Abstract
Gas-to-particle conversion takes readily place in the atmosphere. Detecting the initial clusters, which act as embryos for the newly formed particles, is beyond traditional aerosol instrumentation. Charged atmospheric clusters can be measured with air ion spectrometers, but typical state-of-the-art condensation particle counters, which detect both neutral and charged clusters, only see particles larger than 2.5 nm in diameter. In this study we present a modified pulse-height condensation particle counter (PH-CPC) and confirm by laboratory verification that it is capable of detecting charged clusters with electrical mobility equivalent diameter down to ∼1 nm. We show how the detection efficiency and the pulse heights depend on the calibration particle size, polarity and composition. The effect of butanol supersaturation on the PH-CPC counting efficiency is also discussed. Furthermore, we developed an inversion method for the data to obtain true particle size distribution from the measurement signal.
1. INTRODUCTION
Atmospheric new particle formation from gaseous precursors by nucleation has been observed at numerous locations all over the world (CitationKulmala et al. 2004). Until recent years nucleation pathways have been poorly understood even though several different mechanisms have been suggested (CitationKulmala 2003; CitationKulmala et al.2006). Main reason for that has been the instrumental inability to detect particles below 3 nm in diameter. Direct measurements of both the nucleation process itself and the initial growth of the clusters are crucial in order to resolve the detailed pathways of the particle formation. Only very recently observations of atmospheric neutral particles and clusters below 3 nm have shed light on the first steps of particle formation (CitationKulmala et al. 2005, Citation2007a). Those observations were made using newly developed instruments designed for maximal detection efficiency of small clusters, like UF-02proto swirling flow condensation particle counter pair (CitationMordas et al. 2005; Citation2008a) and an Air Ion Spectrometer (CitationMirme et al. 2007) equipped with an aerosol charger.
Condensation particle counters (CPCs) are most common instruments used in sub-micron and nano-scale particle detection. Operation principles and main specifications of different types of CPCs have been reviewed by CitationMcMurry (2000). Cut-size is a parameter, which describes the particle detection efficiency of a CPC. The cut-size is defined as a mobility diameter of the smallest particles of which 50% are successfully counted. CitationMordas et al. (2008a) reported a cut-size of 1.8 nm for a UF-02proto CPC. For continuous flow TSI-3786 ultrafine water CPC a cut-size of 1.7 nm was reported by CitationMordas et al. (2008b) for water soluble NaCl particles. Very recently, CitationIida (2008) reported 50% activation efficiencies of 1.9 nm for silver and smaller than 1.3 nm for NaCl particles with a modified ultrafine CPC (TSI-3025). He used diethylene glygol or oleic acid vapor to activate the particles and magnify their size so that they were detectable with an ordinary butanol CPC. Very low cut-sizes are reported also for mixing type CPCs (CitationOkuyama et al. 1984; CitationSeto et al. 1997; CitationGamero-Gastaño and Fernández de la Mora 2002; CitationKim et al. 2003; CitationSgro and Fernández de la Mora 2004). For example the mixing type CPC—or “particle size magnifier”—of CitationSeto et al. (1997) was able to activate and grow singly charged ions down to approximately 1 nm in diameter. However, still no reports on the application of low cut-size mixing type CPCs to atmospheric charged or neutral cluster measurements have been published.
A difficulty related to the low cut-size CPCs is how to apply them to field measurements. In an ordinary sub-micron size distribution measurement system, like differential mobility particle sizer (DMPS), losses of 1–2 nm particles are so large and, at the same time, charging probabilities so low, that there is no use for the high efficiency CPC. Thus, the only reasonable option so far has been a parallel deployment of several CPCs with slightly different cut-sizes (e.g., CitationSchröder and Ström 1997; CitationBrock et al. 2000; CitationKulmala 2007a,Citationb). A disadvantage of this method is that when two large numbers are subtracted the uncertainty is typically very high. With low concentrations of sub-3 nm particles the signal is completely masked by slight fluctuations in the particle counting. Another difficulty arises, when the detection efficiency of the CPC is improved by increasing the supersaturation of the condensing vapor as eventually homogeneous nucleation starts (CitationHämeri et al. 1995), which leads to false counts in the optical detector.
