Abstract
An electrodynamic balance was used to investigate the uptake of octanal vapor by single sulfuric acid droplets levitated under various relative humidity (RH) conditions and gas-phase octanal concentrations. In the high octanal concentration experiments (200–300 ppm), we observed that the organic mass yield depended on the acidity of the sulfuric acid droplets and significant uptake of octanal only occurred when the RH was about 10% (H 2 SO 4 wt% ∼ 64%). Furthermore, repartitioning of only a portion of condensed organic compounds was observed after active dilution with octanal-free compressed air, indicating that low-volatility products had formed and that repartitioning is important in affecting the organic mass fraction and chemical composition of atmospheric organic aerosols. When a relatively lower octanal concentration (700–900 ppb) was used, no significant uptake of octanal vapor by the sulfuric acid droplets was observed even at 10% RH with long exposure time (>25 h). Our findings suggest that both particle acidity and gas-phase octanal concentration are the critical factors that influence the organic mass yield of levitated acidic droplets. Because of the severe conditions of low RH and high octanal conditions required to effect the reactions, the reactive uptake of aldehydes, especially those that have chemical structures and properties very similar to octanal, into acidic particles may not be an important pathway in secondary organic aerosol formation under actual atmospheric conditions.
INTRODUCTION
Atmospheric aerosols have been found to exert pronounced effects on human health and the radiative balance of the earth's atmosphere and thus on the global climate (CitationDockery et al. 1993; CitationPöschl 2005; CitationIPCC 2007). Organic aerosols are major constituents of atmospheric aerosols and their composition and properties change continuously throughout their atmospheric lifetime as a result of various physical and chemical aging processes (CitationKanakidou et al. 2005; CitationRudich et al. 2007). In addition to the large uncertainties about the emissions inventories of primary organic aerosols (POA) as well as the gas-phase precursors of secondary organic aerosols (SOA), our poor understanding of the chemical aging processes of organic aerosols also limits the accuracy and reliability of current global climate modeling (CitationKanakidou et al. 2005).
While the literature predominately describes SOA formation as a photooxidation process involving gas-phase organic precursors followed by the partitioning of gas-phase products into the particle phase, the heterogeneous reactions between gas-phase and particle-phase materials have been recognized as another vital chemical aging process of organic aerosols in the last decade. A number of recent laboratory studies have focused on investigating the aging of particulate organics in the presence of gas-phase oxidants (e.g., CitationBertram et al. 2001; CitationRudich 2003; CitationMolina et al. 2004; CitationZahardis and Petrucci 2007; CitationGeorge et al. 2007). These oxidative aging processes modify the composition of organic aerosols as well as their hygroscopicity, cloud condensation nuclei (CCN) activity and density (e.g., CitationKatrib et al. 2005; CitationPetters et al. 2006; CitationShilling et al. 2007; CitationLee and Chan 2007a,Citationb). In addition, reactive uptake of volatile organics, especially carbonyl compounds, into preexisting acidic particles has been proposed to further explain the huge abundance of SOA in the atmosphere (CitationJang et al. 2004), but their potential atmospheric importance is still under debate based on the existing particle mass yield/growth data, mechanistic, and kinetic information obtained from laboratory studies (CitationJang et al. 2001; CitationEsteve and Nozière 2005; CitationKroll et al. 2005; CitationZhao et al. 2005; CitationLiggio and Li 2006; CitationCasale et al. 2007) and thermodynamic (CitationBarsanti and Pankow 2004) and kinetic calculations (CitationErvens and Kreidenweis 2007).
CitationJang et al. (2002) first proposed the significance of acid-catalyzed reactions of volatile carbonyl compounds, such as glyoxal and octanal, in SOA formation. On the basis of a series of chamber and flow reactor experiments, Jang and coworkers concluded that the presence of sulfuric acid particles catalyzes the reactions of most carbonyl compounds to form low-vapor-pressure products in the aerosol phase and hence markedly increases the SOA mass production, via various mechanisms including hydration, aldol condensation, acetal/hemicetal formation (in the presence of alcohols), and polymerization (CitationJang and Kamens 2001; CitationJang et al. 2003a,Citationb; Citation2005). However, CitationKroll et al. (2005) did not observe any significant aerosol growth due to reactive uptake of most carbonyls that they investigated (e.g., formaldehyde, 2,4–pentanedione, octanal, etc.), which is in contrast with the findings reported by Jang and coworkers. In Kroll et al.'s work, single exception was glyoxal but they found that large glyoxal uptake is due to the high ionic strength of inorganic seed particles instead of particle acidity. Furthermore, CitationZhao et al. (2005) reported that octanal was physically absorbed by sulfuric acid without undergoing irreversible reactions although they observed irreversible reactive uptake of 2,4-hexadienal. The inconsistencies reported in the literature suggest the need of further investigation of the importance of acid-catalyzed reactions of carbonyl compounds under atmospheric conditions.
