Abstract
An atmospheric measurement campaign took place in the spring of 2006 to characterize the emission of particles from an integrated iron and steelmaking site. During the measurement campaign, the PM 10 daily limit value of 50 μ g m − 3 was not exceeded during any day. However, excursions in PM 10 concentrations occurred over periods of a few hours which were associated with wind passing over the steelworks' site. Measurements with an Aerosol Time-of-Flight Mass Spectrometer (ATOFMS) showed six particle classes associated with emissions from steelmaking processes. Two of these were iron-rich, one showing internal mixing with nitrate, the other internally mixed with phosphate, which subsequent analysis identified as arising from the ironmaking sector and the hot and cold mills as the dominant sources, respectively. Other ATOFMS classes were rich in lead, zinc, and nickel, which were also associated with steelmaking sources. A Micro Orifice Uniform Deposit Impactor (MOUDI), used to measure particle size distributions over periods of 19–42 hours, showed two characteristic size distributions for iron, one bimodal with modes at 0.45 μ m and 4 μ m, the other unimodal centered at 6 μ m. In the former case, the smaller mode exhibited a peak for lead at the same particle size and in the case of the larger mode, phosphate and calcium also showed a peak at 4 μ m diameter, consistent with the ATOFMS findings. An additional particle type with a unimodal size distribution centered at about 1.2 μ m, with internally mixed Pb, Zn, and Cl but not Fe was also found.
1. INTRODUCTION
Recent toxicological studies have suggested that particle number, size and composition may all play important roles in adverse health impacts (CitationDonaldson et al. 1997). In particular, epidemiological and toxicological investigations have sought to establish the chemical components primarily responsible for particle toxicity (CitationHarrison and Yin 2000). Such studies have frequently implicated the metal content (particularly water-soluble metal) as a possible harmful component of particulate matter (PM), which triggers the generation of reactive oxygen species (ROS), switching on cellular pro-inflammatory response pathways (CitationCosta and Dreher 1997; CitationDonaldson et al. 1997; CitationAdamson et al. 2000).
The sources of particle-bound metals are vast, with metals such as aluminum, silicon, iron, and calcium arising from crustal materials, whilst heavy metals such as lead, cadmium, mercury, nickel, chromium, zinc, and manganese can be emitted from anthropogenic sources such as metallurgical processes and road transport (CitationAQEG 2005, CitationLough et al. 2005; CitationBirmili et al. 2006). The impact of steel plants on chemical composition of the PM has been investigated in a number of studies. CitationPope (1996) reported that, during the closure of a steel mill in the Utah valley, a reduction in PM10 mass and changes in its composition were associated with decreases in morbidity and mortality of the local population. In a similar way, CitationHutchison et al. (2005) reported an increase in particle metal content when samples were taken downwind of a steelworks, which in turn were associated with an increased inflammation of a rat lung.
A few studies have endeavored to determine tracers for identifying impacts from steelworks, for example CitationOravisjarvi et al. (2003) used receptor modeling to determine the steelworks contribution to ambient PM2.5 concentrations, and found that K, Cl, Cd, and Pb were good tracers for emissions from sintering whereas Mn, F, Zn, and Fe were representative of smelting processes. However, there is still limited data characterizing the emission of fine and ultrafine particulate matter by iron and steelmaking processes.
The integrated iron and steelmaking process is the main production route used in Europe and worldwide and is so-called because it involves a number of linked processes whereby iron is extracted from its ores in the blast furnace (BF) and the resulting molten iron is subsequently refined into liquid steel by the basic oxygen steelmaking (BOS) process. The integrated process also includes iron ore sintering and cokemaking facilities which are used to prepare agglomerated iron ores and coke, respectively, for use in the blast furnace burden. Owing to the non-continuous nature of many steelmaking processes, traditional off-line particle sampling techniques such as filter-based techniques do not have sufficient time resolution (typically 24 hours) to capture short-lived emission events arising from specific operations. Moreover, traditional filter-based techniques suffer from sampling artifacts and provide very limited information on the mixing state of the particles. In this article we combine a time-averaged sampling technique (cascade impaction) with Aerosol Time-of-Flight Mass Spectrometry (ATOFMS) which measures the aerodynamic size and chemical composition of individual particles between 0.1 and 3 μ m in real time (CitationSuess and Prather 1999; CitationGard et al. 1997). These measurements can provide great insights into the association between chemical species within individual particles. The aim is to elucidate the link between composition of particles from specific sources and atmospheric composition. Both aerodynamic diameter size distributions and chemical mixing states of specific types of airborne particles will be discussed.
2. EXPERIMENTAL
2.1. Sampling Site and Steelworks Sources
Sampling took place in the coastal town of Port Talbot next to one of the largest integrated steelworks in the UK. The sampling site chosen forms part of the UK national monitoring station which is located between the steelworks, to the south-west of the site, and a major motorway network to the east/north-east of the site. The proximity of the site to residential receptors can be seen in , along with the major steelworks processes. Emissions from integrated steelmaking facilities are a complex mixture of stationary source and fugitive emissions associated with the main processes, and fugitive emissions from general site operations such as the stocking, blending, and transportation of particulate raw materials on site. The situation is further complicated by the fact that some processes operate continuously while others are batch processes, some of which may be quasi-continuous. Some of the main emission sources, their characteristics and components of the emissions are summarized in .