In this paper, we show that a use of a pulse height condensation particle counter (CitationWiedensohler et al. 1994; CitationWeber et al. 1995; CitationSaros et al. 1996; CitationWeber et al. 1998; CitationDick et al. 2000) allows one to detect sub-3 nm particles and even atmospheric ion-, and neutral clusters. We show that supersaturated conditions above the onset of homogeneous nucleation can be used inside the PH-CPC's condenser and still be able to distinguish between droplets formed via homogeneous and heterogeneous nucleation. Furthermore, we show that this instrument can substitute a high cost battery of CPCs with different cut-sizes. We explored the performance of the PH-CPC in the particle size range of approximately 1–6 nm (mobility equivalent diameter), investigated the effect of total particle concentration on the detection efficiency, and studied the effect of particle polarity and composition. Utilization of a Herrmann type nano-DMA (CitationHermann et al. 2000) and mobility standards (CitationUde and Fernández de la Mora 2005) like tetrahepthyl ammonium bromide ions allowed us to perform extremely accurate calibration measurements, which are of great importance in the sub-3 nm size range. We also developed a simple data inversion method which performance was tested with simulations. The inversion was applied to field data to obtain the particle size distributions down to ∼1.5 nm in mobility diameter.
2. EXPERIMENT
2.1. The Pulse Height Condensation Particle Counter
PH-CPC used in this study comprises a TSI-3025A ultrafine CPC with modified optics and a multichannel analyzer (MCA) (CitationDick et al. 2000). The PH-CPC exploits an axial gradient of butanol supersaturation inside the CPC condenser (CitationStolzenburg and McMurry 1991). Particles entering the condenser are activated for growth at different axial positions depending on their initial size, which leads to a monotonic link between the original particle size and the final droplet size. Bigger particles activate earlier and since they have more time to grow, they reach larger droplet sizes, whereas smaller particles have to travel further inside the condenser before activation and therefore they yield smaller droplets in the end. For large particles the onset supersaturation is not a very strong function of particle diameter and therefore particles larger than ca. 15 nm activate practically at the same axial position (CitationSaros et al. 1996) thus yielding final droplets of approximately same size. Scattering cross-section for white light is a monotonic function of droplet size. Therefore measuring the intensity of the scattered light gives information about the initial particle size. In order to achieve the monotonic link between the scattering and the droplet size a monochromatic light source of 3025A is replaced by a white light source. This pulse height analysis method has been used for size distribution measurements between 3 and 10 nm (CitationWiedensohler et al. 1994; CitationWeber et al. 1995, Citation1998).
Actually the matter is a bit more complicated: The activation probability depends not only on the size, but also on the particle composition (CitationO'Dowd et al. 2002, Citation2003), and electrical charge (CitationWinkler et al. 2008). Furthermore, the activation probability depends on the total particle concentration activated inside the condenser due to the vapor consumption of the growing droplets. In addition to these factors, also the intensity of the light source affects the final measured pulse heights. In the current setup the light intensity is drifting slowly.
To maximize the activation probability of the smallest clusters, butanol supersaturation was increased by elevating the saturator temperature from 37°C up to 41–44°C and decreasing the condenser temperature by 0–2°C from a nominal 10°C set point. We utilized pulse height analysis to distinguish between butanol homogeneous self-nucleation and heterogeneous nucleation on cluster sized objects inside the condenser. To improve nanoparticle transport efficiency we increased the aerosol flow through the PH-CPC's capillary thus decreasing diffusion losses.
The experiments were carried out after service of the PH-CPC, which included cleaning the saturator and flow calibrations. At the same time, the aerosol flow (Qaer) through the capillary was increased from the nominal 0.5 cm3s−1 to 0.6 cm3s−1. In all experiments, the total sample flow (condenser flow) and the inlet flow were kept at nominal 5 cm3s−1, and 25 cm3s−1, respectively.
2.2. Aerosol Generation Methods
Experimental setup used in this study is presented in . Different methods were applied to generate calibration aerosol particles. For generation of mobility standards, e.g., monomer, dimer, and trimer of tetraheptyl ammonium bromide (THAB) an electrospray source was used. Tungsten oxide particles were produced with a Grimm 7860 WOx-generator and self-charged NiCr particles were generated with a hotwire generator (CitationPeineke et al. 2006). For silver and ammonium sulphate particles a tube furnace was used. WOx, silver and ammonium sulphate particles were charged with a radioactive Am-241 neutralizer before classification. Also cluster ions produced inside the same neutralizer were used for the detection efficiency studies.