In this study, an electrodynamic balance (EDB) was used to investigate the reactions between octanal vapor and single levitated sulfuric acid droplets. The EDB has been shown to be very useful in examining particle hygroscopicity because it is very sensitive to any changes in particle mass in response to changes in the surrounding air (CitationPeng et al. 2001; CitationChoi and Chan 2002). This feature also makes the EDB an ideal tool to directly measure the mass yield (or loss) of levitated particles when they interact with gas-phase reactants (CitationLee and Chan 2007a,Citationb). The ability to levitate single particles for extended periods of time allows investigation of complex reactions, such as ozonolysis of organic acids at ozone concentrations relevant to atmospheric conditions. Another distinct advantage of the EDB in the current study is that the octanal vapor supply can be easily cut off after a certain period of exposure to examine whether any repartitioning of condensed organic materials occurs after purging with octanal-free compressed air, which has been considered in both laboratory experiments and model predictions of SOA formation (CitationPankow 1994; CitationGrieshop et al. 2007; CitationRobinson et al. 2007). This additional information may help us understand the relative importance of physical and reactive uptakes of octanal vapor by sulfuric acid droplets. Furthermore, single particle Raman spectroscopy was also used to probe the changes in chemical compositions of the levitated sulfuric acid droplets (CitationLing and Chan 2007; CitationLee et al. 2008). The experiments were conducted under different relative humidity (RH) conditions (10–50%) and gas-phase octanal concentrations (both ppb and ppm levels). The objectives of this study were (1) to determine the effects of laboratory conditions, including particle acidity and gas-phase octanal concentrations, on the organic mass yield of SOA and (2) to shed light on the atmospheric importance of the acid-catalyzed reactions of aldehydes in SOA formation.
EXPERIMENTAL METHODS
Generation of Inorganic Seed Particles
A small amount of diluted sulfuric acid solution was introduced into a piezoelectric particle generator (Uni-Photon Inc., New York, USA, Model 201). By applying electric pulses to the droplet generator, solution droplets (∼ 20–40 μ m) were generated and charged by passing them across a metal induction plate before they entered into the electrodynamic balance (EDB). Single sulfuric acid droplets were levitated and held stationary with proper adjustments of the combination of AC and DC electric fields inside the EDB. A similar procedure was used to prepare ammonium sulfate (> 99%, Sigma-Aldrich) particles in the control experiments.
Generation of Octanal Vapor
The octanal vapor was generated by passing RH-controlled compressed air over an octanal reservoir. To determine the gas-phase concentration, octanal vapor was trapped using volatile organic carbon (VOC) adsorption tubes (SKC sorbent tube) and subsequently quantified using a combination of thermal desorption (Perkin Elmer, Automated Thermal Desorber Turbo Matrix ATD) and gas chromatography-mass spectroscopy (Perkin Elmer, Clarus 500). The calibration curve was constructed by evaporating a known amount of octanal at about 50°C and then collected by a VOC adsorption tube. The octanal concentrations ranged from approximately 200 to 300 ppm in the high octanal concentration experiments. In the low and medium octanal concentration experiments, the particles were exposed to octanal vapor with concentrations about 700–900 ppb and 5–7 ppm, respectively, for more than 25 h.
The Electrodynamic Balance and Mass Yield Measurements
The principle of the EDB has been well documented (CitationDavis 1997) and therefore is not described in detail here. In brief, a charged particle with about a 20–40 μ m diameter is trapped at the null point of the cell through a combination of DC and AC electric fields surrounding the particle. Assuming that there is no loss of charge, the mass of the particle is proportional to the applied DC voltage. Any relative mass change in the particle due to any physical (e.g., evaporation or condensation of water in response to the changes in ambient RH) or chemical changes is determined by recording the DC voltage required to balance the weight of the particle. This makes the EDB as an ideal tool to measure particle hygroscopicity and mass yield (or loss) caused by chemical reactions (CitationLee and Chan 2007a,Citationb).
In this study, the sulfuric acid droplets were first equilibrated at specific RH conditions and then the relative mass changes of the levitated particles caused by physical uptake of organics and/or heterogeneous reactions were monitored at 1–2 h intervals over a whole period of octanal exposure. The experimental results are presented here in the form of mass ratios (m t /m 0), which is the ratio of the particle mass at a given octanal exposure time, m t , to the initial particle mass, m 0. The weight percentage of sulfuric acid in the levitated droplets at specific RH conditions was predicted using the Aerosol Inorganics Model (AIM) (CitationClegg et al. 1998). The RH inside the EDB was adjusted by mixing a stream of saturated air and another of dry air at controlled flow rates. The overall experimental error in the mass ratios was within 1% for droplets, and the error in the determination of RH was estimated to be ± 1% at RH = 40–80%.
Raman Spectroscopy of Single Levitated Particles
We measured the Raman scattering of levitated particles undergoing heterogeneous reactions for aerosol composition (functional group) analyses. In this study, we used a Raman spectroscopy system similar to that used in our previous study (CitationLee et al. 2008). It consisted of a 5 W argon ion laser (Coherent I90-5) and a 0.5 m monochromator (Acton SpectraPro 500) attached to a CCD (Andor Technology DV420-OE), which was integrated with the EDB system. The 514.5 nm line of an argon ion laser with output power between 25–50 mW was used as the source of excitation. A pair of lenses, which matched the f/7 optics of the monochromator, was used to focus the 90 scattering of the levitated droplet in the EDB onto the slit of the monochromator. A 514.5 nm Raman notch filter was placed between the two lenses to remove the strong Rayleigh scattering. A 300 g/mm grating of the monochromator was selected. The integration time of each spectrum was 30 s (30 frames, each with an accumulation time of 1 s). The resolution of the spectra obtained was about 6 cm− 1. All measurements were made at ambient temperatures of 22–24°C.
Light Scattering Pattern Measurements
The laser-illuminated light scattering pattern of the levitated particles was monitored and captured through the window of the EDB using a microscope (5× objective and 20× eyepiece) coupled with a digital camera (Nikon Coolpix 990). A He-Ne laser with a wavelength of 632.8 nm was used as the illuminating source. The laser beam passed through the levitated particle from the bottom of the EDB and the microscope was positioned at an angle of 90° with respect to the laser beam. The light scattering image was first focused so that two clear light spots could be observed via the eyepiece of the microscope. After that, the eyepiece was removed to obtain the laser-illuminated light scattering pattern (the particle image was actually out of focus). If the levitated particle was a homogeneous droplet, then the light scattering pattern included some sharp horizontal lines, with the number of horizontal lines depending on the particle size. If the levitated particle contained solids or crystalline materials or had an irregular coating, then the light scattering pattern became irregular and fluctuated (CitationLee and Chan 2007b). CitationParsons et al. (2006) and CitationOlsen et al. (2006) also used a similar experimental approach to study the nucleation of levitated droplets.
