Abstract
The effect of working fluid on size-dependent activation efficiencies with the laminar flow ultrafine condensation particle counter described by CitationStolzenburg and McMurry (1991) was studied theoretically and experimentally. Criteria considered include tendency to avoid homogeneous nucleation within the condenser and toxicity. The working fluids that were identified have vapor pressures below that of butanol, so particles grow to smaller sizes and are more difficult to detect optically. Therefore we use a second, conventional CPC as a “booster” to grow particles to a detectable size. Experiments were performed to obtain the size- and material-dependent activation efficiencies for ethylene glycol, diethylene glycol, propylene glycol, oleic acid, and DOS. Using diethylene glycol and oleic acid, values of the 50% activation efficiency diameter, Dp50 , for negatively charged particles generated by evaporating sodium chloride, ammonium sulfate, and silver were < 1.2 (< 0.8) nm, 1.4–1.5 (1.0–1.2) nm, and 1.9–2.0 (1.5) nm as mobility (mass) diameter, respectively. The stability of the UCPC for long-term operation using ethylene glycol and propylene glycol as working fluids was tested by monitoring the instrument's response to silver particles having size near Dp50 in an air stream at 40–45% relative humidity. The performance was steady (±3%) for several days indicating the instrument performs stably during unattended operation for realistic atmospheric sampling conditions.
INTRODUCTION
The development of condensation particle counters (CPCs) has a history that spans more than a century, extending back to the original work of John Aitken in 1888 (CitationMcMurry 2000). CPCs are generally categorized into two groups: (adiabatic) expansion and continuous flow. In expansion type CPCs, the sampled aerosol is trapped in a chamber, saturated with a vapor (typically water), and then quickly expanded to produce the supersaturated vapor that condenses on particles, causing them to grow to a size that can be detected optically. In continuous flow CPCs the supersaturation is achieved either by saturating and subsequently cooling the sampled aerosol, or by blending the cool aerosol with warm air that contains the saturated vapor. Regardless of configuration, the fundamentals of the operation are the same for all CPC types. The sampled aerosol is saturated with a vapor (the “working fluid”), a method is found to cause the working fluid to become supersaturated, and the supersaturated vapor then heterogeneously nucleates on particles causing them to grow by condensation to a size ∼ 10 μ m or so. The growth takes place in less than a second, and the grown particles are detected by light scattering.
The Kelvin Equation establishes a thermodynamic relationship between the degree of supersaturation and the minimum particle size above which particles will grow indefinitely by heterogeneous nucleation (CitationSeinfeld and Pandis 1998; CitationFriedlander 2000). The particle composition is generally different from the working fluid; therefore, the minimum detectable size is influenced by the particle solubility in the condensing vapor, the wettability of the particle surface by the condensing vapor, and the charge state of the particles. Recent theoretical and experimental work shows that these effects become stronger as the sizes of sampled particles decrease (CitationHienola et al. 2007; CitationKulmala et al. 2007). CitationWilson (1900) was the first to show that for water, the degree of supersaturation required to activate ionic molecular clusters is lower than for homogeneous nucleation, and that negative ions activate more readily than positive ions. Winkler et al. (2007; 2008) studied the activation of mobility-classified nanoparticles by propanol. Similar to CitationWilson (1900), they found that negatively charged particles are activated more readily than positively charged particles, and that neutral particles are more difficult activate than charged ones.
CPCs offer the advantage of high sensitivity and high signal-to-noise since they can count individual particles, and because no counts are detected if no particles are present. The major limitation of CPCs is the minimum size that can be detected. Efforts have been made in recent years to decrease the size detection limit of continuous flow CPCs. Continuous flow CPCs include laminar flow (LCPC) and turbulent mixing (MCPC) instruments. Gamero-Castano and Fernandez de la Mora (2000; 2002) have shown that MCPCs using dibutyl phthalate (DBP) can activate the growth of tetraheptyl ammonium bromide positive ions (0.9–2.5 nm as mass diameter) and tetramethyl ammonium positive ions (0.4–0.5 nm as mass diameter). As the saturation ratio inside a mixing region was increased the particle counts approached a plateau indicating that 100% of these ions inside the mixing region were activated. CitationKim et al. (2003) described an ethylene glycol MCPC that detects sodium chloride particles down to 1.6 nm mobility diameter (1.2 nm geometric size) with an efficiency of 100%. MCPCs have only seen limited use for atmospheric field measurement campaigns since their stability over long hours of operations is not well established. On the other hand, the TSI 3025 ultrafine CPC (UCPC), which was originally developed by CitationStolzenburg and McMurry (1991), is known to perform stably over extended periods of unattended operation. The lowest size detection limit of this UCPC is about 2.5–3.0 nm (CitationBartz et al. 1985; CitationKesten et al. 1991; CitationStolzenburg and McMurry 1991; CitationWiedensohler et al. 1997; CitationAnkilov et al. 2002; CitationKulmala et al. 2007). This laminar flow CPC is used routinely for atmospheric measurements.
Our goal was to extend the minimum detection limit of laminar flow CPCs to smaller sizes by using working fluids other than butanol. Using theory and available experimental data, CitationMagnusson et al. (2003) concluded that working fluids having high surface tension (e.g., water and glycerol) could potentially lower the size detection limit of CPCs. In this article we describe a procedure for identifying working fluids that can activate sub-2 nm particles without nucleating homogeneously. After identifying suitable working fluids we then carried out experiments to obtain the dependence of activation efficiencies on particle size, charge, and composition. A simple but accurate data inversion scheme that was used to obtain activation efficiencies from our measurements is also described. Finally, we experimentally investigated the stability of our new laminar flow CPC for extended periods of unattended operation.
THEORETICAL ANALYSIS
Method
The activation efficiency of the ultrafine CPC was simulated using the method described by CitationStolzenburg (1988). The method analytically solves the mass and heat transfer equations in a fully developed laminar flow accounting for radial conduction/diffusion. is a schematic of the domains where the temperature and vapor concentration inside that UCPC are simulated.
FIG. 1 Schematic of the domains where the temperature and vapor concentration inside the UCPC are simulated.
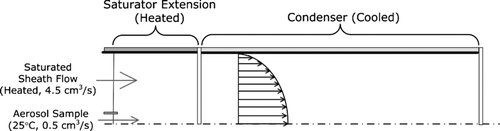
The solution gives the axisymmetric two-dimensional profile of temperature and vapor concentration within the saturator extension and condenser. Using these solutions the minimum particle size that can be activated, which is defined as the Kelvin diameter in this work, is calculated at each location within the condenser. We have not attempted to account for the solubility or wettability of the working fluid on detected particles, effects which will cause the activated size to be different from the Kelvin diameter. Chapter 5 of CitationStolzenburg (1988) describes in detail the method to calculate the activated fraction of the particles having these Kelvin diameters.
The properties of the working fluid needed to calculate activation efficiencies are: saturation vapor pressure as a function of temperature, surface tension, liquid density, molecular weight, and diffusion coefficient. For the working fluids investigated in this study all of these properties including those needed to calculate diffusion coefficient are directly obtained from the property table of CitationYaws (1999). The method of CitationWilke and Lee (1955), which is described in CitationReid et al. (1987), was used to calculate the diffusion coefficient of the working fluid vapor. Simulations were performed using all organic compounds found in the table of CitationYaws (1999) that have melting points below 20°C and boiling points above 60°C. Since there are above 800 organic compounds that satisfy these conditions, it was helpful to use the following parameterized form, which accurately represents calculated activation efficiencies, to summarize our results:
The flow configuration including the length of saturator and condenser are identical to those of the ultrafine CPC (CitationStolzenburg and McMurry 1991): 5.0 cm3/s of total flow, 0.5 cm3/s of capillary flow, 2.2 cm length of saturator extension, 11.4 cm length of condenser, and 0.46 cm inner diameter of the cylindrical condenser. For working fluids with a melting point below 10°C the condenser temperature was set to 10°C, which is the same as the condenser temperature of commercially available UCPC. The saturator temperature was changed until the calculated particle count rate due to homogeneous nucleation from the condenser equals 1 particle min–1. The overall nucleation rate was found by integrating the classical homogenous nucleation rate, J (time−1-volume−1), over the entire condenser volume.