The charged particles were classified using a high resolution Herrmann Differential Mobility Analyzer (HDMA, CitationHerrmann et al. 2000) operated in an open loop configuration with the sheath flow of approximately 500–1000 liters per minute (lpm) and the aerosol and the sample flow varying from 5 to 10 lpm. The maximum resolution of the DMA is ∼2% (full width at half maximum in mobility space at 1.5 nm). We performed the experiments with the resolution varying from 5 to 10%, because we wanted to eliminate any possible effects arising from significant pressure changes if the system was operated with the best resolution (i.e., the highest flow rates). The sheath air was filtered laboratory air. Filtration efficiency was, however, only approximately 90% and therefore typically few tens up to few hundreds of particles per cm3 were present in the sheath air and thus also in the aerosol sample air. These particles were clearly distinguishable in pulse height spectra and their existence did not affect the experiments. Depending on the demanded particle polarity the DMA was operated either with negative or positive high voltage in the center electrode.
The DMA was calibrated using THAB monomer, dimer and trimer as mobility standards. THAB was electrosprayed from an approximately 1mM methanol solution. Since mobilities of these ions are known (CitationUde and Férnandez de la Mora 2005), transformation of DMA voltage V to electrical mobility Z can be written as
Charged particles were detected with a TSI-3068B Faraday cage electrometer operated at the flow rate of 5 lpm. This instrument was used as a reference to the PH-CPC and they were operated in parallel.
In the experiments where the effect of the background particle population was investigated, a 109 mm Hauke DMA was used to extract 15 nm WOx particles. The concentration of the background particles was altered by adjusting furnace temperature of the WOx-generator.
2.3. Testing Protocols
The counting efficiency of the PH-CPC for THAB cations, negative and positive WOx particles, positively charged NiCr particles and charger generated ions of both polarities was investigated at different butanol supersaturations inside the condenser. Since both negative and positive particles were measured, the experiments provided insight into the effect of the particle charge on the detection efficiency. The experimental data were compared with calculated CPC transport losses. Also the detection efficiency response to differences in the composition of above mentioned calibration ions and particles was studied.
Because negative particles presumably activate more easily (CitationWinkler et al. 2008), most of the calibrations were performed with positively charged particles. Thereby the determined counting efficiencies should be more realistic when applied to the field measurement data, since most of the atmospheric sub-3 nm particles and clusters are expected to be neutral. Because of a lack of an accurate reference instrument for neutral sub-3 nm particles no calibrations with the neutral particles were performed.
Amount of homogenous nucleation inside the PH-CPC is strongly affected by the concentration of sampled particles, since they deplete the butanol vapor and reduce the supersaturation. This phenomenon is of importance during ambient measurements as the atmospheric concentrations of larger aerosol particles can vary several orders of magnitude. Thus the effect of background aerosol population on the homogeneous nucleation and on the detection efficiency for THAB-cations was explored. In these experiments, variable concentrations of 15 nm WOx particles were sampled along with the THAB-dimers.
Besides the detection efficiency, we also measured how the pulse heights relate to calibration particle sizes. Furthermore, we measured, using silver and ammonium sulphate particles, a set of PH-CPC transfer functions to be able to convert the PH spectra to true particle size distributions.
3. RESULTS AND DISCUSSION
3.1. Laboratory Experiments
We generated cluster ions by passing dry filtrated air through an Am-241 radioactive source and selected positive 1.3 nm (electrical mobility equivalent diameter) ions with the HDMA. The PH-CPC was operated with Tsat= 44°C, Tcond= 10°C, and Qaer= 0.6 cm3s−1. The obtained PH-spectra of this experiment are shown in . The leftmost mode (low pulse heights, small final droplets) is due to homogeneous nucleation and the rightmost mode (high pulse heights, large final droplets) is caused by the large (>10 nm) background particles resulting from imperfect sheath air filtration. Between these two modes, the pulses from the activated 1.3 nm ions show up (circles). When the ions were removed, only the homogeneous nucleation and the large particle activation occurred (solid line).
FIG. 2 An example of a measured pulse height spectrum, when PH-CPC is measuring with a high enough temperature difference to initiate homogeneous nucleation while detecting also large (>15 nm) particles (solid line). Homogeneously nucleated butanol droplets are seen in multichannel analyzer (MCA) channels 200–400 and aerosol particles in the MCA-channels 600–800. When calibration ions generated with a 60 MBq Am-241 source and sized with a Herrmann-DMA are directed to the PH-CPC, they show up at the MCA-channels 400–600 (circles).