RESULTS AND DISCUSSION
In the current study, the uptakes of octanal vapor by single levitated sulfuric acid droplets were investigated under various RH conditions and octanal vapor concentrations using the EDB. On the basis of the observations from the high octanal concentration experiments, we first consider the effect of particle acidity on the organic mass yield of levitated sulfuric acid droplets. Second, we provide evidence of the formation of low-volatility products and the repartitioning of particle-phase organic materials. Last, the effect of gas-phase octanal concentration on the organic mass yield is discussed for the case of sulfuric acid droplets that equilibrated at 10% RH and kinetic analysis is used to evaluate the contribution of acid-catalyzed reactions of octanal in SOA formation in the atmosphere.
Effects of Particle Acidity on the Organic Mass Yield in the High Octanal Concentration Experiments
Figures 1a, b, and c show the relative mass changes of sulfuric acid droplets as a function of exposure time (first 10 h) in the high octanal concentration experiments (200–300 ppm) at 10%, 30%, and 50% RH, respectively. These octanal concentrations were several orders of magnitude higher than typical atmospheric concentrations of carbonyl compounds, which are normally on the ppb level. Significant uptakes of octanal vapor were observed in the sulfuric acid droplets equilibrated at 10% RH and their masses appreciably increased by about 20–70% of their original mass. The variation in organic mass fractions was probably due to differences in particle size and fluctuations in octanal concentrations between each individual experiment in . For the case of 30% RH, the masses of the sulfuric acid droplets slightly increased by about 2–3%. When the sulfuric acid droplets were exposed to octanal vapor at 50% RH, no significant particle mass changes were observed. Ammonium sulfate ((NH4)2SO4) aqueous droplets equilibrated at 50% RH were exposed to octanal vapor as control experiments. shows that the masses of supersaturated (NH4)2SO4 droplets remained almost unchanged after 10 h of octanal exposure at 50% RH, indicating that both physical and reactive uptakes of octanal vapor were insignificant in (NH4)2SO4 aqueous droplets even at very high octanal concentrations.
FIG. 1 The particle mass growth curves obtained in the high octanal concentration experiments (200–300 ppm) with different RH conditions and seed particle compositions. The octanal supply was cut off at 10 hr as indicated by the dash line.
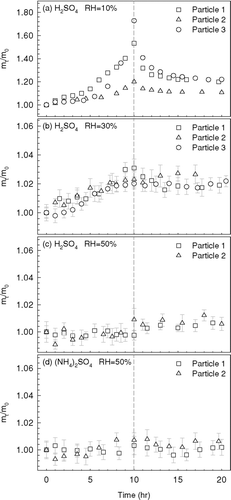
The Raman spectra of the levitated (NH4)2SO4 droplets before and after octanal exposure were almost identical as shown in , which further confirms that there was no significant uptake of octanal vapor by the supersaturated (NH4)2SO4 droplets at 50% RH. In contrast, sulfuric acid droplets equilibrated at 10% RH had strong fluorescence signals that masked all the Raman signals as shown in , which is commonly observed in Raman spectra of sulfuric acid solutions especially with high concentrations when a small amount of organic contaminants exist (CitationHegglin et al. 2002). Although the strong fluorescence signals make it difficult to identify the functional characteristics of the condensed organic materials, the fluorescence signal increases in intensity as a function of octanal exposure (), indicating the gradual formation of fluorescence products. Furthermore, distortion in the light scattering pattern was observed after the reactions at 10% RH as presented in and 3b, suggesting that the levitated droplet had inhomogeneous configuration. The organics probably formed non-uniform or partially coating on the surface of the sulfuric acid droplets (see discussion below). On the other hand, the light scattering patterns of the supersaturated (NH4)2SO4 droplets remained unchanged after exposure to octanal ( and 3d).
FIG. 2 The Raman spectra of (a) (NH4)2SO4 droplets at 50% RH and (b) H2SO4 droplets at 10% RH at different octanal exposure times. The Raman spectra were normalized by the signal from elastic scattering (514.5 nm).
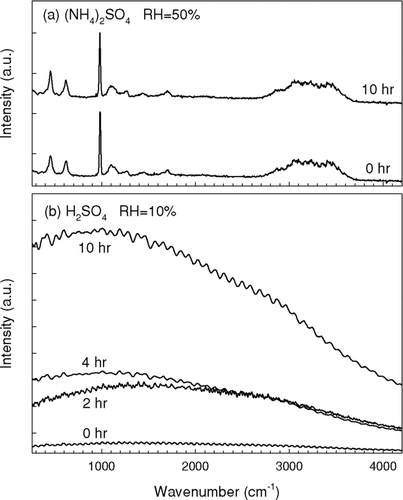
FIG. 3 The light scattering patterns of (a) and (b) H2SO4 droplets at 10% RH and (c) and (d) supersaturated (NH4)2SO4 droplets at 50% RH during different octanal exposure times.
