducted to evaluate our device and further assess the influence of static charge. Measurements were made of the suspected origin of static charge, triboelectric charging. The effectiveness of 210Po sources to neutralize filters was quantified by measuring the charge remaining on filters after neutralization. Neutralized filters were weighed with a microbalance and data analysis techniques were employed to determine if bias due to static charge was still present.
INSTRUMENT DESIGN
A Faraday cage was used to measure static charge. The combination of the Faraday cage, signal cables, and associated electronics is referred to as the charge measurement device or CMD. CitationKeithley (2004) generically described measuring static charge with a Faraday cup. CitationMurtomaa et al. (2005) designed a conceptually similar device for real-time aerosol charge measurements.
The Faraday cage, shown in , consists of an outer aluminum box that serves as a grounded, shielding electrode. The inner box is connected to the signal wire, a low-noise coaxial cable connected to a charge amplifier, Kistler model 504E. The two electrodes are separated by four insulating Teflon bars. The charge amplifier circuit is shown in . The capacitance of the signal cable (C2) and Faraday cage is inconsequential because the potential difference across the cable is always zero and the inverting input is kept at virtual ground. The output of the charge amplifier is a –10 to 10 V signal that is proportional to the induced charge. A wide dynamic range is achieved by varying the value of C1, thereby varying the proportionality constant and the charge amplifier's transfer function.
The ability of the charge amplifier to accurately measure static charge was evaluated using a built-in 1000 ± 5 picoFarad (pF) capacitor. A calibration curve was generated by varying a DC voltage (V) across the capacitor (C), resulting in a charge (Q = C·V) present at the charge amplifier input. The output voltages were measured with a Hewlett-Packard 34401A digital voltmeter and converted to charge. The expected charge error was calculated by propagating the error in charge and voltmeter reading. The measured charge error was calculated by taking the standard deviation of ∼50 consecutively taken data points. The results of this accuracy check are shown in . The charge amplifier's response is linear (slope = 1) and perfectly correlated (r2= 1.0). The charge error bars are negligibly small and the experimental data is not corrected with the least-squares regression line.
EXPERIMENTAL METHODS
Basic Charge Measurement
To measure the charge on a filter the operator inserts the filter (held by a forceps) into the inner electrode and holds it there while the charge amplifier voltage is recorded. National Instruments hardware and Labview® software was used to collect the data, which was sampled at 10 Hz. A characteristic of charge amplifiers is their internal time constant. For static measurements, the time it takes to insert the filter in the Faraday cage must be short compared to the time it takes for the charge to bleed away. The decay of charge from the charge amplifier is governed by
Charge Measurement Noise
The CMD is an open-ended device that is sensitive to moving charge outside of the device. The CMD interprets this charge as filter charge as the filter is inserted into the Faraday cage. This charge can be made small and is measurable. shows a representative, simulated charge measurement where the motions that would occur when inserting a filter in the Faraday cage are replicated but no filter is carried. In this example, the motion was repeated 7 times; the induced “noise” charge of ∼0.4 pC must have been charge present on the CMD operator. The average noise charge is subtracted from the measured filter charge and the lower limit of detection is ∼1 pC due to the uncertainty in this correction.
To minimize the noise correction all insulating plastic objects must be removed from the measurement. Plastic acquires surface charge that negatively influence the result. The forceps cannot include a plastic finger rest. A grounded, non-insulated wrist strap must be used. It is also common practice to wear disposable plastic gloves while weighing filters. This practice is not advisable for static charge measurements or during filter weighing because the plastic insulates the forceps and may allow charge to transfer from the gloves to the filter. These suggestions were followed for our measurements.
Filter Neutralization
Many different experiments were performed where a neutralization treatment was applied to a filter. For each neutralization, we used the Duostat™ BF-1000 (NRD LLC) to position two 210Po sources the recommended distance from the filter (3.4 cm). The filter was manually held between the sources and was horizontally and vertically centered.