TABLE 1 Brief summary of emission sources and types, and main components
2.2. Measurement Techniques
2.2.1. Aerosol Time-of-Flight Mass Spectrometry
The ATOFMS was housed in a mobile laboratory parked within 10 m of the national air quality monitoring station and sampled continuously from 19:00 24 April 2006 until 13:00 5 May 2006. The ATOFMS (TSI Model 3800–100) collects bipolar mass spectra of individual aerosol particles. Ambient aerosol is focused by an aerodynamic lens into a narrow particle beam for sizes between 100 nm and 3 μ m (CitationSu et al. 2004). This is a major improvement towards smaller particle sizes compared to the previous model of the ATOFMS (3800) that utilized a nozzle/skimmer combination for the inlet (CitationGard et al. 1997). Using a 2-laser velocimeter, particle sizes are determined from particle velocity after acceleration into the vacuum. In addition the light scattered by the particles is used to trigger a pulsed high power desorption and ionization laser (λ 266 nm, ∼ 1 mJ/pulse) which evaporates and ionizes the particle in the centre of the ion source of a bipolar reflectron ToF-MS. Thus from an individual particle that is detected in the velocimeter positive-ion and negative-ion spectra are obtained, reflecting qualitatively the chemical composition of the particle. Deriving quantitative information from the ATOFMS is not yet straightforward, showing a strong size dependence, mainly due to the inlet design (CitationAllen et al. 2000b; CitationDall'Osto et al. 2006; CitationQin et al. 2006). During this study a Scanning Mobility Particle Sizer (SMPS TSI 3071A) and an Aerodynamic Particle Sizer (APS TSI 3321) were deployed and ATOFMS counts were scaled following procedures described elsewhere (CitationDall'Osto et al. 2006; CitationQin et al. 2006). The ATOFMS datasets are imported into YAADA (www.YAADA.org) where single particle mass spectra can be grouped with Adaptive Resonance Theory neural network ART-2a (CitationSong et al. 1999; learning rate 0.05, vigilance factor 0.85 20 iterations). The resulting area matrices (AM) for the particle clusters represent the average intensities for each mass-to-charge (m/z) for all particles within the group and therefore reflect the average of the mass spectrum of the particles in the cluster.
2.2.2. Micro-Orifice Uniform Deposit Impactor (MOUDI)
A type 100 MOUDI (MSP Corporation) was co-located with the ATOFMS with sampling times ranging from 19 to 42 hours. The MOUDI collects particles across-eight stages with 50% cut-points for the particle aerodynamic diameter (μ m) as follows: 18, 9.9, 6.2, 3.1, 1.8, 1.0, 0.57, 0.356, and 0.196 (CitationMarple et al. 1991). The pump used to supply the sampling flow rate 30 l min− 1) was a Gast twin piston pump. The 50% cut-off parameters were adjusted according to the actual flow rates employed and the data have been inverted and plotted according to the methods outlined by CitationAllen et al. (2001). The particles fractionated at each MOUDI stage were collected on Teflon filters which were previously treated with silicone grease to avoid particle bounce. The size-segregated particle ensembles of each impaction substrate were analyzed by procedures described elsewhere (CitationHuang et al. 2004; CitationAllen et al. 2000a). Ion chromatographic analysis was performed for Na+, K+, Ca2 +, Mg2 +, Cl−, PO4 3 −, SO4 2 −, and NO3 −. Furthermore, the MOUDI substrates were analyzed for 23 elements (Be, Na, Mg, Al, K, Ca, V, Cr, Mn, Fe, Co, Ni, Cu, Zn, As, Se, Sr, Ag, Cd, Cs, Ba, Tl, Pb) by ICP/MS.
2.2.3. Meteorological and PM 10 Data
Meteorological parameters (temperature, humidity, solar radiation, wind speed, and direction) were monitored at the site using a Davis Vantage Pro meteorological station that provided 1-minute resolution data. PM10 measurements were obtained from a tapered element oscillating microbalance monitor (TEOM, Rupprecht & Pataschnick, Inc.) operated by the national monitoring network based on fifteen minute averages. Mean figures for 1 hour were calculated for each parameter.
3. RESULTS AND DISCUSSION
3.1. Overview
The weather during the field study was characterized by warm conditions (mean hourly temperature 11.5 ± 3.1°), relative humidity (72.8 ± 12.7%) and low rainfall (only two small events within two weeks). Furthermore, wind speed was generally low (average during the field study 1.3 ± 1.0 m/s), with very few occasions of strong wind (> 6 m/s). The local wind pattern was characterized on some days by a typical sea-breeze pattern with wind from the land to the sea during the night and in the opposite direction during the afternoon. shows wind speed and direction for the whole study period. The total PM10 data from the UK monitoring site at Port Talbot (expressed as TEOM output × 1.3) is depicted in . This adjustment factor (1.3) is applied in the United Kingdom to compensate for the loss of semi-volatile constituents in the heated (50°C) inlet of the TEOM (CitationAPEG 1999). Throughout the entire campaign the average of hourly PM10 was 33 ± 22 μ g m− 3 (s.d.), while the average daily concentration was 33 ± 8 μ g m− 3. The 24 h limit value for PM10 of 50 μ g m− 3 was not exceeded during any day of the field study. However, shows that some PM10 episodes of elevated concentrations occurred with the highest concentration of PM10 (about 140 μ g m− 3) recorded between 15:00–18:00 on 5 January 2006.
FIG. 2 (a) Wind direction (WD, Degrees) and wind speed (WS, m s− 1) for the field study at Port Talbot, Spring 2006; (b) PM10 hourly concentrations; and (c) ATOFMS temporal trends (1 hour resolution) of main ATOFMS classes expressed as sum of the main 3 groups of particles shown in .
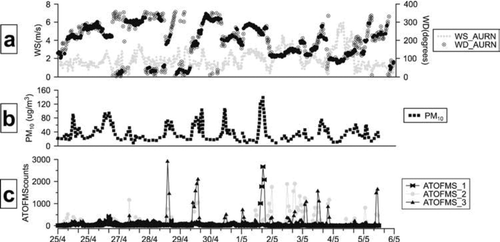
Due to both a change in synoptic winds during the sampling period as well as mesoscale effects such as the land-sea breeze circulation none of the MOUDI samples corresponded to constant wind directions, therefore all will contain some contribution from both the steelworks and motorway sources as well as from the marine and terrestrial backgrounds. However, the high temporal resolution of the ATOFMS (1 h time resolution shown in ) did allow specific periods with a steelworks influence to be observed. In the following section ATOFMS data will be presented, showing specific particle types associated with discrete steelworks wind direction sectors. In a second step, the short but intense episodes of ATOFMS unique particle types linked with the steelworks will be discussed along with the PM10 hourly concentrations. Finally, size-segregated MOUDI mass loading samples will show complete size distributions of different inorganic species, including a description of the chemical composition of the particulate matter and the steelworks processes likely to generate it.