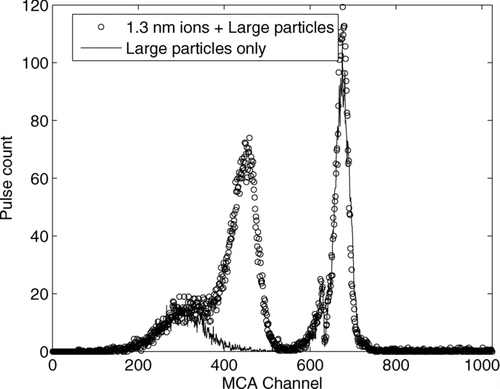
Disregarding the pulses due to the homogeneous nucleation and the large particles, the pulses N detected over a time interval t related to calibration ion activation can be extracted from the spectrum shown in . The number concentration C calculated from the pulse counts is then simply
summarizes the experimental results of the counting efficiency studies for different calibration ions and particles maintaining the condenser at a temperature of 10°C and varying the saturator temperature between 37°C and 44°C. At Tsat= 44°C even the smallest (∼1.2 nm) ions were detected with the counting efficiency of ∼5%. The efficiency increased rapidly with increasing particle size reaching 50% at ∼2.7 nm. Even at such a high supersaturation the 50%-counting efficiency, or cut-size, is only 0.3 nm better than the nominal cut-size of the 3025A CPC (3 nm). This was expected since at very high supersaturation the losses in aerosol transport line start to dominate the detection efficiency. The transport losses were calculated according to Stolzenburg and McMurry (1992) and they comprise the losses in the aerosol capillary, CPC's inner inlet and the 15 cm inlet tube from the inlet of the reference electrometer. Clearly not all of the smallest particles which theoretically can enter the condenser are activating. This is partly due to the fact that a fraction of smallest particles diffuse away from the activation region inside the condenser. The size of the effective activation region depends on the supersaturation and on the particle properties.
FIG. 3 The counting efficiency for positive charger generated ions and positive WOx –particles with a different saturator temperatures (Tsat) keeping the condenser temperature constant at 10°C. Capillary flow rate was 0.6 cm3s−1. At Tsat= 44°C and Tcond= 10°C even the smallest (∼1.2 nm) ions were detected with a counting efficiency of ∼5%. Transport efficiency was calculated according to Stolzenburg and McMurry (1992) and accounts for the losses in the aerosol capillary, CPC's inner inlet and 15 cm inlet tube.
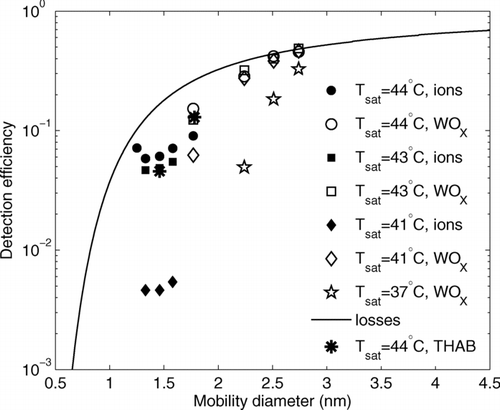
The counting efficiency for the smallest particles dropped drastically with decreasing Tsat (). Lowering the saturator temperature from 44°C to 41°C deteriorated the counting efficiency at 1.5 nm by a factor of 10. With the nominal saturator temperature Tsat= 37°C, no sub-2 nm particles were observed.
Growing droplets consume significant amounts of condensing vapor inside the condenser. This leads to a decrease in the supersaturation and consequently it reduces the homogeneous nucleation rate (). As the background aerosol number concentration increased from zero to 4000 cm−3, the homogeneously nucleated droplet concentration dropped from ∼200 to ∼20 cm−3. Larger background concentrations completely suppressed the homogeneous nucleation. As the background particles depleted the butanol vapor, they subsequently lowered the counting efficiency () in concert with the increasing background particle number concentration. Atmospheric particle concentrations can easily vary by 4–5 orders of magnitude from extremely polluted urban environments to pristine marine locations (e.g., CitationKulmala et al. 2005). This variation can cause measurement errors if it is not accounted for and in the worst case restrain reliable PH-CPC measurements altogether. Implementing an active control of the supersaturation to the PH-CPC or filtering out some of the background particles would improve the applicability of the PH-CPC.