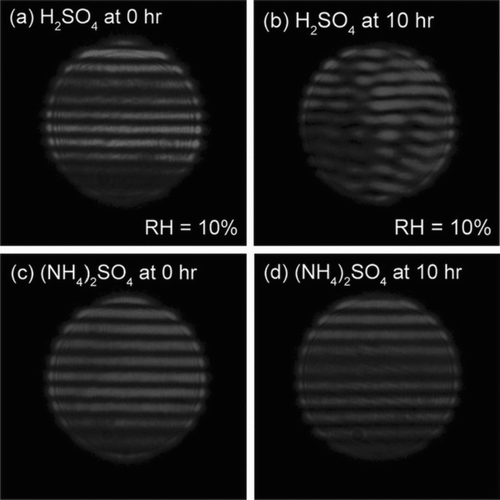
Our direct particle mass measurements clearly illustrate that the uptakes of octanal vapor by sulfuric acid droplets strongly depend on the RH of the surrounding air under such a high octanal concentrations. Since sulfuric acid is very hygroscopic in nature, the RH inside the EDB can directly influence the water content of the levitated droplets and consequently their acidity. Based on the AIM model (CitationClegg et al. 1998), we determined the initial weight percentage of sulfuric acid in the levitated droplets to be about 64, 50, and 43% when they are equilibrated at 10%, 30%, and 50% RH, respectively. Obviously, the organic mass yield increased with the particle acidity and the organic fraction markedly increased when the weight percentage of sulfuric acid reached 64%. CitationGarland et al. (2006) studied the acid-catalyzed reaction of hexanal vapor on sulfuric acid droplets. The partial pressure of hexanal ranged from 0.004 to 0.009 atm in their experiments, which are also much higher than typical ambient levels. They showed that increasing the weight percentage of sulfuric acid leads to more organic materials being incorporated into the droplets.
Formation of Low-Volatility Products and Repartitioning of Condensed Organic Materials in the High Octanal Concentration Experiments
To examine whether the organic materials accumulated in the particles evaporated back into the gas phase when the octanal was no longer supplied, the EDB was purged with octanal-free compressed air after the reactions took place for about 10 hrs. At 30% RH, no significant changes in particle mass were observed during the purging period as shown in , indicating that the formed organic materials were non-volatile and the overall uptake process was irreversible under ambient temperatures. In contrast, a dramatic drop in particle mass was observed in the first two hours of purging in the case of 10% RH and over 50% of the condensed organic materials ultimately evaporated back into the gas phase (). About 10–25% and 2–3% of the organic mass remained after purging in the 10% RH and 30% RH experiments, respectively. These observations clearly demonstrate that low-volatility products that tend to remain in the particle phase formed and that a portion of particle-phase organic materials repartitioned under the octanal-free environment.
The uptake behavior of octanal vapor at 10% RH is shown in . The organic mass of the levitated droplets increased slowly during the first 5–6 h of exposure while a two-fold or higher increase in the uptake rate was observed after 6 h. Our hypothesis is that the physical uptake of octanal vapor is limited at the beginning of exposure because octanal has a high vapor pressure (on the order of 10− 4 atm) and an extremely low solubility in aqueous solutions. However, as larger amounts of less-volatile organic compounds are formed in the particles due to high acidity (e.g., at 10% RH), their accumulation on the particle surface modifies the chemical properties of the droplets, such as the mass accommodation coefficient and hydrophilicity (CitationDonaldson and Vaida 2006), enhancing the condensation growth (physical uptake) of octanal. The overall uptake process of octanal vapor is therefore predominantly driven by chemical reactions in the first 5–6 h and then physical uptake afterwards ().
FIG. 4 Uptake behavior of octanal vapor by H2SO4 droplets at 10% RH. Significant changes in the octanal uptake rates were observed after about 5–6 hrs of octanal exposure (200–300 ppm).
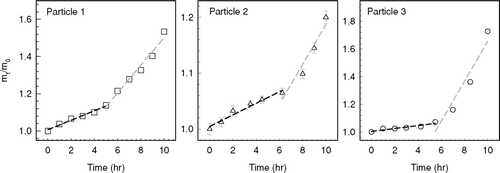
Since octanal and reaction products are immiscible with 64 wt% of sulfuric acid as observed in our bulk liquid investigation (CitationLi et al. 2008), we expected the acid-catalyzed reaction of octanal to occur at the organic-aqueous interface instead of inside the solution droplets. As the time for all octanal to undertake the reaction in the organic–aqueous interface to form low-volatility products may be insufficient, a portion of the octanal rapidly evaporated into the gas phase after active dilution with octanal-free compressed air, which explains the partial repartitioning of particle-phase organics as shown in . Some volatile/semi-volatile products (if any) may also escape to the gas phase during the purging period but their contribution to the overall evaporative loss is very small since the acid-catalyzed reactions of octanal do not likely generate high volatility products.
The absorptive gas-particle partitioning of SOA formed from the condensation of semi-volatile oxidation products of gas-phase precursors has been included as a reversible process at equilibrium in current models (e.g., CitationPankow 1994; CitationOdum et al. 1996). However, CitationTsigaridis and Kanakidou (2003) pointed out that there is substantial uncertainty in SOA predictions because of the repartitioning of low-volatility particle-phase organics formed by heterogeneous reactions. Similar to the current study, partial repartitioning of condensed organic matter was experimentally verified in some recent studies on POA and SOA formation. For instance, CitationGrieshop et al. (2007) investigated the reversibility of gas-particle partitioning in SOA formed from α -pinene ozonolysis in a smog chamber using the isothermal dilution approach. Their results demonstrated that only a portion of SOA formed from α -pinene ozonolysis repartitions reversibly when active dilution reduces the gas-phase concentration of semi-volatile organic species and the SOA composition varies systematically with partitioning based on their aerosol mass spectrometer data. CitationRobinson et al. (2007) presented diesel exhaust data measured at different levels of atmospheric dilutions. In that work, the POA emission factor decreased with increasing dilution ratios because of the evaporation of semi-volatile organic compounds to maintain the phase equilibrium. Their observation also suggests that the repartitioned primary organics are probably oxidized and re-condensed into the particle phase, consequently enhancing SOA formation.