3.2. ATOFMS Particle Types
The ATOFMS sized 4,679,911 particles of which 534,567 particles were ionized. The ART-2a algorithm used 507,262 particles in generating 507 particle clusters representing 94.89% of the total number of successfully ionized particles. By merging similar clusters (CitationDall'Osto and Harrison 2006), the total number of clusters describing the whole database was reduced to 30. By coupling the ATOFMS real-time data with meteorological data, 9 different particle types were identified which could be associated with wind directions from the steelworks and all show rapid excursions, compared with baseline particle counts, on a number of occasions throughout the measurement campaign indicating influences from local sources. These 9 classes represent 31.6% of the total number of particles ionized by the ATOFMS. As shown in non-metallic types constituted 27.1% of the particles, whilst metal-rich types comprised 4.5% of the total. The remaining 68.4% of the ionized particles were found to be attributable to particle types commonly observed in ambient air studies including sea-salt, soil dust, biomass burning, and lubricating oil, all of which could be associated with other non-steelworks sources. A detailed discussion of the non-metallic particle types is presented in CitationDall'Osto et al. (2008). Briefly, three non-metallic particle main classes were found. The ATOFMS detected for the first time a unique elemental sulphur-rich particle type, likely to originate from the blast furnaces. AMS results, supported also by laboratory studies, confirm this finding by reporting elevated mass ratios m/z 64/48 and m/z 64/80. Two other types of particulate matter were found to be associated with the steelworks. One was characterized by nitrogen-containing organic species, aromatic compounds and low molecular weight (MW) polycyclic aromatic hydrocarbons (PAH) and was associated with the hot and cold mills emissions. The second was found to be rich in organic carbon internally mixed with elemental carbon, nitrate, sulphate, and PAH with higher MW.
TABLE 2 Summary of the main ATOFMS classes obtained using ART-2a, detected during spring 2006, Port Talbot
The following discussion will focus on the metallic particle types that were found to be unique to the study in Port Talbot and could be related to particular steelworks process sectors.
3.3. Temporal Profile of Steelwork Particle Clusters
As shown in the major steelworks processes are located in very close proximity to each other making it difficult to distinguish between a number of the processes. However, by examining the temporal trend of each steelworks-related particle type, three main groups of ATOFMS particle types within the steelworks were identified. Each group was characterized by specific wind sectors and related to potential steelworks source groups within those sectors including: (1) sinter plant/blast furnaces/iron-ore stockpiles (ironmaking, 230–270 o ); (2) steelmaking, cokemaking, and coal stockyards (steel/cokemaking 190–230 o ); and (3) hot and cold rolling mills (mills, 160–190 o ). The temporal trends of the sum of ATOFMS particle types comprising each of the 3 groups reported in are shown in together with wind speed, wind direction and PM10 concentration. The results in show that the sharp increases observed in the temporal trend of the ATOFMS classes can be linked with excursions in PM10 concentration typically lasting a few hours. It is also observed that the majority of periods with PM10concentrations above 50 μ g m− 3 correspond to wind directions from the steelworks (170–300°). However, it should be noted that these excursions did not lead to an exceedance in the 24-hour PM10 limit value at this site. To investigate further the sources of these higher PM10 concentrations the internal and external chemical mixing of the particle types listed in need to probed.
TABLE 3 Summary of Port Talbot steel industry sources linked with wind sectors
The strongest associations between a given source sector and particle types were observed for iron-containing ATOFMS classes, denoted FeP and Fe-rich. The mass spectra of the Fe-rich particle type, shown in , indicate internal mixing with sodium and potassium at m/z 23 and 39 in the positive spectrum, respectively. Strong peaks at m/z –46 and −62 due to nitrate can also be seen in the negative-ion mass spectrum with smaller peaks at m/z −35, −79, and −97 confirming the presence of chloride, phosphate, and sulphate, respectively. The absence of a signal at m/z 27 [Al]+ is a key feature for this particle type, which makes this class different from soil/rock dust previously reported in other ATOFMS studies (CitationSilva et al. 2000; CitationLiu et al. 2003; CitationDall'Osto and Harrison 2006). A number of features of the mass spectra of the Fe-rich particle type are similar to iron-rich dust particles previously detected in other urban field studies. However, occurrences of peaks in time series of the Fe-rich particle type suggest that the largest single impact of this particle type originated primarily from the ironmaking sector as observed on 1 May (2 pm–8 pm) when it was in concurrence with one of the non-metallic ATOFMS classes, the sulphur-rich type (CitationDall'Osto et al. 2008). Both particle-types are likely to be due to emissions from the BF as a result of furnace tapping and slag quenching operations. In general, emissions of iron-rich particles from the ironmaking sector are not surprising and can result from sinter plant and blast furnace processes and also from stocking and blending of raw materials—(). In addition, sinter plant PM emissions are known to contain high concentrations of potassium, and to a lesser extent sodium and lead (CitationSchofield et al. 2007). The extent to which these species are internally mixed within the emissions is not well characterized but it is likely that both lead and potassium will be found in finer particles compared to sodium and iron. Two studies by Moreno et al. (Citation2004a,Citationb) have been reported where sampling took place at the same sampling site in Port Talbot. Results showed that both fine and coarse samples, collected predominantly downwind of the steelworks, contained Fe-rich spherules complementing observations made in this study. The morphologies of particles from different sources within the ironmaking sector could shed further light on the sources from which they originate. Thus, for example, Fe-rich particles from tapping of the BF are produced by oxidation of molten iron and will almost certainly be spherical, whereas those from iron ore sintering and raw materials stockyards originate from the iron ores used on site and are expected to be more angular.