Filter Charging Mechanisms
Three suspected origins of static charge are: triboelectric charging by a glass Petri dish, triboelectric charging through contact with a filter cassette, and charge deposition/generation during sampling. Two experiments were conducted to determine the magnitude of the triboelectric charging events. For each experiment, 25 (of each) 47 mm in diameter Teflon with perfluoralkoxy (PFA) support ring (“Teflon”) and Pallflex TX40H120-WW (“TX40”) filters were used. In the first experiment, each filter was neutralized for 1 min using two 430 μCi 210Po sources after which each filter was set in a glass Petri dish where it remained for 1 min. The filter's charge was measured after it was removed from the dish. In the second experiment, each neutralized filter was loaded into and out of a filter cassette and the resulting charge was measured. The cassettes used in all experiments were ABS plastic and prescribed for use with 2007 US Diesel PM measurements.
Particles in Diesel and gasoline engine exhaust have been measured to have a net neutral charge (CitationKittelson et al. 1986; CitationMaricq 2006). Filter fibers can be charged by friction when air passes through the filter, which may lead to the enhanced collection of charged particles. The net charge due to sampling is expected to be small compared with the charge generated by handling. Handling is an inevitable part of sampling, thus, our methods are unable to directly measure the charge generated or captured during sampling.
A filter can be charged by other mechanisms: dropping it on the floor, bringing into the vicinity of a highly charged object, etc. It was not possible to investigate all possible sources of charging due to poor practices in this work and it is the responsibility of the weighing technician to ensure filters are appropriately handled.
Filter Neutralization Time
Teflon and TX40 filters were used in a series of experiments designed to determine the time required to neutralize highly charged filters. The experiments were performed in a temperature (22 ± 1°C) and humidity (50 ± 4% RH) controlled weigh room, using the following procedure to measure the decay of charge from each filter.
1. | Charge filter with cassette holder—measure charge on filter | ||||
2. | Neutralize filter using two 210Po sources for 1 s—measure charge on filter | ||||
3. | Neutralize filter for 1 min to remove almost all charge | ||||
4. | Charge filter with cassette holder | ||||
5. | Neutralize filter using two 210Po sources for 2 s—measure charge on filter |
Theoretical Analysis of the Neutralization Time
Following the analysis of CitationChase and Schamp (2007), a theoretical calculation of the neutralization time is developed to later compare with the experimental measurements. The electric field (E) due to the charged filter is modeled as a charged sheet of infinite dimensions, with a magnitude of
Influence of Static Charge on Filter Mass Measurements
Two experimental methods are used to demonstrate different methods of detecting charge with data analysis and to determine if 5 s of neutralization would decrease the influence of static charge on TX40 and Teflon filters to <1 μg when the filters were weighed with a 1 μg resolution Cahn 31 microbalance. The weigh room was temperature (21.5 ± 0.25°C) and humidity (50 ± 1 %RH) controlled.
Static Charge Detection: Method 1 (TX40 Filters)
In method 2, a number of similar filters are weighed multiple times and each filter is neutralized between weighings. The average mass of each filter is calculated. The average (for all filters) of the mass minus the average mass (for each individual filter) is plotted as a function of the number of weighing events. If this average decreases with the weigh number, it must be that static change is being progressively removed, the length of neutralization is insufficient, and the average filter mass is biased high. In this work, 10 filters were charged with the cassette holder and neutralized/weighed 6 times. The length of neutralization between each weighing, in seconds (strength of source, μCi), was 5 (80), 5 (235), 10 (235), 10 (235), 10 (235), and 10 (235).
Static Charge Detection: Method 2 (Teflon Filters)
Method 2 assumes that 1 min of neutralization with two 430 μCi sources is sufficient to remove all detectable charge. Thus, weighing a filter after this length of neutralization provides a “true” measure of the filter's mass. The average difference between the mass of 5 highly charged Teflon filters neutralized for 5 s with 80 μCi or 235 μCi 210Po sources and the “true” mass of each filter is calculated.
RESULTS
Filter Charging Mechanisms
Filter contact with the Petri dish and filter cassette resulted in significant static charge gains on the filter and a summary of the results can be seen in . The more abrasive filter cassette charging is 3 times stronger than the Petri dish charging. On average, Teflon filters are negatively charged more than the TX40 filters by an order of magnitude. This difference can be explained by the triboelectric relationships between materials. For example, when the TX40 filter is placed in the Petri dish the glass fiber-glass contact does not produce much charge whereas the Teflon-glass contact is optimum for triboelectric charging. Glass readily losses electrons and Teflon willingly accepts them.