Effects of Gas-Phase Octanal Concentration on Organic Mass Yield
Although we observed the dependence of organic mass enhancement on acidity and the reversible partitioning phenomenon in the high octanal concentration experiments, it is particularly important to determine if a significant organic mass yield can be obtained under more realistic atmospheric carbonyl concentrations. For this reason, the cases of relatively lower octanal concentrations (700–900 ppb) and highly acidic sulfuric acid droplets (64 wt% at 10% RH) were also investigated in the current study. After 25 h of low octanal exposure at 10% RH, insignificant changes in particle mass were observed (), indicating that both physical and reactive uptakes were not important under such low octanal concentrations. The same conclusion was drawn when the medium octanal concentration (5–7 ppm) was used as shown in .
FIG. 5 The particle mass growth curves of H2SO4 droplets obtained in the low (700–900 ppb, triangles and circles) and medium (5–7 ppm, squares) octanal concentration experiments at 10% RH conditions.
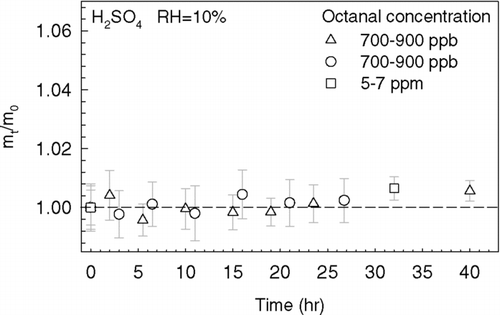
By incorporating our findings from the high octanal concentration experiments with those the more realistic experiments, we suggest that gas-phase octanal concentration is a critical factor, in addition to the particle acidity, that influences the organic mass fraction of levitated acidic droplets. In our previous study, we investigated heterogeneous reactions between octanal vapor and deposited sulfuric acid droplets using a reaction flow cell and offline GC-MS characterization. A similar conclusion on the effect of gas-phase organic concentration on SOA formation was drawn (CitationLi et al. 2008). In that study, none of the accretion reaction products, which have relatively high molecular weight and low vapor pressure, were detected even at RH less than 1% if ∼ 900 ppb of octanal vapor was employed in the gas–particle experiments. In addition, at 30% RH (H2SO4 wt% ∼ 50%), no accretion reaction products were observed after exposing the deposited sulfuric acid droplets to ∼ 20 ppm of octanal vapor whereas cyclotrimerization products were dominant in the bulk experiments under these acidic conditions. The discrepancy between the bulk and the gas–particle experiments is probably due to the limited availability of octanal in the deposited sulfuric acid droplet experiments. This observation suggests the need to re-examine the reaction mechanisms proposed in the literature that is based on bulk experiments.
Some previous laboratory studies also investigated the uptake of octanal vapor using different techniques and their conclusions are somewhat controversial. CitationKroll et al. (2005) reported negligible growth when mixed ammonium sulfate/sulfuric acid droplets were exposed to 500 ppb of octanal vapor at RH approximately equal to 50% in their smog chamber experiments. CitationZhao et al. (2005) examined the heterogeneous uptake of octanal vapor on a 60–85 wt% sulfuric acid solution using an octanal concentration on the order of 10− 1 ppm. They found that octanal was physically absorbed by sulfuric acid without undergoing an irreversible reactive uptake process. These results are generally in a good agreement with our observations. Furthermore, CitationBarsanti and Pankow (2004) considered the thermodynamics of the accretion reactions of octanal, including hydration, aldol condensation, acetal/hemicetal formation, and polymerization, and they concluded that these accretion reactions are not thermodynamically favored either in the real atmospheric environment or under the experimental conditions used by CitationJang and Kamens (2001). On the contrary, Jang and coworkers reported a significant growth in sulfuric acid droplets in a number of carbonyl compounds including octanal under various RH conditions, aldehyde concentrations and inorganic seed particle compositions (CitationJang and Kamens 2001; CitationJang et al. 2003a,Citationb; Citation2005). Even at a relatively low octanal concentration (∼ 230 ppb at 20% RH), they observed significant aerosol growth (CitationJang et al. 2005). It is obvious that further research is needed to understand the discrepancies between these studies.
Kinetic Analysis
Aldol condensation has been suggested to be one of the most important reactions of aldehydes in acidic solutions and the reaction mechanisms are described by CitationCasale et al. (2007). Following CitationErvens and Kreidenweis (2007), who assume that the rate-limiting step in aldol condensation is the particle phase reaction between the aldehyde and its corresponding enolization form, the reaction rate can be calculated as,
CONCLUSIONS AND ATMOSPHERIC IMPLICATIONS
In this study, we observed two phenomena in the high octanal concentration experiments (200–300 ppm). First, we found that the organic mass yield depends on the acidity of the sulfuric acid droplets and significant uptake of octanal can be only observed when the RH inside the EDB is equilibrated at about 10% (H2SO4 wt% ∼ 64%). Some possible mechanisms of acid-catalyzed reactions of aldehyde have been proposed in the literature (e.g., CitationJang et al. 2002; CitationGarland et al. 2006; CitationLiggio and Li 2006). Although the mechanisms of heterogeneous acid–catalyzed reactions of aldehyde still remain uncertain based on the existing laboratory measurements, thermodynamic and kinetic calculations (CitationBarsanti and Pankow 2004; CitationCasale et al. 2007; CitationErvens and Kreidenweis 2007; CitationLi et al. 2008), it is reasonable that our observed organic mass yield (after purging) is mainly caused by the formation of high molecular weight products with relatively low vapor pressure.