FIG. 3 Positive (+) and negative (−) ART-2a area vectors attributed to (a) Fe-rich, (b) FeP, (c) Pb, (d) Zn, (e) KCl, (f) Ni.
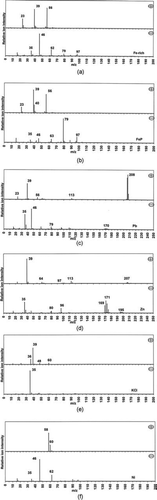
A second iron-containing particle class was observed during this study, the FeP class, for which the mass spectra are shown in . The positive-ion spectrum indicates the presence of [Na]+ (m/z 23), [K]+ (m/z 39) and [Fe]+ (m/z 56), whereas the absence of satellite peaks from calcium-containing species (i.e., m/z 57 [CaO]+ or m/z 96 [Ca2O]+ suggests that the peak at m/z 40 is due to broadening of the potassium peak at m/z 39. In the negative-ion spectrum, strong internal mixing with phosphate is observed (m/z -63 [PO2]− and m/z -79 [PO3]−) with smaller peaks attributed to [Cl]− (m/z 35 and 37), [NO2]− (m/z -46) and [NO3]− (m/z −62). Several earlier studies have attributed iron-containing particles to the steel industry (CitationInjuk et al. 1998; CitationMachemer 2004; CitationAhn and Lee 2006). In particular, CitationMachemer (2004) reported iron as the predominant element in PM samples taken directly from BF and BOS sources. XRD studies demonstrated that in both cases iron was found either as hematite, magnetite, maghemite or iron oxide, but there was no evidence cited of iron being present in combination with phosphorus from either BF or BOS sources. In this study it was found that the major peaks in the FeP class were related to periods when the wind was from a southerly direction in which the only major steelworks sources are the hot and cold mills. From a consideration of the process of rolling steel the most plausible explanation for the occurrence of FeP particles is that the phosphate is derived from materials used in the rolling mills, with water treatment chemicals or hydraulic fluids being the most likely sources. Further investigation revealed that corrosion inhibitors added to water used in the hot mill contain phosphates among other ingredients. The water is used in high-pressure jets for de-scaling steel slabs prior to rolling and also for cooling the rolls during the rolling process. In both instances the water comes into contact with the hot steel surface and evaporation of water may lead to the formation of the FeP particle type. More iron oxide fume is produced in the rolling process as the steel surface area exposed to the atmosphere increases and hence it is considered more likely that the Fe-P particles are formed to a greater extent, for example, in the rolling process than in de-scaling.
In comparison, the temporal trends for Pb and Zn particle types showed that these ATOFMS classes originated from all major steelwork source sectors. However, the strongest peaks for both metal classes were observed from the mills sector. It should be noted that the positive-ion and negative-ion mass spectra for Pb and Zn classes show that there is internal mixing between each of these metals to some extent, for example the Zn class () shows presence of zinc by m/z at 64 [Zn]+, 97 [ZnCl]+ and –169 [ZnCl2]− as well as lead (m/z 208) and sulphate (m/z -80 and -96). There are also strong signals due to potassium (m/z 39) and chloride (m/z –35) in the positive-ion and negative-ion spectra, respectively. Moreover, the presence of [K2Cl]+ (m/z 113) supports the mixing state of these two elements. The nature of particulate emissions from hot and cold rolling processes are not well characterized with the only detailed study (CitationTsai et al. 2007) reporting that Na, Fe, K, Ni, and Ca are the major metals present in the stack emissions, where the reheating furnaces used coke oven gas as the fuel. It should be noted that at Port Talbot natural gas is used as the fuel for reheating furnaces which is likely to lead to different emission characteristics from hot and cold rolling mill stacks to those observed by (CitationTsai et al. 2007). Fugitive emissions also result from hot and cold rolling processes and emissions from cooling towers, both natural-and forced-draught systems, which may result in diffuse emissions of metals that are dissolved in the water. For example, zinc and molybdenum are known additives in certain water treatment chemicals used in the rolling mills, while sodium, potassium, iron, and calcium will be present in water used on site. However, the occurrence of lead-containing particulate from the mills area is difficult to explain, since there is no leaded steel production at Port Talbot, and insofar as is known no lead compounds are used in the water treatment chemicals used in the hot and cold mills. There are many water-cooling systems on an integrated steelworks all of which require the use of specialized water treatment chemicals to prevent corrosion, legionella and mould growth. Evaporation of water in cooling towers will give rise to multiple sources of diffuse emissions of fine particles of dissolved salts present in the water either naturally or from the water treatment chemicals. The cooling water circuits operate continuously and are distributed across the site so that diffuse emissions from cooling towers will contribute to the background concentrations of fine particles and metals such as zinc, sodium, potassium, and calcium. Zinc and lead are known to be significant components of sinter plant and BOS plant particle emissions. The sinter plant in particular is known to be a major source of Pb, K, and Cl that are found predominantly in fine and ultrafine particle fractions (CitationSchofield et al. 2007; CitationTsai et al. 2007). Past studies of BOS plant particle emissions indicate the presence of both Zn and Pb in the fine particle fraction (CitationSchofield et al. 2007) with the possibility of some internal mixing.
The ATOFMS KCl particle type had a very different temporal trend compared with the other metals, such that the baseline particle count was higher and there were fewer notable excursions. The KCl cluster () exhibited peaks at m/z 39 [K]+ and m/z -35 [Cl]− and similar particles have previously been attributed to biomass burning (CitationSilva et al. 1999; CitationGuazzotti et al. 2003; CitationHudson et al. 2004; CitationDall'Osto and Harrison 2006). Previous laboratory studies (CitationSchofield 2004) showed that this type of particle also exhibits similar features to the mass spectra of particles apportioned to vegetative debris. However, during the present study the presence of elemental carbon (m/z 36, 48, and 60) and the absence of m/z -26 [CN]− and m/z –42 [CNO]− suggests a different source. There are two major steelwork sources that together are likely to account for the observed temporal trend. In particular, K and Cl are known to be major components in sinter plant emissions (CitationSchofield et al. 2007; CitationOravisjarvi et al. 2003) which as discussed earlier is a continuous emission source that could give rise to the elevated baseline particle count. It is also possible that peaks in KCl are associated with particles formed via coke quenching processes that occur in the steel/cokemaking sector.