Filter Neutralization Time
compares the exponential decay curves computed with Equation Equation4 with the experimental measurements. The results show that the filters are neutralized rapidly and the experimental time constants are consistent with theory (within experimental and theoretical uncertainty). The results demonstrate that using an older (less potent) source increases the time required for sufficient neutralization. These experiments were repeated with TX40 filters (not shown). The significantly lower initial charge and rapid decay of charge made robust measurements of the time constant for these filters impossible.
FIG. 6 Theoretical (dotted lines) and experimental (solid circles and solid regression line) Teflon filter neutralization curves for (a) 430 μCi (r2= 0.95) and (b) 200 μCi (r2= 0.80) 210Po sources. The two dotted lines in each figure are produced using Equation (Equation4). The difference between the lines is in the assumed mobility (1.2 or 1.9 cm2 · V–1 ·s–1) of the ions produced by the neutralization source.
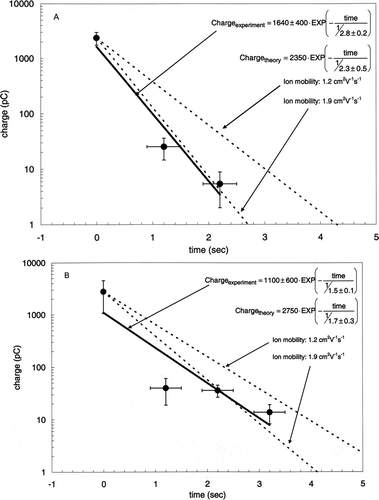
Validation of Results—Mass Measurements
The average charge on highly charged Teflon and TX40 filters was 4000 and 400 pC, respectively (refer to ). The average charge remaining on these filters after 5 s of neutralization with two 80 μCi sources is predicted by the experimental time constants to be 2 and 0.2 pC, given the relationships
plots the average in the mass-average mass for each weigh number (Method 1). Analysis of variance indicates that at a confidence level of α= 0.1, there is no difference between the 6 data points. Additionally, a neutralization decay trend is not evident and we conclude that 5 s of neutralization with two 80 μCi 210Po sources is sufficient to neutralize highly charged TX40 filters. The data also shows that, on average, the filter mass after 5 s of neutralization is <1 μg different from mass measured after 50 s of neutralization. depicts the results of method 2, a measure of the average difference between the “true mass” and the mass measured after 5 s neutralization for both neutralization treatments. The results show that the mass difference is less than 0.5 μg for both treatments and is not statistically significantly (α= 0.05) different from 0. Both methods of data analysis show how a microbalance could itself be used as a static charge detector.
DISCUSSION
This work was motivated by recent mass measurement regulations that require “charge” measurement so it will be useful to put our results in context with this requirement. We showed that the length of neutralization necessary can be predicted when the charge and strength of the neutralizing source are known. A worst-case neutralization scenario can be imagined as a static charge of 10,000 pC and a single pair of 80 μCi 210Po sources. The more conservative results of the low-mobility theoretical model and a charge-to-mass relationship of 20 pC = 1 μg is assumed. If a static charge influence of <0.1 μg is desired, given the above scenario, the results of this work will allow the establishment of a standard neutralization method. The method would require “filters to be neutralized on each side for at least 6 s using an alpha particle source that has an activity of no less than 80 μCi.” This recommendation is consistent with our experimental results, but there are still uncertainties that may prevent a purely time-based method from being adaptable to regulation. For example, the initial activity of commonly used 500 μCi sources ranges from 400 to 500 μCi (CitationSwanson 2008). In this work, the observed static charge decay occurred slightly faster than predicted by theory, suggesting the initial activity of our particular sources was near the upper end of this range. Additionally, we have rather optimistically assumed that best-practices are always followed and that the handling procedures we use are similar to others' with respect to the maximum charge levels generated. To ensure negligible charge exists on every filter, a charge limit (e.g., 20 pC) would be specified and measurements made with the CMD would be used to demonstrate compliance.