RESULTS
shows the frequency distribution of the 50% cutoff sizes of the activation curve, D p50, for all of the organic compounds analyzed. Note that all of these organic compounds are predicted to activate particles below 4 nm and most would activate particles as small as 3 nm.
FIG. 2 The frequency distribution of the 50% activation sizes (D p50) of 863 organic compounds (CitationYaws 1999). These results apply to all compounds from that compilation that have melting and boiling points below 20°C and 60°C, respectively.
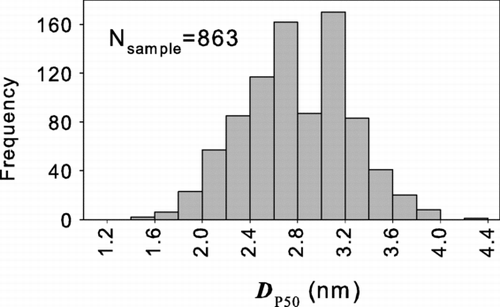
The correlations between D p50 and selected properties of the working fluids were investigated; these properties were surface tension, saturation vapor pressure, molecular mass, and diffusion coefficient. The analysis showed that molecular mass and diffusion coefficient are both weakly correlated with D p50. The surface tension had the strongest correlation with the calculated D p50 among the four properties considered. shows the calculated D p50 as a function of surface tension.
FIG. 3 The calculated 50% activation size, D p50, versus the surface tension evaluated at the temperature equal to the average of the saturator and condenser temperatures.
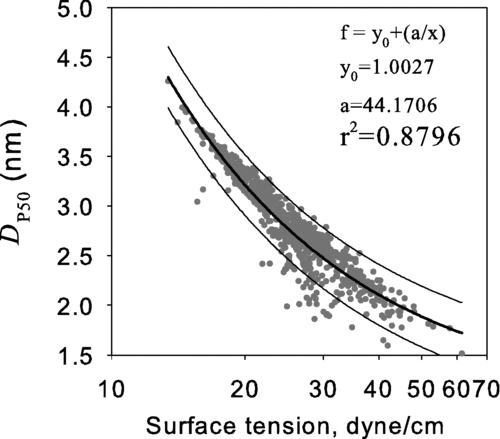
The decreasing trend of D p50 with increasing surface tension occurs because higher surface tensions increase the height of free energy barrier for gas-to-particle conversion. In effect, higher surface tensions suppress homogeneous nucleation by increasing the evaporation rates from subcritical clusters. The logarithm of the saturation vapor pressure had the second strongest correlation with the calculated D p50. shows the saturation ratio versus the saturation vapor pressure where the saturation ratio is the highest along the axis of the condenser. As shown in the figure, a strong correlation is observed between these two parameters. The lower the vapor pressure of a working fluid the lower the collision rate among the working fluid vapor molecules; therefore, a higher value of saturation ratio is needed for the rate of homogeneous nucleation rate to become significant. Since the value of D p50 is based on the Kelvin relation in this work, the higher saturation ratio generally results in lower value of D p50 as shown in .
FIG. 4 (a) The calculated saturation ratio (b) the calculated 50% activation size, D p50, versus the saturation vapor pressure where the saturation ratio is the highest along the axis of the condenser.
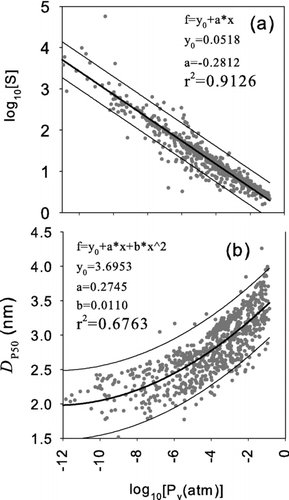
In addition we have imposed the following two practical constraints on working fluids. First, the working fluid must be relatively non-hazardous, since a certain amount of exposure to skin or by inhalation is inevitable. Second, only compounds with room-temperature viscosities of less than 50 centipoise were included, since more viscous liquids would be less effective at wetting the tubular wick in the saturator by capillary action. Finally, priority was given to working fluids that are readily available and reasonably priced.
Based on these criteria, we chose the organic compounds listed in for further study. Calculated activation efficiency curves for these compounds are shown in . The values of D p50 are also given in the table. For those selected compounds the activation efficiency curves are generated by considering the effect of charge on the liquid droplet as it is predicted by the thermodynamic relation of Thomson (CitationSeto et al. 1997; CitationFriedlander 2000). The dielectric constant needed to apply the thermodynamic relationship of Thomson was found in CitationLide et al. (2007). Including the effect of charge on a liquid droplet diethylene glycol predicted the lowest value of D p50.
TABLE 1 Physical/chemical properties of the potential working fluids and parameters describing the performance of UCPC
The surface tensions of the glycols are about twice that of n-butanol. The vapor pressures of DOS and oleic acid at the peak supersaturation inside the condenser are about 2–4 orders of magnitude below that of n-butanol. These two categories of compounds are expected to tolerate relatively high supersaturations without undergoing significant homogeneous nucleation. Based on our literature reviews we concluded that these compounds should all be sufficiently nontoxic for use as CPC working fluids (CitationLove and Muir 1976; CitationEkwall et al. 1982; CitationZeiger et al. 1985; CitationScheuch and Stahlhofen 1987; CitationBrain et al. 1996; CitationMyojo and Sugimoto 1997; CitationHSDB 2007; CitationUS-EPA 2007).
Because vapor pressures of the working fluids that we chose are significantly below that of n-butanol, the droplet sizes following condensation will be smaller. Smaller droplets are more difficult to detect optically. Condensational growth of activated droplets was simulated along the axis starting from the peak of supersaturation. The enhanced evaporation by the Kelvin effect was neglected since evaporation rates are small relative to condensation rates once condensation begins. The change in the droplet temperature by the addition of latent heat and conduction through the surrounding gas were taken into account in the growth model. shows the calculated droplet size along the axis of the condenser.
The final droplet sizes for the glycols are about 1 μ m, which is roughly one order of magnitude smaller than droplets formed with n-butanol. CitationKim et al. (2003) showed that the diameter of grown droplets at the exit of an ethylene glycol based mixing type CPC could be all detected by a laser particle counter with a minimum detection size of 0.1 μ m; therefore, our calculated results are qualitatively consistent with their experimental observations. While particles in the 1 μ m diameter range can be detected by light scattering, they would be more difficult to detect than the 10 μ m droplets produced by butanol instruments. When oleic acid and DOS are used as working fluids, particles grow only to about 100 nm or less. Such small particles would be very difficult to detect optically. Therefore, in our experiments, a conventional butanol CPC (the “booster”) was added downstream of the UCPC to complete the growth of activated particles up to about 10 μ m.
It would be desirable to complete activation and growth within one laminar flow tube rather than adding another CPC downstream of the UCPC condenser, as was done in our research. One way to accomplish this would be to add a short section of heated porous tube wetted with H2O to cause further condensational growth within a short distance (CitationHering et al. 2005). Theoretical analysis of such a system is given in Appendix A.