Finally, unique positive-ion and negative-ion mass spectra of the particle type named Ni are shown in . The positive spectrum is dominated by the presence of m/z 58 and 60 due to nickel. The negative spectrum is dominated by the nitrate peaks (m/z –46 and –62) with a small chloride signature (m/z –35). This class showed some unique temporal trends. On one occasion (29/04/06 12:00–15:00) the temporal trends showed a clear correlation with the other mills classes (Zn-rich, Pb-rich, and KCl-rich). However, two spikes (26/04 22:00–23:00 and 27/04 13:00–17:00) were also detected when the wind direction was about 280–300°. The origin of this particle type may be the nearby docks, power and cement plants.
The sensitivity of the ATOFMS shows a strong size dependence, mainly due to the inlet design (Allen et al. 2000; CitationDall'Osto et al. 2006). The ATOFMS used in this study was equipped with an aerodynamic lens, which greatly improved the transmission efficiency (CitationSu et al. 2004). However, the efficiency still shows a strong size dependence, with its maximum being at around 0.7 μ m. During this study an SMPS and an APS were deployed and ATOFMS counts were scaled following procedures described elsewhere (CitationDall'Osto et al. 2006; CitationQin et al. 2006). While the class Pb-rich shows a maximum at the smallest ATOFMS detectable size (0.3 μ m), class Zn-rich presents a unimodal distribution peaking at 400 nm. The other steelworks inorganic particle types presented unimodal size distributions peaking at 300 nm (Fe-rich), 500 nm (KCl rich), and 600 nm (Ni-rich).
3.4. Comparison of Metallic ATOFMS Steelwork Classes With MOUDI Size Distributions
3.4.1. Trace Metal Size Distributions
Nine MOUDI samples were collected during the measurement campaign. MOUDI samples showing the highest iron concentrations occurred on 25–26 April, 29 April, 1 May, and 2 May. On these days, zinc and phosphate concentrations were particularly elevated on 29 April and slightly elevated on 2 May, while Pb exhibited elevations (with different size distributions) on 25–26 April and 29 April. On the basis of wind directions, ATOFMS particle type temporal trends and PM10 concentrations, it is thought that MOUDI samples from the 25–26 April and 1 May were strongly influenced by the ironmaking wind sector (see ) while those on 29 April and 2 May were influenced by the mills in combination with steel/cokemaking and mills only sectors, respectively. The remaining five samples were less obviously affected by the steelworks with iron concentrations in the range 0.3–0.9 μ g m− 3, compared with the industry-influenced samples that had iron concentrations ranging from 1.3–2.3 μ g m− 3.
The size distributions of iron-containing particles fell into two groups: unimodal and bimodal (). Iron-containing particles apportioned to the ironmaking sector were characterized by a unimodal size distribution peaking at about 6 μ m, while those originating from the mills and steel/cokemaking sector presented a bimodal size distribution (0.45 μ m and 4 μ m). Manganese was also present in particle types apportioned to both the mills and steel/cokemaking, and the ironmaking sources, showing identical size distributions to iron. A number of past studies undertaken in the vicinity of a steel plant have identified Mn as a tracer for steelworks emissions (CitationMazzei et al. 2006; CitationPrati et al. 2000). For example, a study by Ledoux and co-workers (2006) showed that manganese was related to steel plant emissions and maintained the same chemical state during its atmospheric transport, suggesting that the relationship identified between Fe and Mn in this study could also represent the relationship at the emission source. With this in mind, the size distributions of Mn and Fe observed here could go some way towards identifying the potential sources in each of the sectors. The coarse unimodal distribution observed from the ironmaking sector indicates that wind-entrained dust from the iron ore stockpiles is likely to be the major source. Certain iron ores used at this site are known to be pre-mixed with Mn-rich ores before sintering in order to achieve the required iron/steel composition, therefore it is not surprising to observe the co-existence in Mn and Fe from the ironmaking wind sector. In comparison, the bimodal distribution of Mn and Fe observed when samples were influenced by the mills and steel/cokemaking sectors indicates the possibility of two major sources. A major source of Mn in this area is the BOS plant which has emissions that are known to be enriched in Fe and Mn (CitationSchofield et al. 2007) and are likely to result in fine to coarse mode particulates which are internally mixed with Ca, Mn, Mg, Si, and carbonate (CitationMachemer 2004). As reported by CitationTsai et al. (2007), the hot and cold rolling mills may constitute a second source of Mn and Fe in this area, but as stated earlier these emissions are not well characterized. It should be noted that the Fe-rich and FeP ATOFMS classes did not show signs of internal mixing with Mn, which may be due to a lower ATOFMS detection efficiency for Mn compared with other metals such as K, Fe, Pb, and Zn.
FIG. 4 MOUDI size distribution of iron for day 25/04/06 (Ironmaking emissions) and 02/05/06 (Mills and steel/cokemaking). Day 27/04/06 was chosen as a background iron concentration.