Weighing filters inside a Faraday cage may eliminate the influence of static charge. In brief, the filter is weighed inside a metal cage so that the charges on the filter are attracted to the cage but isolated from the surroundings. The charge is not eliminated but it can no longer influence the balance reading. This method has been investigated (CitationKoistinen et al. 1999) and found to increase the variability of filter weights. The authors hypothesized that increased mechanical instability due to the weight of the Faraday cage limited the precision. The weighing pan of an ultra-microbalance is very light and constructing a Faraday cage of equal mass to replace the pan poses an engineering challenge. CitationLawless and Rodes (1999) performed experiments with Teflon filters, a Faraday cage, and an extended balance pan. The extended pan works like the Faraday cage, shielding the bottom of the balance from charge on the outside edge of the filter. The authors obtained similar precisions with the extended pan (1.4 μg) and with an extended pan and Faraday cage (1.7 μg). CitationBrown et al. (2006) weighed charged filters on aluminum foil pans (similar to extended pan idea) and found no significant static charge influence. Weighing filters inside a Faraday cage or with modified balance pans are reasonable ideas but neutralization with radioactive sources is proven to work and is currently more practical. If further restrictions are placed on the handling and usage of radioactive materials, modifying the balance is such ways may become an attractive option.
Issues surrounding charged filters have been present for many years and will likely continue. In one sense, this is unavoidable; ionizing cosmic rays, ion diffusion to the filter's surface, and the exponential-like decay of charge during neutralization all suggest that the amount of charge on a filter will never be zero. Likewise, static charge can never be “completely removed” from a filter. CitationFeeney et al. (1984) suggested that Teflon filters may even charge themselves; that is, when the material mechanically relaxes after handling or movement, polarization charge forms on the surface. This may be an important observation. Even if static charge is significantly reduced by a neutralization source, the act of placing the filter on the balance may “create” charge. The net charge is not changed but in a non-symmetric weighing chamber, the amount and location of static charge influences the result.
CONCLUSIONS
A device was constructed that measures the static charge on filters used for gravimetric analysis. The static charge present on charged PM filters varied from 25–6,000 pC, depending on the procedure and type of filter. Friction charging due to typical handling procedures is believed to be the main contributor of static charge for both filter medias, Teflon membrane with Teflon support ring (Teflon) and Teflon-coated glass fiber (TX40). A neutralization time constant (the time it takes for the charge on the filter to decay by ∼63%) for Teflon filters was measured using 430 and 235 μCi 210Po sources. The time constants were 0.4 and 0.7 s, illustrating that neutralization occurs very quickly. The characteristic decay times matched those predicated by theory within experimental and theoretical error. To evaluate if 5 s of neutralization is sufficient for two filter medias, typical charge levels and a specific balance geometry, gravimetric analysis was performed on TX40 and Teflon filters. The results showed that 5 s of neutralization reduced the static charge influence on highly charged filters to <1 μg. The results allowed us to propose a standard method for static neutralization. The method requires that filters are neutralized on each side for at least 6 s using an alpha particle source that has an activity of no less than 80 μCi. To minimize uncertainty, we recommend that the absence of static charge on a filter always be confirmed with a charge measuring device or with the data analysis techniques we proposed.
Acknowledgments
This work was funded by Donaldson Company, Inc. We acknowledge the technical and editorial advice provided by Winthrop Watts (UMN), David Dikken (Measurement Technology Laboratories), and Richard Chase (formally of Ford Motor Company). Wanshu He (UMN) assisted in the laboratory. We thank the anonymous reviewers for their insight and helpful suggestions.
REFERENCES
- Allen , G. , Oh , J. A. , Koutrakis , P. and Sioutas , C. 1999 . Techniques for High-Quality Ambient Coarse Particle Mass Measurements . J. Air & Waste Manage. Assoc , 49 : PM133 – PM141 .
- Brown , A. S. , Yardley , R. E. , Quincey , P. G. and Butterfield , D. M. 2006 . Studies of the Effect of Humidity and Other Factors on Some Different Filter Materials Used for Gravimetric Measurements of Ambient Particulate Matter . Atmos Environ , 40 : 467 – 4678 .
- Chase , R. E. , Duszkiewicz , G. J. , Lewis , D. and Podsiadlik , H. 2005 . Reducing PM Measurement Variability by Controlling Static Charge . SAE Technical Paper Series , 2005-2001-0193
- Chase , R. E. and Schamp , D. 2007 . Measuring the Electrostatic Charge on a Filter . SAE Technical Paper Series , 2007-2001-0323
- Engelbrecht , D. R. , Cahill , T. A. and Feeney , P. J. 1980 . Electrostatic Effects on Gravimetric Analysis of membrane Filters . J. Air Pollut. Control Assoc , 60 : 391 – 392 .