EXPERIMENT
Experimental Setup
The activation efficiencies of the potential working fluids identified in our theoretical study were experimentally evaluated. shows the setup for generating the test aerosols. Test nanoparticles are generated by the evaporation/condensation technique (CitationScheibel and Porstendöerfer 1983) from sodium chloride, ammonium sulfate, and silver. Dry nitrogen sampled from the head space of liquid nitrogen at 77 K was used as the carrier gas to reduce the likelihood that particle composition would be affected by contaminants.
When sodium chloride crystals or silver are placed in the furnace nanoparticles of the same composition are expected to form. However, nanoparticles formed by heating ammonium sulfate crystals do not consist of ammonium sulfate. For the furnace temperatures used in this study (230–300°C) it is known that ammonium sulfate dissociates into solid products such as ammonium bisulfate and ammonium pyrosulfate and gas phase products that include ammonia, sulfur dioxide, water, and nitrogen (CitationHalstead 1970; CitationKiyoura and Urano 1970). Therefore, we are not certain about the composition of the nanoparticles produced from the ammonium sulfate.
The aerosol exiting from the furnace is diluted and subsequently enters the aerosol charger. Our aerosol charger (CitationChen and Pui 1999) can be used as either a unipolar or bipolar charger by turning on and off the voltage applied to the ion separation region. The charged particles are classified by the Nano-DMA (CitationChen et al. 1998), which was operated with a sheath flowrate of 15.0 LPM. The 1.5 LPM monodisperse aerosol outflow was controlled by the system downstream, as will be shown later. The excess flow at 1.9 LPM was vented through a filter from the bypass flow port of the Nano-DMA, and the flowrate from the exit of furnace to the inlet slit of the Nano-DMA classifier region was controlled by a dilution loop.
shows the schematic of the experimental setup used to evaluate the activation efficiency of the Ultrafine CPC.
FIG. 8 Experimental setup for evaluating the activation efficiency of the Ultrafine CPC that uses various working fluids.
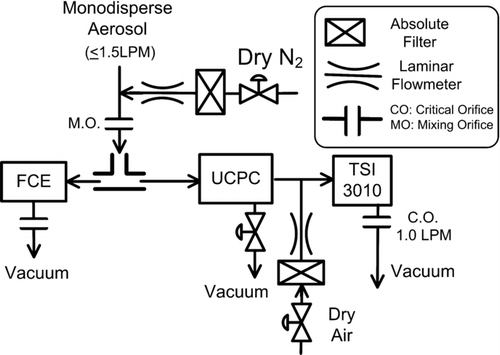
The monodisperse aerosol exiting from the Nano-DMA diluted by dry nitrogen is passed through a 2.2 mm diameter mixing orifice before being symmetrically divided into equal proportions that flow into the UCPC and Faraday cage electrometer (FCE). The Faraday cage is based on the design of CitationOkuyama et al. (1998). During this study the CPC and electrometer flowrates were varied from 1.5 to 1.9 LPM. The difference between the condenser and saturator temperature of the UCPC was maximized to the point where false counts due to homogeneous nucleation were negligible (1 count per ∼ 5 min). As theoretical analysis suggested, the final sizes at the condenser exit were too small to be detected by the UCPC optics; therefore, an additional CPC (TSI 3010) having a 50% cutoff size at 15 nm is used to complete the growth up to optically detectable sizes. The flow control scheme within the UCPC is same as described by CitationStolzenburg and McMurry (1991) and is shown schematically in . We found that a cellulose filter could be effectively wetted by capillary action of the working fluids, while the wick originally installed in UCPC could not. Therefore, we used a rectangular 14 cm by 14 cm sheet of a cellulose filter as the saturator wick. The use of liquid level controller of the UCPC was unnecessary since the selected working fluids did not evaporate noticeably over a period of several days.
FIG. 9 Flow schematic of the ultrafine condensation particle counter, UCPC, and downstream TSI 3010 CPC.
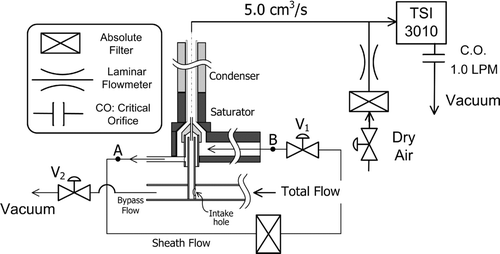
The core portion of the total flow is sampled at 5.0 cm3/s through the aerosol intake hole shown in . The major fraction of the sample flow is filtered and becomes the saturated sheath flow. The aerosol sample flow is introduced along the axis of the saturator extension through a small capillary. The dimensions of the capillary tube and the intake tube of the sample flow are found in CitationStolzenburg (1988). In this study, the capillary flow rate is varied from 0.6 to 0.75 cm3/s. The capillary flow rates were directly calibrated against the pressured drop across A and B, Δ P AB according to the procedure given in Chapter 5 of CitationStolzenburg (1988).
Experimental Results
Activation Efficiency
UCPC activation efficiencies were assumed equal to the ratio of the concentration of activated droplets at the UCPC condenser exit, which is calculated from the particle count rate measured by TSI-3010 and flowrate through UCPC capillary, to the concentration measured by the FCE after accounting for transport losses and biases in the classified sizes due to the finite width of the Nano-DMA transfer function. Appendix B describes the data inversion scheme in detail. The actual width of the Nano-DMA transfer function was evaluated semi-empirically by fitting the theoretical transfer function of CitationStolzenburg (1988) to the mobility spectrum of monodisperse fullerene (C60) monomer which is known to have a geometrical size around 1 nm (CitationSeol et al. 2002; CitationTanaka and Takeuchi 2002). The result showed that the peak mobility of the fitted transfer function was 1.1 cm2/V-s and the width of the transfer function was 1.07 times greater than the theoretical value evaluated at the mobility.
In this study the experimentally obtained 50% activation sizes for the working fluids investigated, D p50, are determined using two theories for the size–mobility relationship. Mobility diameter was calculated using the following relationship between size and electrical mobility in the free molecular regime (Fernandez de la Mora et al. 1998; CitationFriedlander 2000):
The parameters f 1 and f 2 are defined in Equations (26) and (27) of CitationTammet (1995), respectively. Note that f 2 in Equation (Equation4) differs from the expression given in Equation (27) of CitationTammet (1995) by the factor (1 + π α/8)− 1 to convert from continuum expression used by CitationTammet (1995) to the free molecular regime expression in Equation (Equation4). The factor m/f 1 2 equals the reduced mass of a particle having mass m p and a gas molecule. The factor f 2 accounts for two effects: the transition from elastic collisions in the hard sphere limit to inelastic collisions as particles increase from molecular to macroscopic dimensions, and charge-dipole interactions between the charged particle and the carrier gas. The collision diameter D pc equals the sum of the particle and gas collision diameters. The collision diameter of a particle consists of the geometrical diameter of the particle (mass diameter) plus an empirically evaluated “extra distance” due to other unaccounted intermolecular effects (∼ 0.230 nm according to Tammet). In this study the particle mass m p and mass diameter D pm are calculated from m p = ρ π D pm 3/6 where ρ is the bulk density of particle material (e.g., silver: 10.5 g/cm3, sodium chloride: 2.16 g/cm3, and ammonium sulfate: 1.77 g/cm3). Because the composition of particles produced from ammonium sulfate is not known, there is uncertainty in the latter density value.
shows values of D p50 for all the working fluids investigated in this study. The values in square and round brackets on the horizontal axis are the mobility diameter and the mass diameter, respectively. The table also shows the experimentally found saturator and condenser temperature where false counts due to homogeneous nucleation were negligible (1 count per ∼5 min). Using these temperatures we have recalculated the theoretically predicted peak saturation ratio inside the condenser and the value of D p50 predicted by the Kelvin relation.