Second, we have evidence to show the occurrence of partial repartitioning of condensed organic compounds under active dilution of octanal-free compressed air at 10% RH. In the atmosphere, the continuous uptake and release of volatile/semi-volatile organics probably occur throughout the particles' lifetime as the gas-phase concentrations of organic species vary continuously. In addition, the current study and some recent studies (CitationGrieshop et al. 2007; CitationRobinson et al. 2007) demonstrated that the phenomenon of partial repartitioning should be considered in the POA/SOA prediction model and laboratory studies of organic aerosol formation, especially when a relatively high gas phase concentration of organics is employed to compensate for a short exposure time. Otherwise, the predicted and the measured organic aerosol yield caused by the heterogeneous processes may be overestimated.
Although the octanal concentration in the low concentration experiments (700–900 ppb) is much higher than typical atmospheric concentrations, there was no significant uptake of octanal vapor by the sulfuric acid that equilibrated even at 10% RH and a long exposure time (> 25 h). Since sulfuric acid droplets equilibrated at 10% RH can provide sufficient acidity to facilitate reactive uptake in the high octanal concentration experiments, the gas-phase octanal concentration is another critical factor that influences the organic mass fraction of levitated acidic droplets. On the basis of the experimental observations and the kinetic calculations reported here, we conclude that the uptake of octanal vapor by sulfuric acid droplets is only important under the condition when both a high gas-phase octanal concentration and highly acidic particles exist at the same time. These two criteria may be also applicable to the case of other carbonyl compounds, especially those that have chemical structures and properties very similar to octanal.
The significant enhancement of octanal uptake in the latter period of exposure (at 10% RH and 200–300 ppm octanal) is another observation that requires further discussions. This change in uptake is probably due to the accumulation of hydrophobic organic products, which significantly enhances the condensation of volatile/semi-volatile hydrophobic organics, such as octanal in this study, onto the levitated sulfuric acid droplets. This observation implies that heterogeneous chemical processes are a potentially important pathway for incorporating hydrophobic organics into hydrophilic aerosols, and hence modifying the physical uptake properties of volatile/semi-volatile hydrophobic organics of atmospheric aerosols. Although this hydrophilic-to-hydrophobic conversion may not happen under real atmospheric conditions for the case of octanal as reported here, some gas-phase biogenic organics (e.g., isoprene, α -pinene, etc.) and their photooxidation products (e.g., pinoaldehyde) possibly react with acidic particles under atmospheric conditions to facilitate such conversion (CitationLiggio and Li 2006; CitationLiggio et al. 2007; CitationSurratt et al. 2007). Furthermore, physical uptake enhancement may be important to affect the atmospheric gas-phase and heterogeneous chemistry as the volatile/semi-volatile hydrophobic organics would have a higher tendency to partition into the particle phase and subsequently react with atmospheric oxidants or reactants.
This work was supported by the Research Grants Council of the Hong Kong Special Administrative Region China (Project No. HKUST600303).
REFERENCES
- Barsanti , K. C. and Pankow , J. F. 2004 . Thermodynamics of the Formation of Atmospheric Organic Particulate Matter by Accretion Reactions—Part 1: Aldehydes and Ketones . Atmos. Environ. , 38 : 4371 – 4382 .
- Bertram , A. K. , Ivanov , A. V. , Hunter , M. , Molina , L. T. and Molina , M. J. 2001 . The Reaction Probability of OH on Organic Surfaces of Tropospheric Interest . J. Phys. Chem. A , 105 : 9415 – 9421 .
- Casale , M. T. , Richman , A. R. , Elrod , M. J. , Garland , R. M. , Beaver , M. R. and Tolbert , M. A. 2007 . Kinetics of Acid-Catalyzed Aldol Condensation Reactions of Aliphatic Aldehydes . Atmos. Environ. , 41 : 6212 – 6224 .
- Choi , M. Y. and Chan , C. K. 2002 . The Effects of Organic Species on the Hygroscopic Behaviors of Inorganic Aerosols . Environ. Sci. Technol. , 36 : 2422 – 2428 .
- Clegg , S. L. , Brimblecombe , P. and Wexler , A. S. 1998 . A Thermodynamic Model of The System H+–NH4 +–SO4 2 −–NO3 −–H2O at Tropospheric Temperatures . J. Phys. Chem. A , 102 : 2137 – 2154 .
- Davis , E. J. 1997 . A History of Single Aerosol Particle Levitation . Aerosol Sci. Technol. , 26 : 212 – 254 .
- Dockery , D. W. , Pope , C. A. , Xu , X. P. , Spengler , J. D. , Ware , J. H. , Fay , M. E. , Ferris , B. G. and Speizer , F. E. 1993 . An Association Between Air Pollution and Mortality in Six U.S. Cities . N. Engl. J. Med. , 329 : 1753 – 1759 .
- Donaldson , D. J. and Vaida , V. 2006 . The Influence of Organic Films at the Air–Aqueous Boundary on Atmospheric Processes . Chem. Rev. , 106 : 1445 – 1461 .
- Ervens , B. and Kreidenweis , S. M. 2007 . SOA Formation by Biogenic and Carbonyl Compounds: Data Evaluation and Application . Environ. Sci. Technol. , 41 : 3904 – 3910 .