- Federal Register . 2007 . “ Code of Federal Regulations, Title 40: Protection of Environment, Part 86: Control of Emissions from New and In-Use Highway Vehicles and Engines ” . Washington, DC : U.S. Government Printing Office .
- Feeney , P. J. , Cahill , T. A. , Olivera , K. and Guidara , R. 1984 . Gravimetric Determination of Mass on Lightly Loaded Membrane Waste Filters . J. Air Pollut. Control Assoc , 31 : 376 – 378 .
- Fujikoka , N. , Tsunoda , Y. , Sugimura , A. and Arai , K. 1983 . Influence of Humidity on Variation of Ion Mobility with Lifetime in Atmospheric Air . IEEE Trans. Power Syst , PAS-102 : 911 – 917 .
- Halliday , D. , Resnick , R. and Walker , J. 2001 . Fundamentals of Physics , New York : John Wiley & Sons, Inc. .
- Jesse , W. P. and Sadauskis , J. 1953 . Alpha-Particle Ionization in Pure Gases and the Average Energy to Make an Ion Pair . Physical Review , 90 : 1120 – 1121 .
- Khalek , I. A. 2006 . “ 2007 Diesel Particulate Measurement Research ” . In CRC Project E-66, Phase-2 , Southwest Research Institute .
- Keithley Instruments, Inc. 2004 . Low Level Measurements Handbook, , 6th , 4 – 38 . 4 – 39 . Keithley Instruments, Inc. .
- Kittelson , D. B. , Moon , K. C. and Collings , N. 1986 . “ Electrical Charge on Diesel Particles ” . In 2nd Int. Aerosol Conf., Berlin
- Knudsen , E. and Israelsson , S. 1975 . Mobilities of Small Ions in the Atmospheric Surface Layer . Pure Appl. Geophys , 113 : 525 – 533 .
- Koistinen , K. J. , Kousa , A. , Tenhola , V. , Hänninen , O. , Jantunen , M. J. , Oglesby , L. , Künzli , N. and Georgoulis , L. 1999 . Fine Particle (PM 2.5) Measurement Methodology, Quality Assurance Procedures, and Pilot Results of EXPOLIS Study . J. Air & Waste Manage. Assoc , 49 : 1212 – 1220 .
- Lawless , P. A. and Rhodes , C. E. 1999 . Maximizing Data Quality in the Gravimetric Analysis of Personal Exposure Sample Filters . J. Air & Waste Manage. Assoc , 49 : 1039 – 1049 .
- Lui , B. Y. H. and Pui , D. Y. H. 1974 . Electrical Neutralization of Aerosols . J. Aero. Sci , 5 : 465 – 472 .
- Maricq , M. M. 2006 . On the Electrical Charge of Motor Vehicle Exhaust Particles . J. Aerosol Sci , 37 : 858 – 874 .
- Murtomaa , M. , Pekkala , P. , Kalliohaka , T. and Paasi , J. 2005 . A Device for Aerosol Charge Measurement and Sampling . J. of Electrostatics , 63 : 571 – 575 .
- NRD LLC . Staticmaster Microbalance Ionizers and Brushes. Product Literature. www.nrdinc.com
- Takebe , M. 1974 . Positive Ion Species and Their Mobilities in Air . Japanese J. Appl. Physics , 13 : 207 – 217 .
- Swanson , J. J. 2008 . Personal communication with Tom Caffarella, NRD LLC .
- Ungethüm , E. 1974 . The Mobilities of Small Ions in the Atmosphere and Their Relationship . J. Aerosol Sci , 5 : 25 – 37 .
- Vaughan , N. P. , Milligan , B. D. and Ogden , T. L. 1989 . Filter Weighing Reproducibility and the Gravimetric Detection Limit . Ann. Occup. Hyg , 33 : 311 – 337 .
- Vonegut , B. 1973 . “ Atmospheric Electrostatics ” . In Electrostatics and Its Applications , Edited by: Moore , A. D. 393 New York : John Wiley & Sons, Inc. .
- Weil , J. 1990 . “ Static Control for Balances. Cahn Technical Note. Accessed through 40 CFR 50, Appendix L ” .