TABLE 2 The 50% cutoff size of activation curve for the working fluids investigated
The peak saturation ratios, where the actual homogeneous nucleation rate becomes noticeable, are all lower than the values based on the calculations using the classical homogeneous nucleation theory. Additionally, theoretically predicted values of D p50 based on Kelvin diameter using the experimentally found temperature settings are much higher than any of the experimentally obtained values. Theoretically predicted values did not improve appreciably by considering the equilibrium between charged liquid droplet and vapor predicted by the thermodynamic relation of Thomson (CitationSeto et al. 1997; CitationFriedlander 2000) since saturation ratios are still too low to be in the range where the effect of charge has a significant effect on critical size. Other recent experimental studies have also shown that the saturation ratio required to activate sub-10 nm particles are well below the value predicted by the Kelvin relation, and the deviation from the Kelvin relation increases with decreasing size (CitationWinkler et al. 2006a; CitationWinkler et al. 2008).
Activation curves that we measured for diethylene glycol (DEG) and oleic acid (OA) are shown in and , respectively.
DEG and OA are, respectively, examples of high surface tension and low vapor pressure working fluids (see ). There are several important qualitative trends. First, negatively charged particles are more easily activated than positively charged particles. The polarity dependence of the activation efficiency of charged particles has been observed in previous experimental studies using an ethylene glycol based mixing type CPC (CitationKim et al. 2003) and a propanol based expansion type CPC (CitationWinkler et al. 2006b, Citation2007, Citation2008). The activated fractions at given sizes using oleic acid are generally lower than those obtained using diethylene glycol. This might be partly because the 50% cutpoint of the TSI 3010 (> 15 nm) is not low enough to count all of the particles downstream of the OA instrument. The condensational growth of the activated particles can be enhanced by higher concentrations of vapor inside the condenser, which can be done by increasing saturator temperature while maintaining the temperature difference between the saturator and condenser. It is also evident that the activation efficiencies depend on the material of the test particles. Previous theoretical studies indicate the activation efficiencies are strongly affected by the solubility of test particles in the working fluid and the wettability of particle surface by the working fluid vapor (CitationScheibel and Porstendöerfer 1986; CitationWagner et al. 2003; CitationKulmala et al. 2007).
FIG. 10 Measured activation efficiencies η of positively (left) and negatively charged (right) particles using diethylene glycol as working fluid and test aerosols generated from (a) silver, (b) ammonium sulfate, and (c) sodium chloride.
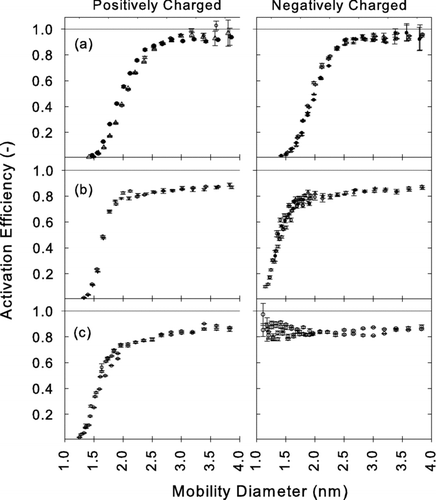
Qualitative trends in the performance of ethylene glycol and propylene glycol were similar to those for diethylene glycol (see ). Diethylene glycol has significantly lower D p50 than propylene glycol for detecting all three different particle materials, and diethylene glycol has significantly lower D p50 than ethylene glycol for detecting particles generated by evaporating silver. Values of D p50 of DOS for sodium chloride particles are not significantly different from silver indicating that the material dependence is slightly different between DOS and the other working fluids investigated. Conclusively, with diethylene glycol or oleic acid, particles having mobility diameter 2.0 nm (mass diameter 1.6 nm) can be detected independent of particle material. Depending on composition, particles having mobility diameter as small as 1.2 nm (mass diameter 0.8 nm) can be detected with these working fluids. If the sampled nanoparticles consist of semivolatile compounds that might evaporate before they enter the condenser, the working fluids which require lower saturator temperature (e.g., glycols) are preferable to those which require high saturator temperature (e.g., oleic acid and DOS).
It is noted that the obtained activation efficiency curves are potentially biased to lower values by the contribution of mobility classified ions penetrating into the Faraday cage electrometer (FCE) along with the mobility classified test particles. shows measured activation efficiencies η versus electrical mobility Z p of ethylene glycol that were obtained by using test aerosols generated from sodium chloride.
FIG. 11 Measured activation efficiencies η of positively charged (left) and negatively charged (right) particles using oleic acid as working fluid and test aerosols generated from (a) silver (b) ammonium sulfate and (c) sodium chloride.
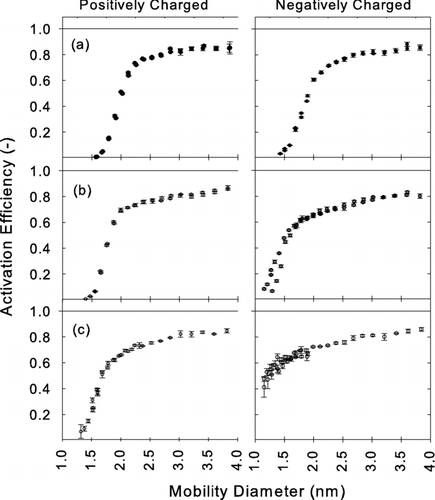
FIG. 12 Activation efficiencies η of ethylene glycol as a function of electrical mobility. The data were obtained by using test aerosols generated from sodium chloride. Mobility distributions of positive and negative ions generated from the aerosol charger are superimposed to illustrate the overlapping size range between ions and particles.
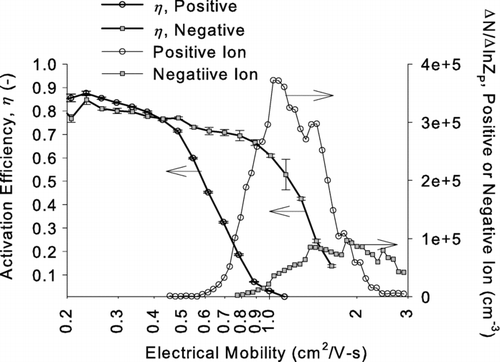
Mobility distributions Δ N/Δ ln Z p of positive and negative ion clusters generated from the aerosol charger are superimposed, and these measurements were taken by directly attaching the FCE at the Nano-DMA exit when no test particles were generated. As shown in the figure the mobility ranges of ion clusters overlap with the range where the activation efficiency of either positively or negatively charged particles start dropping off. Although not shown we have experimentally confirmed that our new CPC using glycol based compounds can activate only a few percent of incoming negative ion clusters and almost none of positive ion clusters, while the FCE is expected to detect 100% of these incoming ion clusters. Therefore, the concentration measured by the FCE in the overlapping mobility range is the sum of incoming charged particles and ion clusters classified within the same mobility window while the concentration measured by the CPC is almost all due to test particles. As a result, the activation efficiency, which is based on the ratio of CPC to FCE, may be partially biased toward lower values to an unknown degree in the size range of D p50. This effect is expected to be greater for positively charged particles since positive ion clusters have lower mobilities (and diffusivitities) than the negative ions; therefore, positive ion clusters penetrate more efficiently to the FCE, and could therefore partly account for the more rapid drop in activitation efficiencies that is observed for positive ions ( and ).