- Esteve , W. and Nozière , B. 2005 . Uptake and Reaction Kinetics of Acetone, 2-Butanone, 2,4-Pentanedione, and Acetaldehyde in Sulfuric Acid Solutions . J. Phys. Chem. A , 109 : 10920 – 10928 .
- Garland , R. M. , Elrod , M. J. , Kincaid , K. , Beaver , M. R. , Jimenez , J. L. and Tolbert , M. A. 2006 . Acid-Catalyzed Reactions of Hexanal on Sulfuric Acid Particles: Identification of Reaction Products . Atmos. Environ. , 40 : 6863 – 6878 .
- George , I. J. , Vlasenko , A. , Slowik , J. G. , Broekhuizen , K. and Abbatt , J. P. D. 2007 . Heterogeneous Oxidation of Saturated Organic Aerosols by Hydroxyl Radicals: Uptake Kinetics, Condensed-Phase Products, and Particle size change . Atmos. Chem. Phys. , 7 : 4187 – 4201 .
- Grieshop , A. P. , Donahue , N. M. and Robinson , A. L. 2007 . Is the Gas–Particle Partitioning in Alpha–Pinene Secondary Organic Aerosol Reversible? . Geophys. Res. Lett. , 34 : L14810 doi:10.1029/2007GL029987.
- Hegglin , M. I. , Krieger , U. K. , Koop , T. and Peter , T. 2002 . Technical Note: Organics-Induced Fluorescence in Raman Studies of Sulfuric Acid Aerosols . Aerosol Sci. Technol. , 36 : 510 – 512 .
- Intergovernmental Panel on Climate Change (IPCC) . 2007 . “ Contribution of Working Group I to the Fourth Assessment Report of the IPCC ” . In Climate Change 2007: The Physical Science Basis , Edited by: Solomon , S. , Qin , D. , Manning , M. , Chen , Z. , Marquis , M. , Averyt , K. B. , Tignor , M. and Miller , H. L. Cambridge University Press . Cambridge and New York
- Jang , M. and Kamens , R. M. 2001 . Atmospheric Secondary Aerosol Formation by Heterogeneous Reactions of Aldehydes in the Presence of a Sulfuric Acid Aerosol Catalyst . Environ. Sci. Technol. , 35 : 4758 – 4766 .
- Jang , M. , Czoschke , N. M. , Lee , S. and Kamens , R. M. 2002 . Heterogeneous Atmospheric Aerosol Production by Acid-Catalyzed Particle Phase Reactions . Science , 298 : 814 – 817 .
- Jang , M. , Carroll , B. , Chandramouli , B. and Kamens , R. M. 2003a . Particle Growth by Acid-Catalyzed Heterogeneous Reactions of Organic Carbonyls on Preexisting Aerosols . Environ. Sci. Technol. , 37 : 3828 – 3837 .
- Jang , M. , Lee , S. and Kamens , R. M. 2003b . Organic Aerosol Growth by Acid-Catalyzed Heterogeneous Reactions of Octanal in a Flow Reactor . Atmos. Environ. , 37 : 2125 – 2138 .
- Jang , M. , Czoschke , N. M. and Northcross , A. L. 2004 . Atmospheric Organic Aerosol Production by Heterogeneous Acid-Catalyzed Reactions . Chem. Phys. Chem. , 5 : 1647 – 1661 .
- Jang , M. , Czoschke , N. M. and Northcross , A. L. 2005 . Semiempirical Model for Organic Aerosol Growth by Acid-Catalyzed Heterogeneous Reactions of Organic Carbonyls . Environ. Sci. Technol. , 39 : 164 – 174 .
- Kanakidou , M. , Seinfeld , J. H. , Pandis , S. N. , Barnes , I. , Dentener , F. J. , Facchini , M. C. , Van Dingenen , R. , Ervens , B. , Nenes , A. , Nielsen , C. J. , Swietlicki , E. , Putaud , J. P. , Balkanski , Y. , Fuzzi , S. , Horth , J. , Moortgat , G. K. , Winterhalter , R. , Myhre , C. E. L. , Tsigaridis , K. , Vignati , E. , Stephanou , E. G. and Wilson , J. 2005 . Organic Aerosol and Global Climate Modeling: A Review . Atmos. Chem. Phys. , 5 : 1053 – 1123 .
- Katrib , Y. , Martin , S. T. , Rudich , Y. , Davidovits , P. , Jayne , J. T. and Worsnop , D. R. 2005 . Density Changes of Aerosol Particles as a Result of Chemical Reaction . Atmos. Chem. Phys. , 5 : 275 – 291 .
- Kroll , J. H. , Ng , N. L. , Murphy , S. M. , Varutbangkul , V. , Flagan , R. C. and Seinfeld , J. H. 2005 . Chamber Studies of Secondary Organic Aerosol Growth by Reactive Uptake of Simple Carbonyl Compounds . J. Geophys. Res. , 110 : D23207 doi:10.1029/2005JD006004.
- Lee , A. K. Y. and Chan , C. K. 2007a . Single Particle Raman Spectroscopy for Investigating Atmospheric Heterogeneous Reactions of Organic Aerosols . Atmos. Environ. , 41 : 4611 – 4621 .
- Lee , A. K. Y. and Chan , C. K. 2007b . Heterogeneous reactions of Linoleic Acid and Linolenic Acid Particles with Ozone: Reaction Pathways and Changes in Particle Mass, Hygroscopicity, and morphology . J. Phys. Chem. A , 111 : 6285 – 6295 .
- Lee , A. K. Y. , Ling , T. Y. and Chan , C. K. 2008 . Understanding Hygroscopic Growth and Phase Transformation of Aerosols Using Single Particle Raman Spectroscopy in an Electrodynamic Balance . Faraday Discuss. , 137 : 245 – 263 .