FIG. 4 Concentration of homogeneously nucleated droplets (a), and the counting efficiency for THAB dimer (1.78 nm, b) as a function of background concentration of 15 nm WOx -particles
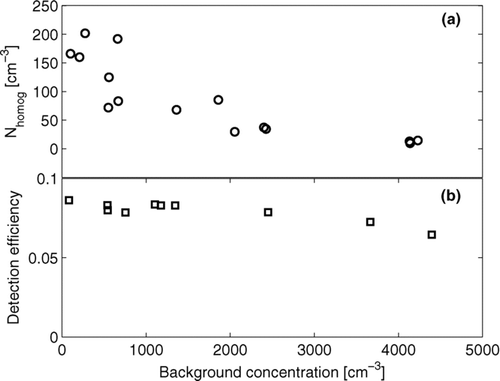
Other sources of error in the concentration measurement including unknown composition of the atmospheric clusters, and the role of the particle charge are apparently even larger than the effect of background concentration as can be estimated from . We investigated the PH-CPC detection efficiency for WOx particles and charger ions of both polarities and for positive NiCr particles. In these experiments as in the previous experiments we used Qaer= 0.6 cm3s−1 and Tcond= 10°C for aerosol sample flow rate and condenser temperature, respectively. But in order to decrease the homogeneous nucleation rate, the saturator temperature was lowered to Tsat= 43°C.
FIG. 5 Effect of charge polarity and particle composition on the counting efficiency. Negative WOx particles are counted more efficiently than positive ones. In the case of Am-241 charger generated ions, the opposite behavior is observed. The counting efficiency for the WOx particles is higher than for the NiCr particles and for the charger ions. Both the charge and the material dependency diminish towards larger particle sizes.
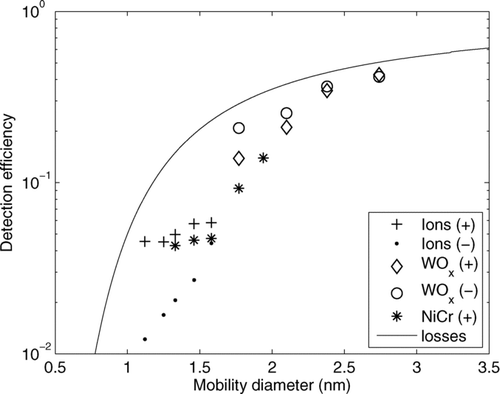
The counting efficiency of the PH-CPC has a clear sign preference. Negative WOx particles were detected more efficiently than the positive ones. The difference, however, rapidly diminished towards larger particle sizes. The opposite was observed for the radioactive charger generated ions. The positive ions were counted far more efficiently than the negative ions. This is most probably related to differences in the chemical composition of these ions, so that the effect of composition overcomes the effect of charge. However, since the nature and dynamics of ions produced inside the charger is not known and controlled in detail, no universal conclusions should be drawn. Because these ions are presumably composed of volatile impurities in the gas phase they can undergo changes in their composition and size also after classification thus affecting the results shown here. The effect of the particle composition can also be seen in the difference between the counting efficiencies for the positive WOx and the positive NiCr particles in favor of WOx. Even though the data are not overlapping, there seems to be a clear step in the detection efficiency between the WOx-particles and the charger generated ions, indicating that the butanol vapor preferably nucleates onto the WOx particles than on the charger ions.
The effect of particle charge has been investigated by CitationWinkler et al. (2008) who studied the activation probabilities of WOx particles in supersaturated propanol vapor. According to CitationWinkler et al. (2008) the negative particles were activated at lower supersaturations than the positive ones, which, in turn, were activated more easily than the neutral clusters of the same size. Importantly, also the neutral particles down to at least 1.7 nm were activated well before the onset supersaturation of homogeneous nucleation (CitationWinkler et al. 2008). This indicates that even though all our measurements are carried out with charged clusters, there is no physical reason why the neutral ones should not be activated inside the PH-CPC's condenser.