However, we believe that the observed sign dependence in the measured activation efficiencies is true since a strong sign dependence is observed in the mobility range where neither positive or negative ions are expected to be present when propylene glycol and silver are used as working fluid and test particles, respectively. A similar sign dependence of activation efficiencies was also observed in previous studies using a different technique (CitationWinkler et al. 2006b, Citation2007, Citation2008). In this technique the saturation ratio inside the expansion chamber is precisely controlled, and the light scattered off from the grown droplets within a viewing volume are measured (CitationWagner 1985; CitationWagner et al. 2003). The activated fraction is considered 100% when the light scattering signals reached a plateau; therefore, the method does not determine the activation efficiency absolutely by independently measuring the total concentration of particles within the viewing volume before and after the activation; however, the technique can precisely determine the saturation ratios where the activation of electrically charged and neutral particles occurs and is able to determine the sign dependence of activation more rigorously.
Performance Stability of Glycol Based CPC
One important application of the UCPC is to measure the concentration of sub 3 nm atmospheric particles. We carried out measurements to assess the stable performance of the prototype instrument. One source of instability is condensation of water in the condenser. This water can be absorbed by the working fluid, thereby affecting the saturation ratio within the condenser. Condensed water can also accumulate in the annular gap between the capillary and the wall of the saturator extension (see ). Microdroplets that form when this trapped water breaks up can contribute large noise counts. We investigated the stability of the glycol-based UCPC by monitoring the instrument activation efficiency over a period of three days when 2.3 nm (mobility size) silver particles were sampled at 40–45% relative humidity. Silver was used because it can easily be produced at stable concentrations over extended periods of time, and this size was chosen since it is near the 50% detection efficiency when the dry particles were introduced (See ); therefore, the instrument performance would likely be sensitive to changes in operating conditions. The water content of the UCPC sheath flow was controlled with a 56 cm long Nafion semipermeable membrane, an approach that can easily be used during atmospheric sampling without affecting the concentrations of sampled nanoparticles (CitationShi et al. 2007). Therefore, these measurements provide a realistic test of the instrument's stability during extended, unattended atmospheric sampling. Although stability tests were not performed using diethylene glycol as the working fluid, we would expect results similar to those found for propylene or ethylene glycol since the physical/chemical properties and performance of these three working fluids are similar. shows the activation efficiencies versus time for detecting 2.3 nm (a) positively charged silver particles using ethylene glycol and (b) negatively charged silver particles using propylene glycol as working fluid. During 2–3 days of testing the pressure reading across the capillary did not show any signs of water accumulation. As shown in the fraction of 2.3 nm silver particles detected by the UCPC remained steady over several days. Note that, for reasons that we do not yet understand, the variability in activation efficiency that is observed in appears to be associated with RH.
CONCLUSION
A laminar flow ultrafine condensation particle counter (UCPC) for detecting particles below 2 nm mobility diameter was developed by modifying the original prototype UCPC developed by CitationStolzenburg and McMurry (1991). Prior to experiments, theoretical analyses were performed using different working fluids to predict activation efficiencies and subsequent condensational growth. We chose to study five working fluids for which the predicted cutoff diameter, D p50, is below 2 nm. We selected working fluids that are non-hazardous and that have relatively low viscosities. However, due to the low vapor pressures, P v , of these working fluids in the condenser a second, conventional CPC is required to grow particles to a readily detectable size. Experiments were performed using test particles generated by evaporating sodium chloride, ammonium sulfate, and silver. To obtain the activation efficiency from the experimental data we have accounted for the size dependence of the input particle size distribution and particle penetration within the diffusion-broadened mobility window of particles selected by the DMA. Using diethylene glycol and oleic acid, values of the 50% activation efficiencies for negatively charged particles generated by evaporating sodium chloride, ammonium sulfate, and silver were found to be < 1.2 (< 0.8) nm, 1.4–1.5 (1.0–1.2), and 1.9–2.0 (1.5) nm as mobility (mass) diameter, respectively. Among the working fluids investigated diethylene glycol (DEG) is the recommended working fluid for atmospheric aerosol sampling because of the smaller sizes of detected particles and the relatively low saturator temperature. Also, because its vapor pressure is higher than that for some of the other working fluids, particles grew somewhat larger and were therefore easy to detect. The stability of the UCPC for long-term operation was tested by monitoring the instrument's response to 2.3 nm silver particles in an air at ∼ 40% RH using ethylene glycol and propylene glycol as working fluids, which reasonably simulates sampling conditions encountered for atmospheric aerosols. The performance was sufficiently steady (± 5% fluctuation) for several days, giving us confidence that this instrument will perform stably during unattended operation.
APPENDIX A: COMPLETING THE GROWTH OF ACTIVATED PARTICLES BY USING A HEATED POROUS TUBE WETTED WITH WATER
In our experiments, a butanol-based “booster” CPC was added downstream of the UCPC to grow particles to a size where they could easily be detected optically (∼ 10 μ m). However, it would be preferable to complete activation and growth within a single laminar flow tube. One way to accomplish this would be to add a short section of heated porous tube wetted with H2O to enhance the condensational growth (CitationHering et al. 2005). shows an example of the design. In this example, the condenser length and temperature are set to 10 cm and 5°C, respectively. The droplet size along the axis was simulated using the setup in as model input.
FIG. A1 Schematic of an approach for achieving nanoparticle activation and growth within a single laminar flow tube.
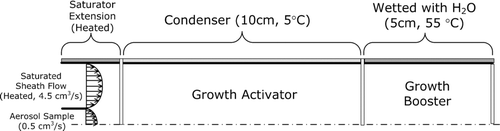
Composition of the particles growing within the growth booster changes from liquid droplets consisting mostly of the organic working fluid at the entrance to nearly pure water at the exit. The organic working fluid and the water condense simultaneously within the growth booster. Appling the Raoult's law the mole ratio of these two components within droplets were used to predict the vapor pressure of each component at the droplet surface. Calculations accounted for the temperature change caused by the latent heat of condensation and conduction through the surroundings. The calculations presented here only apply in the limit of low particle concentrations, where latent heat release and vapor depletion do not affect the supersaturation of working fluid vapor and growth of activated particles, respectively. shows the simulated results for all the activating fluids introduced in except for n-butanol. The growth curve for n-butanol is also given as a reference.
FIG. A2 The simulated droplet growth within growth activator and booster using the setup shown in as model input. The growth curve of n-butanol already shown in is also given as a reference.
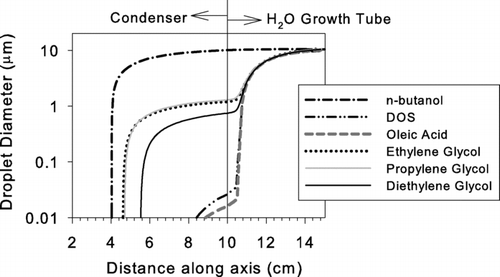
The growth due to the condensation of supersaturated water vapor initiates shortly after the aerosol enters the growth booster. Although the droplet sizes are initially different, depending on the activating working fluid, they are expected to asymptotically reach ∼ 10 μ m following the condensation of water. Although this single two-stage growth tube is theoretically feasible there is one design problem that would need to be overcome. It would be necessary to ensure that water condensing in the booster tube is kept separate from the organic working fluid, as this could affect saturation ratios that are achieved in first condensation stage.
APPENDIX B: DATA INVERSION
When sub 3 nm particles are classified by the Nano-DMA operating at 15 LPM sheath flow and 1.5 LPM aerosol flow, significant diffusional broadening in the width of DMA transfer function occurs. Therefore, it is not necessarily valid to assume that particles entering the detectors (UCPC and FCE) are monodisperse. In this section an inversion procedure used in our study to determine the size-dependent activation efficiency is described. The procedure accounts for the effect of the diffusional broadening in the width of DMA transfer function (CitationStolzenburg 1988; CitationStolzenburg and McMurry 2008), and the method is relatively straightforward and also yields reasonably accurate results.
and b show the portions of our experimental setup that are relevant for our data inversion procedure.