- Li , Y. J. , Lee , A. K. Y. , Lau , A. P. S. and Chan , C. K. 2008 . Accretion Reactions of Octanal Catalyzed by Sulfuric Acid: Product Identification, Reaction Pathways and Atmospheric Implications . Environ. Sci. Technol. Accepted , doi: 10.1021/es7031373.
- Ling , T. Y. and Chan , C. K. 2007 . Formation and Transformation of Metastable Double Salts From the Crystallization of Mixed Ammonium Nitrate and Ammonium Sulfate Particles . Environ. Sci. Technol. , 41 : 8077 – 8083 .
- Liggio , J. and Li , S.-M. 2006 . Reactive Uptake of Pinonaldehyde on Acidic Aerosols . J. Geophys. Res. , 111 : D24303 doi:10.1029/2005JD006978.
- Liggio , J. , Li , S.-M. , Brook , J. R. and Mihele , C. 2007 . Direct Polymerization of Isoprene and α-Pinene on Acidic Aerosols . Geophys. Res. Lett. , 34 : L05814 doi:10.1029/2006GL028468.
- Molina , M. J. , Ivanov , A. V. , Trakhtenberg , S. and Molina , L. T. 2004 . Atmospheric Evolution of Organic Aerosol . Geophys. Res. Lett. , 31 : L22104 doi:10.1029/2004GL020910.
- Odum , J. R. , Hoffmann , T. , Bowman , F. , Collins , D. , Flagan , R. C. and Seinfeld , J. H. 1996 . Gas/Particle Partitioning and Secondary Organic Aerosol Yields . Environ. Sci. Technol. , 30 : 2580 – 2585 .
- Olsen , A. P. , Flagan , R. C. and Kornfield , J. A. 2006 . Single-Particle Levitation System for Automated Study of Homogeneous Solution Nucleation . Rev. Sci. Instrum. , 77 : 073901
- Pankow , J. F. 1994 . An Absorption-Model of the Gas Aerosol Partitioning Involved in the Formation of Secondary Organic Aerosol . Atmos. Environ. , 28 : 189 – 193 .
- Parsons , M. T. , Riffell , J. L. and Bertram , A. K. 2006 . Crystallization of Aqueous Inorganic-Malonic Particles: Nucleation Rates, Dependence on Size, and Dependence of the Ammonium-to-Sulfate Ratio . J. Phys. Chem. A , 110 : 8108 – 8115 .
- Peng , C. , Chan , M. N. and Chan , C. K. 2001 . The Hygroscopic Properties of Dicarboxylic and Multifunctional Acids: Measurements and UNIFAC Predictions . Environ. Sci. Technol. , 35 : 4495 – 4501 .
- Petters , M. D. , Prenni , A. J. , Kreidenweis , S. M. , DeMott , P. J. , Matsunaga , A. , Lim , Y. B. and Ziemann , P. J. 2006 . Chemical Aging and the Hydrophobic-to-Hydrophilic Conversion of Carbonaceous Aerosol . Geophys. Res. Lett. , 33 : L24806 doi:10.1029/2006GL027249.
- Pöschl , U. 2005 . Atmospheric Aerosols: Composition, Transformation, Climate and Health Effects . Angew. Chem.—Int. Edit. , 44 : 7520 – 7540 .
- Robinson , A. L. , Donahue , N. M. , Shrivastava , M. K. , Weitkamp , E. A. , Sage , A. M. , Grieshop , A. P. , Lane , T. E. , Pierce , J. R. and Pandis , S. N. 2007 . Rethinking Organic Aerosols: Semivolatile Emissions and Photochemical Aging . Science , 315 : 1259 – 1262 .
- Rudich , Y. 2003 . Laboratory Perspectives on the Chemical Transformations of Organic Matter in Atmospheric Particles . Chem. Rev. , 103 : 5097 – 5124 .
- Rudich , Y. , Donahue , N. M. and Mental , T. F. 2007 . Aging of Organic Aerosol: Bridging the Gap Between Laboratory and Field Studies . Annu. Rev. Phys. Chem. , 58 : 321 – 352 .
- Shilling , J. E. , King , S. M. , Mochida , M. and Martin , S. T. 2007 . Mass Spectral Evidence That Small Changes in Composition Caused by Oxidative Aging Processes alter Aerosol CCN Properties . J. Phys. Chem. A , 111 : 3358 – 3368 .
- Surratt , J. D. , Lewandowski , M. , Offenberg , J. H. , Jaoui , M. , Kleindienst , T. E. , Edney , E. O. and Seinfeld , J. H. 2007 . Effect of Acidity on Secondary Organic Aerosol Formation from Isoprene . Environ. Sci. Technol. , 41 : 5363 – 5369 .
- Tsigaridis , K. and Kanakidou , M. 2003 . Global Modeling of Secondary Organic Aerosol in the Troposphere: A Sensitivity Analysis . Atmos. Chem. Phys. , 3 : 1849 – 1869 .
- Zahardis , J. and Petrucci , G. A. 2007 . The Oleic Acid-Ozone Heterogeneous Reaction System: Products, Kinetics, Secondary Chemistry, and Atmospheric Implications of a Model System—A Review . Atmos. Chem. Phys. , 7 : 1237 – 1274 .
- Zhao , J. , Levitt , N. P. and Zhang , R. Y. 2005 . Heterogeneous Chemistry of Octanal and 2,4-Hexadienal with Sulfuric Acid . Geophys. Res. Lett. , 32 : L09802 doi:10.1029/2004GL022200.