Measured relation between the mobility diameter of the calibration aerosol particles and the MCA peak channel is shown in . The MCA channel is shown in relative units, where 0 refers to the homogeneous nucleation peak channel and 1 corresponds to the peak channel where the large particles accumulate (). Relative units are used since the pulse heights, or the MCA channel numbers where the peaks are observed, depend on several factors including slowly drifting intensity of the white light source. One can immediately see from that there is no universal relation between the particle size and the relative MCA peak channel, but the relative channels depend on the supersaturation. At high supersaturation, the relative channels are larger than at lower supersaturations. The detection efficiency, when plotted as a function of relative MCA channel is on the contrary not strongly affected by the supersaturation (). This was expected, given that both pulse height and detection efficiency are related to final droplet size. Therefore, in field measurements it could be meaningful to estimate the detection efficiency from the MCA channel and determine the particle size more accurately, e.g., using a diffusion battery (CitationSipilä et al. 2008).
FIG. 6 Relation between the MCA peak channel and the particle diameter at different saturator temperatures (Tsat) for different positively charged calibration ions. Channel–diameter relation strongly depends on the supersaturation. Fitting to the data obtained with Tsat= 43°C is used in the data inversion.
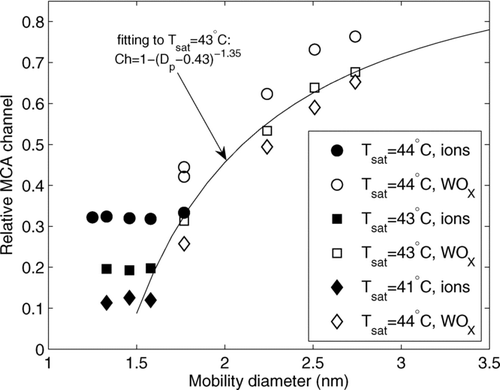
3.2. Data Inversion
For inverting the pulse height spectra to real size distributions, we measured the pulse height distributions from monodisperse silver particles of different sizes (referred here as a kernel function). Silver particles were produced in a tube furnace and size classified with a high resolution Herrmann DMA. Saturator temperature of 43°C and condenser temperature of 10°C were used. The relative width of the transfer function of the DMA is assumed to be negligible. shows the number of pulses divided by the concentration measured with the TSI electrometer as a function of relative channel for 9 different size classes from 1.57 to 5.66 nm. As particle size diminishes, the pulse distribution gets wider and the detection efficiency lower.
FIG. 8 Measured (solid lines) and modeled (dashed lines) kernel functions for monodisperse silver particles, and the modeled peak height (thick line). The concentrations are normalized by dividing with the particle concentration measured with the TSI electrometer. The measurements were done with the temperature settings Tsat= 43°C and Tcond= 10°C.
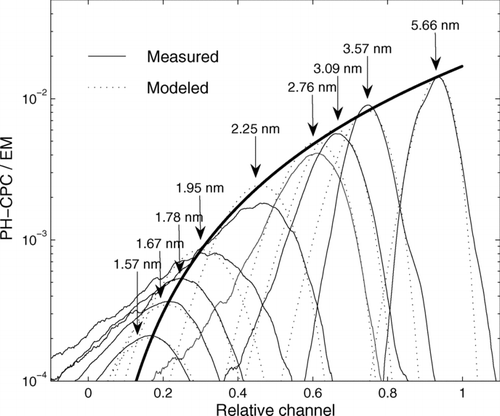
Particle size distribution N Dp is related to measured pulse height spectrum P ch by:
At Tsat= 43°C and Tcond= 10°C the relative channel number of the kernel function peak Ch is related to the particle mobility diameter Dp as presented by the fitting in :
also presents the modeled kernel functions as dashed lines and the modeled peak height as a thick black line. The modeled functions correspond to the measured ones fairly well, but the broadening towards lower channels is somewhat underestimated especially in the lower sizes. The effect of background aerosol concentration on the detection efficiency of clusters is not included.
Now the real number size distribution can be obtained by multiplying the measured smoothed pulse height distribution with the pseudoinverted kernel function matrix. The matrix was constructed by calculating 9 equally distributed kernel functions with 20 data points in each. The size of the matrix was chosen as a compromise between good size resolution and smoothness of the solution.