FIG. B1 Detailed schematics of the experimental setup for evaluating the activation efficiency of the Ultrafine CPC using (a) Nano-DMA as mobility classifier and the (b) UCPC and FCE as detectors
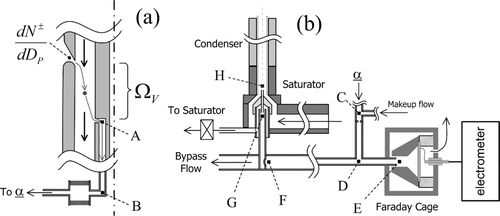
The inversion algorithm was originally developed by CitationStolzenburg (1988) and consists of two steps. The first step uses the least squares scheme to solve for the particle size distribution dN ±/ dD p of the positively or negatively charged particles at DMA inlet slit from the concentrations measured by FCE over a range of DMA voltages. The concentration measured by the FCE at a given classifying voltage V, N FCE | V , and the input particle size distribution, dN ±/ dD p , are theoretically related by
Only wall diffusion losses were considered to evaluate the size dependent transport efficiencies. summarizes the description of theoretically estimated diffusion losses through the transport segments in our experimental setup shown in .
TABLE B1 Description of theoretically accounted diffusion losses through transport within the experimental setup
The penetration through a straight segment of the tube were evaluated using the Gormley-Kennedy equation (CitationHinds 1999), and penetration through the annular section are approximated by the expression for the plane-parallel channel (CitationFuchs and Sutugin 1970). There are two sections where a core portion of sample flow is extracted along the axis of the flow; sample intake hole of UCPC and the inlet of the capillary tube. The penetration within the core portion of the flow was estimated by solving the Graetz problem, which solves the axial convection by fully developed flow and radial diffusion using the method of separation of variables. We assumed that flow instantaneously become fully developed after any 90-degree bends. This assumption is expected to underestimate the losses particularly at the flow segments D to F and F to G where losses within the core portion of the flow are calculated. While the losses through the core portion of the flow are minimal, higher losses are expected to occur as the flow mixes to some degree after 90-degree bends. It is emphasized that the activation efficiencies obtained by our inversion are lower than the true values overall since underestimating the losses through these two segments lowers the activation efficiency obtained by our data inversion technique.
The simplified scheme developed for this study avoids using Stolzenburg's least squares approach for evaluating dN ±/dD p in Equation (B1). Instead we assume that the aerosol reaching the FCE is monodisperse, which allows the removal of dN ±/dD p and P FCE from the integral leading to the following result.
In order to carry out the integral in Equation (B2), dN ±/dD p needs to be a continuous function of size over D p = 0 to ∞; accordingly, the value of N FCE appearing in Equation (B3) needs to be a continuous function of DMA voltage V which is a function of any size D p *. This is accomplished by either interpolating data among the voltages where the measurements were taken or extrapolating beyond the voltage range. compares the values of the particle size distribution of negatively charged particles dN −/dln D p obtained by formal inversion (Equation [B1]) and monodisperse approximation (Equation [B3]).
FIG. B2 Comparison of particle size distribution dN/dln D p of negatively charged particles at Nano-DMA inlet slit calculated by formal inversion (Equation [B1]) and by monodisperse approximation (Equation [B3]).
![FIG. B2 Comparison of particle size distribution dN/dln D p of negatively charged particles at Nano-DMA inlet slit calculated by formal inversion (Equation [B1]) and by monodisperse approximation (Equation [B3]).](/cms/asset/cf5322b7-0d0a-4344-8041-17e97f2eeb4a/uast_a_348987_o_f000b2g.gif)
The peak features of the particle size distribution obtained by the monodisperse approximation are less pronounced than these obtained by formal inversion due to the effect of finite width of DMA transfer function. The effect of finite width of the DMA transfer function on the activation efficiency is strong when the integrand in Equations (B1) and (B2) has a strong size dependence. So as to illustrate extreme errors in the monodisperse approximation made in this study, we chose a data set in which the obtained particle size distribution at the DMA inlet slit decreased sharply as mobility diameters dropped below 1.5 nm mobility diameter. shows the concentration reaching Faraday cage electrometer (FCE) calculated from theory (Equation [B1]) using particle size distributions dN −/dln D p obtained by formal inversion and monodisperse approximation.
FIG. B3 Concentration reaching Faraday cage electrometer (FCE) calculated from theoretical expression (Equation [B1]) using particles size distributions obtained by formal inversion and monodisperse approximation shown in . Experimentally measured concentrations are also given to show the goodness of fit.
![FIG. B3 Concentration reaching Faraday cage electrometer (FCE) calculated from theoretical expression (Equation [B1]) using particles size distributions obtained by formal inversion and monodisperse approximation shown in Figure B2. Experimentally measured concentrations are also given to show the goodness of fit.](/cms/asset/5e17129c-8b75-4e1e-bfc2-d088965f9e9a/uast_a_348987_o_f000b3g.gif)
Experimentally measured concentrations are also given to show the goodness of fit. The monodisperse approximation leads respectively to an underestimate and an overestimate of the theoretically calculated concentrations reaching the Faraday cage electrometer near the peaks and the valley between two peaks. As it will be shown later the overall effect on the obtained activation efficiency is small. The error in the input particle size distribution causes error in the value of theoretically calculated concentration reaching the CPC as well. As seen in Equation B2 the activation efficiency is the ratio of concentration reaching CPC to FCE; therefore, this error tends to cancel out.
The second step of the inversion is also simplified by neglecting the size dependence of activation efficiency η within the numerator of Equation (B2) to explicitly solve for η :
CitationMordas et al. (2008) developed a similar approach to account for the effect of the finite width of the DMA transfer function on the sizes of the DMA-classified particles.
In contrast, it is often assumed that efficiencies can be evaluated at the DMA centroid diameter. In this case the activation efficiency would be calculated using the following expression:
Note that Equation (B6) neglects the size dependence of dN ±/dD p , P CPC , P FCE , and η inside the integrals of Equation (B2), while Equation (B4) neglects the size dependence of η only. compares activation efficiencies with results obtained by formal inversion scheme of Stolzenburg with results obtained using Equation (B6) (the “monodisperse approximation”) and Equations (B4) and (B5) (the simplified inversion scheme).
FIG. B4 Comparison of activation efficiency η obtained by the monodisperse approximation (Equation [B6]), the formal inversion of Stolzenburg (Equations [B1] and [B2]), and the simplified approach introduced in this work (Equations [B4] and [B5]). The test particles are generated by evaporating the ammonium sulfate crystals.
![FIG. B4 Comparison of activation efficiency η obtained by the monodisperse approximation (Equation [B6]), the formal inversion of Stolzenburg (Equations [B1] and [B2]), and the simplified approach introduced in this work (Equations [B4] and [B5]). The test particles are generated by evaporating the ammonium sulfate crystals.](/cms/asset/fdab0d2a-8c48-4723-9ef0-dedfb62cfb4f/uast_a_348987_o_f000b4g.gif)
The values of D p50 obtained by the monodisperse approximation, the formal approach of CitationStolzenburg (1988), and the simplified scheme in this study are 1.20 nm, 1.27 nm, and 1.30 nm, respectively. Since the distribution function used for these measurements varies sharply with size this example should provide a reasonable estimate of the maximum error in D p50 caused by the monodisperse approximation in this study. The value of D p50 estimated by the simplified approach in this study agrees within 2.2% with that obtained using Stolzenburg's formal inversion indicating that the method yields sufficiently accurate results. All the experimental results in this study were processed by this approach.