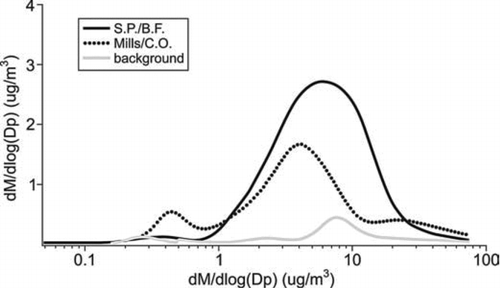
While iron-containing particles apportioned to the ironmaking sector were characterized by a unimodal size distribution peaking at about 6 μ m, internally mixed with Mn only, Fe-rich particles originating from mills and steel/cokemaking sectors were internally mixed with a number of other chemical species as shown in . This was confirmed by the unique ATOFMS FeP particle type detected primarily when airborne particles originated from wind sectors associated with mills and also the steel/cokemaking sectors (). The first mode at 0.45 μ m suggests that Fe and Pb are internally mixed in one particle type. This hypothesis is confirmed by the ATOFMS results, showing an independent Pb particle type internally mixed with iron (). The second mode at 1.2 μ m in shows the presence of Pb and Zn. It is important to note that these particle types are not internally mixed with iron, which corresponds directly with the Zn particle type detected with the ATOFMS (). The Zn size distributions observed in this study compare reasonably well with those reported by CitationVan Malderen et al (1996) where the size of Zn-rich particles varied between 0.3 and 1.0 μ m. As discussed earlier, fugitive BOS plant roof emissions are a possible source of Zn; however, zinc-rich particles are also likely to result from water treatment chemicals used in various water cooling circuits around the works. The third, coarser mode shown in at about 4 μ m is characterized by the presence of Fe and phosphate in rather similar abundances. These data are consistent with the ATOFMS FeP particle type, which was also found mainly in the coarser particles. Enrichment in PM10 phosphate mass concentration (average of 0.4 μ g m− 3) was found in the two mills-dominated samples compared with the other 7 samples (average of 0.07 μ g m− 3). As previously discussed, it is thought the presence of phosphate could be due to the water treatment chemicals or hydraulic oils used in the hot mills, where phosphate is added as an ingredient of corrosion inhibitors used in water cooling circuits in the hot mills.
FIG. 5 MOUDI size distributions for day 29/04/06 of zinc, lead, iron, and phosphate. Data are in dM/dlog (D p ) [μ g m− 3] for Fe and Phosphate, dM/dlog (D p ) [ng m− 3] for Zn and Pb. Sample was thought to be influenced by mills in combination with steel/cokemaking.
![FIG. 5 MOUDI size distributions for day 29/04/06 of zinc, lead, iron, and phosphate. Data are in dM/dlog (D p ) [μ g m− 3] for Fe and Phosphate, dM/dlog (D p ) [ng m− 3] for Zn and Pb. Sample was thought to be influenced by mills in combination with steel/cokemaking.](/cms/asset/c8bf62d7-5387-492a-8576-ac1fef4cb822/uast_a_334125_o_f0005g.gif)
The MOUDI data did not reveal any other major metals associated with the steel industry emissions. Unlike previous steelworks studies, specific sources of chromium were not found during this study and this metal was not found internally mixed with any of the dust particle types apportioned. Barium and copper were also not found internally mixed with any of the metal-rich particle types. These two elements were found in significant amounts only in one sample (03/05/07), presenting a mono-modal size distribution at 3 μ m when wind directions suggested a motorway origin, consistent with their use in brake pads (CitationBirmili et al. 2006).
3.4.2. Anion and Cation Size Distributions
As expected, ion chromatography results indicated a strong presence of sea salt aerosols, which is to be expected given the coastal location of the works. Unimodal size distributions of Na+, Ca2 +, Mg2 +, and Cl− peaking at about 3–5 μ m were observed in most of the samples, implying that most of the mass of these elements was due to the sea salt aerosols. However, samples typical of the mills source with some steel/cokemaking influence exhibited different size distributions for Ca2 + and Cl−; for Ca2 + a single mode was observed at about 5 μ m, while Cl− showed a predominant fine mode at about 2 μ m (see ). Although past studies have shown that the major constituents of coarse particles generated from a sintering plant are Fe2O3 and CaO (CitationAhn and Lee 2006), our study found no evidence for Ca in combination with Fe-rich particles associated with the ironmaking wind sector. The reason for this may simply be due to the difference in the particle size classes studied: CitationAhn and Lee (2006) characterized particles in the range 9.4–137 μ m, whereas the MOUDI samples in this study were < 10 μ m. In summary, the ATOFMS results support the interpretation of the MOUDI data. Firstly, no calcium was found in the fine metal-rich particle type and secondly, chloride presents an independent mode at about 2 μ m, confirming that other particle types (KCl, Zn, Pb) are specific particle types not internally mixed with iron.
CONCLUSIONS
During spring 2006, an Aerosol Time-of-Flight Mass Spectrometer was deployed next to a major integrated steelworks in Wales (UK), providing size information within the range 0.1 to 3 micrometers on individual particle in real time. A Micro-Orifice Uniform Deposit Impactor (MOUDI) was also deployed, showing the size-segregated mass loading of particle matter. Six metal-rich particle types associated with the steelworks were characterized by the ATOFMS which were named: Fe-rich, FeP, Pb, Ni, Zn, and KCl. The mass spectra indicated the Fe-rich and Fe-P types to be rich in iron, potassium, sodium, and nitrate, but varying in their phosphate content. The Pb-type was rich in lead, together with potassium, chloride, and nitrate. The Zn particle type contained also potassium and chloride, while the Ni type contained little other than nickel and nitrate. Using directional analysis, three main sources within the steelworks were identified: ironmaking, steel/cokemaking, and the rolling mills. Fe-rich particles from the first two sources presented a unimodal size distribution peaking at about 6 μ m, showing internal mixing with nitrate and manganese. The Fe-rich particles originating from the rolling mills presented a bimodal size distribution, peaking at 0.45 μ m and 4 μ m. The former mode was internally mixed with lead, the latter with phosphate and calcium. An additional particle type linked with the mills sector with a unimodal size distribution centered at about 1.2 μ m, with internally mixed Pb, Zn, and Cl but not with Fe was also found.
The ATOFMS and MOUDI techniques proved highly complementary despite their very different averaging times. Together, they provide a valuable insight into the composition and size distributions of emissions from the steel industry.
This work was undertaken with financial grants from the Research Fund for Coal and Steel of the European Community and the UK National Centre for Atmospheric Science (NCAS). The authors would also like to thank Mr Paul Collins for trace metal analysis of MOUDI substrates, Dr. Frank Drewnick for provision of meteorology data, and the UK AURN network for provision of gas and PM10 data.
REFERENCES
- Adamson , I. Y. R. , Prieditis , H. , Hedgecock , C. and Vincent , R. 2000 . Zinc is the Toxic Factor in the Lung Response to an Atmospheric Particulate Sample . Toxicol. Appl. Pharmacol , 166 ( 2 ) : 111 – 119 .
- Ahn , Y. C. and Lee , J. K. 2006 . Physical, Chemical, and Electrical Analysis of Aerosol Particles Generated from Industrial Plants . J. Aerosol Sci , 37 ( 2 ) : 187 – 202 .
- Allen , A. G. , Harrison , R. M. , James , J. D. , Davison , B. M. , Robertson , L. and Hewitt , C. N. 2000a . Impactor Aerosol Size Distributions at Two North Atlantic Coastal Sites . J. Aerosol Sci , 31 ( 1 ) : 372 – 373 .
- Allen , A. G. , Nemitz , E. , Shi , J. P. , Harrison , R. M. and Greenwood , J. C. 2001 . Size Distribution of Trace Metals in Atmospheric Aerosols in the United Kingdom . Atmos. Environ , 35 : 4581 – 4591 .
- Allen , J. O. , Fergenson , D. P. , Gard , E. E. , Hughes , L. S. , Morrical , B. D. , Kleeman , M. J. , Gross , D. S. , Galli , M. E. , Prather , K. A. and Cass , G. R. 2000b . Particle Detection Efficiencies of Aerosol Time of Flight Mass Spectrometers Under Ambient Sampling Conditions . Environ. Sci. Technol , 34 ( 1 ) : 211 – 217 .
- APEG . 1999 . “ Source Apportionment of Airborne Particulate Matter in the United Kingdom ” . Airborne Particles Expert Group, DETR, London.
- AQEG . 2005 . “ Particulate matter in the UK ” . Air Quality Expert Group, DEFRA, London.
- Birmili , W. , Allen , A. G. , Bary , F. and Harrison , R. M. 2006 . Trace Metal Concentrations and Water Solubility in Size-Fractionated Atmospheric Particles and Influence of Road Traffic . Environ. Sci. Technol , 40 ( 4 ) : 1144 – 1153 .
- Costa , D. L. and Dreher , K. L. 1997 . Bioavailable Transition Metals in Particulate Matter Mediate Cardiopulmonary Injury in Healthy and Compromised Animal Models . Environ. Health Perspect , 105 : 1053 – 1060 .
- Dall'Osto , M. and Harrison , R. M. 2006 . Chemical Characterisation of Single Airborne Particles in Athens (Greece) by ATOFMS . Atmos. Environ , 40 ( 39 ) : 7614 – 7631 .
- Dall'Osto , M . 2008 . Real Time Measurements of Non Metallic Fine Particulate Matter Next to a Steel Works, In preparation.
- Dall'Osto , M. , Harrison , R. M. , Beddows , D. C. S. , Freney , E. J. , Heal , M. R. and Donovan , R. J. 2006 . Single-Particle Detection Efficiencies of Aerosol Time-of-Flight Mass Spectrometry During the North Atlantic Marine Boundary Layer Experiment . Environ. Sci. Technol , 40 ( 16 ) : 5029 – 5035 .
- Donaldson , K. , Brown , D. M. , Mitchell , C. , Dineva , M. , Beswick , P. H. , Gilmour , P. and MacNee , W. 1997 . Free Radical Activity of PM10: Iron-Mediated Generation of Hydroxyl Radicals . Environ. Health Perspect. , 105 : 1285 – 1289 .
- Gard , E. , Mayer , J. E. , Morrical , B. D. , Dienes , T. , Fergenson , D. P. and Prather , K. A. 1997 . Real-Time Analysis of Individual Atmospheric Aerosol Particles: Design and Performance of a Portable ATOFMS . Anal. Chem , 69 ( 20 ) : 4083 – 4091 .
- Guazzotti , S. A. , Suess , D. T. , Coffee , K. R. , Quinn , P. K. , Bates , T. S. , Wisthaler , A. , Hansel , A. , Ball , W. P. , Dickerson , R. R. , Neususs , C. , Crutzen , P. J. and Prather , K. A. 2003 . Characterization of Carbonaceous Aerosols Outflow From India and Arabia: Biomass/Biofuel Burning and Fossil Fuel Combustion . J. Geophys. Res.—Atmospheres , 108 ( D15 ) art. no.-4485.
- Harrison , R. M. and Yin , J. X. 2000 . Particulate Matter in the Atmosphere: Which Particle Properties are Important for Its Effects on Health? . Sci. Total Environ , 249 ( 1–3 ) : 85 – 101 .
- Huang , Z. , Harrison , R. M. , Allen , A. G. , James , J. D. , Tilling , R. M. and Yin , J. X. 2004 . Field Intercomparison of Filter Pack and Impactor Sampling for Aerosol Nitrate, Ammonium, and Sulphate at Coastal and Inland Sites . Atmos. Res , 71 ( 3 ) : 215 – 232 .
- Hudson , P. K. , Murphy , D. M. , Cziczo , D. J. , Thomson , D. S. , de Gouw , J. A. , Warneke , C. , Holloway , J. , Jost , J. R. and Hubler , G. 2004 . Biomass-Burning Particle Measurements: Characteristic Composition and Chemical Processing . J. Geophys. Res.—Atmospheres , 109 ( D23 ) art. no. D23S27.
- Hutchison , G. R. , Brown , D. M. , Hibbs , L. R. , Heal , M. R. , Donaldson , K. , Maynard , R. L. , Monaghan , M. , Nicholl , A. and Stone , V. 2005 . The Effect of Refurbishing a UK Steel Plant on PM10 Metal Composition and Ability to Induce Inflammation . Respiratory Research 6 ,
- Injuk , J. , Van Grieken , R. and De Leeuw , G. 1998 . Deposition of Atmospheric Trace Elements Into the North Sea: Coastal, Ship, Platform Measurements and Model Predictions . Atmos. Environ , 32 ( 17 ) : 3011 – 3025 .