Acknowledgments
This work was supported by NSF Award Number ATM-0506674. We greatly appreciate the insightful comments and suggestions of Prof. Fernandez de la Mora at Yale University and Prof. Okuyama at Hiroshima University, which significantly impacted this work.
Notes
a Chemical Abstracts Service, a division of the American Chemical Society.
b (CitationLide et al. 2007), abbreviations: s (soluble); sl (slightly soluble); i (insoluble); msc (miscible); vs (very soluble); ace (acetone); bz (benzene); chl (chloroform); ctc (carbon tetrachloride); eth (diethyl ether); EtOH (ethanol).
d Dielectric constants needed to apply the thermodynamic relationship of Thomson could not be found.
a One standard deviation among the different experiments.
b The lowest particle diameter tested is shown since the obtained activation efficiency curves are above 50% at the size.
REFERENCES
- Ankilov , A. , Baklanov , A. , Colhoun , M. , Enderle , K. , Gras , J. , Julanov , Y. , Kaller , D. , Lindner , A. , Lushnikov , A. , Mavliev , R. , McGovern , F. , Mirme , A. , O'Connor , T. C. , Podzimek , J. , Preining , O. , Reischl , G. P. , Rudolf , R. , Sem , G. J. , Szymanski , W. W. , Tamm , E. , Vrtala , A. E. , Wagner , P. E. , Winklmayr , W. and Zagaynov , V. 2002 . Particle Size Dependent Response of Aerosol Counters . Atmos. Res. , 62 : 209 – 237 .
- Bartz , H. , Fissan , H. , Helsper , C. , Kousaka , Y. , Okuyama , K. , Fukushima , N. , Keady , P. B. , Kerrigan , S. , Fruin , S. A. , McMurry , P. H. , Pui , D. Y. H. and Stolzenburg , M. R. 1985 . Response Characteristics for Four Different Condensation Nucleus Conters to Particles in the 3–50 nm Diameter Range . J. Aerosol Sci. , 16 : 443 – 456 .
- Brain , J. D. , Blanchard , J. D. , Heyder , J. , Wolfthal , S. F. and Beck , B. D. 1996 . Relative Toxicity of Di(2-ethylhexyl) Sebacate and Related Compounds in an In Vivo Hamster Bioassay . Inhal. Toxicol. , 8 : 579 – 593 .
- Chen , D.-R. and Pui , D. Y. H. 1999 . A High Efficiency, High Throughput Unipolar Aerosol Charger for Nanoparticles . J. Nanoparticle Res. , 1 : 115 – 126 .
- Chen , D.-R. , Pui , D. Y. H. , Hummes , D. , Fissan , H. , Quant , F. R. and Sem , G. J. 1998 . Design and Evaluation of a Nanometer Aerosol Differential Mobility Analyzer (Nano-DMA) . J. Aerosol Sci. , 29 : 497 – 509 .
- Ekwall , B. , Nordensten , C. and Albanus , L. 1982 . Toxicity of 29 Plasticizers to HeLa Cells in the MIT-24 System . Toxicol. , 24 : 199 – 210 .
- Fernandez de la Mora , J. , De Juan , L. , Eichler , T. and Rosell , J. 1998 . Differential Mobility Analysis of Molecular Ions and Nanometer Particles . Trends in Analytical Chemistry , 17 : 328 – 339 .
- Friedlander , S. K. 2000 . Smoke, Dust, and Haze: Fundamentals of Aerosol Dynamics , New York : Oxford University Press .
- Fuchs , N. A. and Sutugin , A. G. 1970 . Highly Dispersed Aerosols , Ann Arbor/London : Ann Arbor Science Publishers .
- Gamero-Castano , M. and Fernandez de la Mora , J. 2000 . A Condensation Nucleus Counter (CNC) Sensitive to Singly Charged Sub-Nanometer Particles . J. Aerosol Sci. , 31 : 757 – 772 .
- Gamero-Castano , M. and Fernandez de la Mora , J. 2002 . Ion-Induced Nucleation: Measurement of the Effect of Embryo's Size and Charge State on the Critical Supersaturation . J. Chem. Phys. , 117 : 3345 – 3353 .
- Halstead , W. D. 1970 . Thermal Decomposition of Ammonium Sulfate . J. Appl. Chem. , 20 : 129 – 132 .
- Hering , S. V. , Stolzenburg , M. R. , Quant , F. R. , Oberreit , D. R. and Keady , P. B. 2005 . A Laminar-Flow, Water-Based Condensation Particle Counter (WCPC) . Aerosol Sci. Technol. , 39 : 659 – 672 .
- Hienola , A. I. , Winkler , P. M. , Wagner , P. E. , Vehkamaki , H. , Lauri , A. , Napari , I. and Kulmala , M. 2007 . Estimation of Line Tension and Contact Angle From Heterogeneous Nucleation Experimental Data . J. Chem. Phys. , 126
- Hinds , W. C. 1999 . Aerosol Technology: Properties, Behavior, and Measurement of Airborne Particles , New York : Wiley .
- HSDB . 2007 . Hazardous Substances Data Bank: Deithylene Glycol, National Library of Medicine
- Kesten , J. , Reineking , A. and Porstendoerfer , J. 1991 . Calibration of a TSI model 3025 Ultrafine Condensation Particle Counter . Aerosol Sci. Technol. , 15 : 107 – 111 .
- Kim , C. S. , Okuyama , K. and Fernãndez de la Mora , J. 2003 . Performance Evaluation of an Improved Particle Size Magnifier (PSM) for Single Nanoparticle Detection . Aerosol Sci. Technol. , 37 : 1 – 13 .
- Kiyoura , R. and Urano , K. 1970 . Mechanism, Kinetics, and Equilibrium of Thermal Decomposition of Ammonium Sulfate . Industrial & Engineering Chemistry Process Design and Development , 9 : 489 – 494 .
- Knutson , E. O. and Whitby , K. T. 1976 . Aerosol Classification by Electric Mobility: Apparatus, Theory, and Applications . J. Aerosol Sci. , 6 : 443 – 451 .
- Kulmala , M. , Mordas , G. , Petäjä , T. , Grönholm , T. , Aalto , P. P. , Vehkamäki , H. , Hienola , A. I. , Herrmann , E. , Sipilä , M. , Riipinen , I. , Manninen , H. E. , Hämeri , K. , Stratmann , F. , Bilder , M. , Winkler , P. M. , Birmili , W. and Wagner , P. E. 2007 . The Condensation Particle Counter Battery (CPCB): A New Tool to Investigate the Activation Properties of Nanoparticles . J. Aerosol Sci. , 38 : 289 – 304 .
- Lide , D. R. , Baysinger , G. , Berger , L. I. , Goldbuerg , R. N. , Kehiaian , H. V. , Kuchitsu , K. , Rosenblatt , G. , Roth , D. L. and Zwillinger , D. eds. 2007 . Handbook of Chem. Phys. 88th ed. “A Ready-Reference Book of Chemical and Physical Data.” Boca Raton, FL
- Love , R. G. and Muir , D. C. 1976 . Aerosol Deposition and Airway Obstruction . Amer. Rev. Respir. Dis. , 114 : 891 – 897 .
- Magnusson , L.-E. , Koropchak , J. A. , Anisimov , M. P. , Poznjakovskiy , V. M. and Fernandez de la Mora , J. 2003 . Correlations For Vapor Nucleating Critical Embryo Parameters . J. Phs. Chem. Ref. Data , 32 : 1387 – 1410 .
- McMurry , P. H. 2000 . The History of Condensation Nucleus Counters . Aerosol Sci. Technol. , 33 : 297 – 322 .
- Mordas , G. , Sipilä , M. and Kulmala , M. 2008 . Nanometer Particle Detection by the Condensation Particle Counter UF-02proto . Aerosol Sci. Technol. , 45 : 521 – 527 .
- Myojo , T. and Sugimoto , M. 1997 . Comparative Study of Challenge Aerosols for Performance Test for Dust Respirators . Industrial Health , 35 : 502 – 507 .
- Okuyama , K. , Shimada , M. , Okita , A. , Otani , Y. and Cho , S. J. 1998 . Performance Evaluation of Cluster-DMA with Integrated Electrometer and its Application to Ion Mobility Measurements . Earozoru Kenkyu , 13 : 83 – 93 .
- Reid , R. C. , Prausnitz , J. M. and Poling , B. E. 1987 . The Properties of Gases and Liquids, 4th ed. , New York : McGraw-Hill .
- Scheibel , H. G. and Porstendöerfer , J. 1983 . Generation of Monodisperse Silver and Sodium Chloride Aerosols with Particle Diameters between 2 and 300 nm . J. Aerosol Sci. , 14 : 113 – 126 .
- Scheibel , H. G. and Porstendöerfer , J. 1986 . Counting Efficiency and Detection Limit of Condensation Nuclei Counters for Submicrometer Aerosols . J. Colloid Interface Sci. , 109 : 261 – 274 .
- Scheuch , G. and Stahlhofen , W. 1987 . Particle Deposition of Inhaled Aerosol Boluses in the Upper Human Airways . J. Aerosol Sci. , 18 : 725 – 727 .
- Seinfeld , J. H. and Pandis , S. N. 1998 . Atmos. Chem. Phys.: From Air Pollution to Climate Change , New York : Wiley .
- Seol , K. S. , Yabumoto , J. and Takeuchi , K. 2002 . A Differential Mobility Analyzer with Adjustable Column Length for Wide Particle-Size-Range Measurements . J. Aerosol Sci. , 33 : 1481 – 1492 .
- Seto , T. , Okuyama , K. , de Juan , L. and Fernãndez de la Mora , J. 1997 . Condensation of Supersaturated Vapors on Monovalent and Divalent Ions of Varing Size . J. Chem. Phys. , 107 : 1576 – 1585 .
- Shi , Q. , Sakurai , H. , H. and M. P. 2007 . Characteristics of Regional Nucleation Events in Urban East St. Louis . Atmos. Environ. , 41 : 4119 – 4127 .
- Stolzenburg , M. R. 1988 . “ An Ultrainfe Aerosol Size Distribution Measuring System ” . In Dept. of Mech. Eng. , Minneapolis, Minnesota, USA : Univ. Minnesota .
- Stolzenburg , M. R. and McMurry , P. H. 1991 . An Ultrafine Aerosol Condensation Nucleus Counter . Aerosol Sci. Technol. , 14 : 48 – 65 .
- Stolzenburg , M. R. and McMurry , P. H. 2008 . Equations Governing Single and Tandem DMA Configurations and a New Lognormal Approximation to the Transfer Function . Aerosol Sci. Technol. , 42 : 421 – 432 .
- Tammet , H. 1995 . Size and Mobility of Nanometer Particles, Clusters and Ions . J. Aerosol Sci. , 26 : 459 – 475 .
- Tanaka , H. and Takeuchi , K. 2002 . C60 Monomer as an Inherently Monodisperse Standard Nanoparticle in the 1 nm Range . Jpn. J. Appl. Phys. , 41 : 922 – 924 .
- Topas-GmbH . 2007 . Specification and MSDS Sheet for Di-Ethyl-Hexyl-Sebacat Available online at http://www.topas-gmbh.de/_DEHS_en.htm (accessed October 2008).
- US-EPA , I. 2007 . Integrated Risk Information System: Ethylene glycol , US-EPA .
- Wagner , P. E. 1985 . A Constant-Angle Mie Scattering Method (CAMS) for Investigation of Particle Formation Processes . J. Colloid and Interface Sci. , 105
- Wagner , P. E. , Kaller , D. , Vrtala , A. , Lauri , A. and Kulmala , M. 2003 . Nucleation Probability in Binary Heterogeneous Nucleation of Water-n-Propanol Vapor Mixtures on Insoluble and Soluble Nanoparticles . Physical Review E: Statistical, Nonlinear, and Soft Matter Physics , 67 ( 2–1 ) : 1 – 12 .
- Wang , S. C. and Flagan , R. C. 1990 . Scanning Electrical Mobility Spectrometer . Aerosol Sci. Technol. , 13 : 230 – 240 .
- Wiedensohler , A. , Orsini , D. , Covert , D. S. , Coffman , D. , Cantrell , W. , Havlicek , M. , Brechtel , F. J. , Russell , L. M. , Weber , R. J. , Gras , J. , Hudson , J. G. and Litchy , M. 1997 . Intercomparison Study of the Size-Dependent Counting Efficiency of 26 Condensation Particle Counter . Aerosol Sci. Technol. , 27 : 224
- Wilke , C. R. and Lee , C. Y. 1955 . Estimation of Diffusion Coefficients for Gases and Vapors . J. Industrial and Engineering Chemistry , 47 : 1253 – 1257 .
- Wilson , C. T. R. 1900 . On the Comparative Efficiency as Condensation Nuclei of Positively and Negatively Charged Ions . Phil. Trans. R. Soc. Lond. A , 193 : 289 – 308 .
- Winkler , P. M. , Steiner , G. W. , Reischl , G. P. , Vrtala , A. and Wagner , P. E. 2007 . “ The Effect of Seed Particle Charge on Heterogenenous Nucleation ” . In 17th International Conference on Nucleation and Atmospheric Aerosols , Edited by: O'Dowd , C. and Wagner , P. E. 358 – 362 . Ireland : Galway .
- Winkler , P. M. , Steiner , G. W. , Reischl , G. P. , Vrtala , A. , Wagner , P. E. , Gaman , A. , Vehkamaki , H. and Kulmala , M. 2006a . “ Observation of Nucleation of Organic Vapours by Nanoparticles Already at Particle Sizes Well Below the Kelvin Prediction ” . In 7th International Aerosol Conference , St. Paul. Minnesota, , USA : American Association for Aerosol Research .
- Winkler , P. M. , Steiner , G. W. , Reischl , G. P. , Vrtala , A. , Wagner , P. E. , Hienola , A. , Vehkamäki , H. and Kulmala , M. 2006b . Experimental Study of Heterogeneous Nucleation on Charged and Uncharged Nanoclusters, in Formation and Growth of Atmospheric Aerosols , 22 – 23 . Otsego, Minnesota, , USA : DOE-NSF-ACCENT-BACCI-iLEAPS-IGAC-SOLAS .
- Winkler , P. M. , Steiner , G. W. , Vrtala , A. , Vehkamäki , H. , Noppel , M. , Lehtinen , K. E. J. , Reischl , G. P. , Wagner , P. E. and Kulmala , M. 2008 . Heterogeneous Nucleation Experiments Bridging the Scale From Molecular Ion Clusters to Nanoparticles . Sci. , 319 : 1374 – 1377 .
- Yaws , C. L. 1999 . Chemical Properties Handbook: Physical, Thermodynamic, Environ., Transport, Safety, and Health Related Properties for Organic and Inorganic Chemicals , New York : McGraw-Hill .
- Zeiger , E. , Haworth , S. , Hortelmans , K. and Speck , W. 1985 . Mutagenicity Testing of di(2-ethylhexyl)phthalate and Related Chemicals in Salmonella . Environ. Mutagen. , 7 : 213 – 232 .