- Ledoux , F. , Laversin , H. , Courcot , D. , Courcot , L. , Zhilinskaya , E. A. , Puskaric , E. and Aboukais , A. 2006 . Characterization of Iron and Manganese Species in Atmospheric Aerosols From Anthropogenic Sources . Atmos. Res , 82 ( 3–4 ) : 622 – 632 .
- Liu , D.-Y. , Wenzel , R. J. and Prather , K. A. 2003 . Aerosol Time-of-Flight Mass Spectrometry During the Atlanta Supersite Experiment: 1. Measurements . J. Geophys. Res , 108 ( D7 ) : 8426
- Lough , G. C. , Schauer , J. J. , Park , J. S. , Shafer , M. M. , Deminter , J. T. and Weinstein , J. P. 2005 . Emissions of Metals Associated with Motor Vehicle Roadways . Environ. Sci. Technol , 39 ( 3 ) : 826 – 836 .
- Machemer , S. D. 2004 . Characterization of Airborne and Bulk Particulate From Iron and Steel Manufacturing Facilities . Environ. Sci. Technol , 38 ( 2 ) : 381 – 389 .
- Marple , V. A. , Rubow , K. L. and Behm , S. M. 1991 . A Microorifice Uniform Deposit Impactor (Moudi)—Description, Calibration, and Use . Aerosol Sci. Technol , 14 ( 4 ) : 434 – 446 .
- Mazzei , F. , D'Alessandro , A. , Lucarelli , F. , Marenco , F. , Nava , S. , Prati , P. , Valli , G. and Vecchi , R. 2006 . Elemental Composition and Source Apportionment of Particulate Matter Near a Steel Plant in Genoa (Italy). Nuclear Instruments & Methods . Physics Research Section B—Beam Interactions with Materials and Atoms , 249 : 548 – 551 .
- Moreno , T. , Jones , J. P. and Richards , R. J. 2004a . Characterisation of Aerosol Particulate Matter From Urban and Industrial Environments: Examples From Cardiff and Port Talbot, South Wales, UK . Sci. Total Environ. , 334–35 : 337 – 346 .
- Moreno , T. , Merolla , L. , Gibbons , W. , Greenwell , L. , Jones , T. and Richards , R. 2004b . Variations in the Source, Metal Content and Bioreactivity of Technogenic Aerosols: A Case Study From Port Talbot, Wales, UK . Sci. Total Environ , 333 ( 1–3 ) : 59 – 73 .
- Oravisjarvi , K. , Timonen , K. L. , Wiikinkoski , T. , Ruuskanen , A. R. , Heinanen , K. and Ruuskanen , J. 2003 . Source Contributions to PM2.5 Particles in the Urban Air of a Town Situated Close to a Steel Works . Atmos. Environ , 37 ( 8 ) : 1013 – 1022 .
- Pope , C. A. 1996 . Particulate Pollution and Health: A Review of the Utah Valley Experience . J. Exposure Anal. Environ. Epidemiol. , 6 ( 1 ) : 23 – 34 .
- Prati , P. , Zucchiatti , A. , Lucarelli , F. and Mando , P. A. 2000 . Source Apportionment Near a Steel Plant in Genoa (Italy) by Continuous Aerosol Sampling and PIXE Analysis . Atmos. Environ , 34 ( 19 ) : 3149 – 3157 .
- Qin , X. Y. , Bhave , P. V. and Prather , K. A. 2006 . Comparison of Two Methods for Obtaining Quantitative Mass Concentrations From Aerosol Time-of-Flight Mass Spectrometry Measurements . Anal. Chem , 78 ( 17 ) : 6169 – 6178 .
- Schofield , M. J. 2004 . “ Sources and Properties of Airborne Particulate Matter. Ph.D. Thesis; School of Geography, Earth and Environmental Sciences ” . Birmingham : University of Birmingham .
- Schofield , M. J. , Hodges , J. , Horne , A. and Anderson , D. A. December 2007 . “ Characterisation of Fine and Ultrafine Particles From Steelmaking Processes ” . December , 13 – 14 . Paris : Presentation at ATS International Steelmaking Conference .
- Silva , P. J. , Carlin , R. A. and Prather , K. A. 2000 . Single Particle Analysis of Suspended Soil Dust From Southern California . Atmos. Environ , 34 ( 11 ) : 1811 – 1820 .
- Silva , P. J. , Liu , D. Y. , Noble , C. A. and Prather , K. A. 1999 . Size and Chemical Characterization of Individual Particles Resulting From Biomass Burning of Local Southern California Species . Environ. Sci. Technol. , 33 ( 18 ) : 3068 – 3076 .
- Song , X. H. , Hopke , P. K. , Fergenson , D. P. and Prather , K. A. 1999 . Classification of Single Particles Analyzed by ATOFMS Using an Artificial Neural Network, ART-2A . Anal. Chem , 71 ( 4 ) : 860 – 865 .
- Su , Y. X. , Sipin , M. F. , Furutani , H. and Prather , K. A. 2004 . Development and Characterization of an Aerosol Time-of-Flight Mass Spectrometer With Increased Detection Efficiency . Anal. Chem , 76 ( 3 ) : 712 – 719 .
- Suess , D. T. and Prather , K. A. 1999 . Mass Spectrometry of Aerosols . Chemical Reviews , 99 ( 10 ) : 3007 – 3035 .
- Tsai , H.-L. , Lin , K.-H. , Chen , C.-Y. , Ding , J.-Y. , Choa , C.-J. and Chiang , H.-L. 2007 . Chemical Constituents in Particulate Emissions From an Integrated Iron and Steel Facility . J. Hazard. Mat. , 147 ( 1–2 ) : 111 – 119 .
- Van Malderen , H. , Hoornaert , S. and VanGrieken , R. 1996 . Identification of Individual Aerosol Particles Containing Cr, Pb, and Zn Above the North Sea . Environ. Sci. Technol , 30 ( 2 ) : 489 – 498 .