To attest the validity of the simplified inversion described above, we tested another method for inverting the PH-spectra, the extreme value estimation (EVE), which has been described in detail by CitationPaatero (1990) (see also CitationWiedensohler et al. 1994). This method allowed us to use measured kernel functions, which were determined using ammonium sulfate particles with mobility diameters from 1.5 nm up to 5.5 nm, only the noise was filtered. We used 36 kernel functions of which three examples are shown in . Solid line is the measured signal and squares show the filtrated kernel functions.
FIG. 9 Examples of the measured (solid lines) and filtered (squares) kernel functions for monodisperse ammonium sulfate particles.
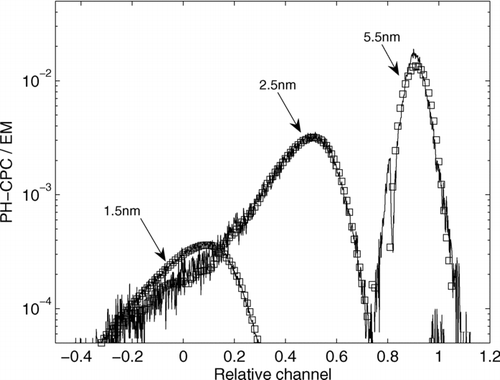
Both of the inversion methods were tested numerically by assuming three different kinds of multi-modal lognormal distributions that could be found in the atmosphere. First of them represents a situation where there is a distinguishable cluster mode at 2 nm and smaller modes at 6 nm and 50 nm. In the second and third case a growing nucleation mode appear, which acts as coagulation sink for the cluster mode particles. The distributions were converted into pulse counts and then random noise (up to ±5 pulses) was added before inverting the data with the modeled kernel functions using pseudoinversion method, or measured kernel functions using EVE method. In the inverted distributions are compared to the original ones. In case of measured transfer functions, central solution from EVE (CitationPaatero, 1990) has been plotted. The results agree considerably well between the size-range 1.5–6 nm, which validates the use of both inversion methods. However, the EVE method seems to underestimate the small particle (<2.5 nm) concentration slightly while overestimating the concentration of larger particles.
FIG. 10 The simulated lognormal size distributions (solid line) and the same distribution after converting it to MCA pulses, adding random noise and then inverting it back to real concentration using simulated kernel functions and pseudo inversion method (circles), or measured kernel functions and central solution from extreme value estimation (stars).
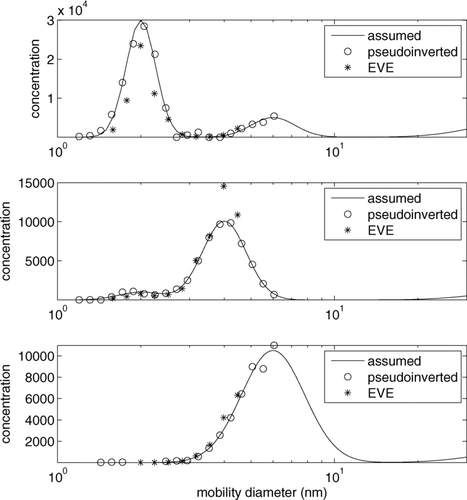
We tested the modeled transfer functions and pseudoinversion for the atmospheric data measured at the Hyytiälä field station in Southern Finland earlier published by CitationSipilä et al. (2008). They found out that clustering of the trace gases took place also outside nucleation events, even though those clusters did not reach the 3 nm detection limit of the basic aerosol instrumentation. Their estimation for the typical cluster concentrations ranged from some thousands up to few tens of thousands. shows the inverted pulse height spectra from 4th of April 2007. Night-time concentration of clusters prior the nucleation event varies around 1–2 · 103 particles cm−3. Boundary layer development dilutes both the larger particles and clusters after 7 a.m. On this chosen day a new particle formation event was taking place starting around 11 a.m., which can be seen as a sharp concentration increase in all sizes down to 1.5 nm. Throughout the day, also outside the nucleation event, there's a clearly distinguishable mode of sub-2 nm clusters with concentrations varying from less than a thousand up to few thousands. This result is in reasonable accordance with our earlier estimation (CitationSipilä et al. 2008) that on that day some thousands of clusters are needed to explain the observed pulse height spectra.
FIG. 11 Inverted PH-CPC data from Hyytiälä 4th April 2007. The upper panel shows the size distribution of particles between 1.2 and 5 nm. The lower panel shows total concentration of particles between smaller than 3 nm (black stars) and bigger than 3 nm (grey circles).
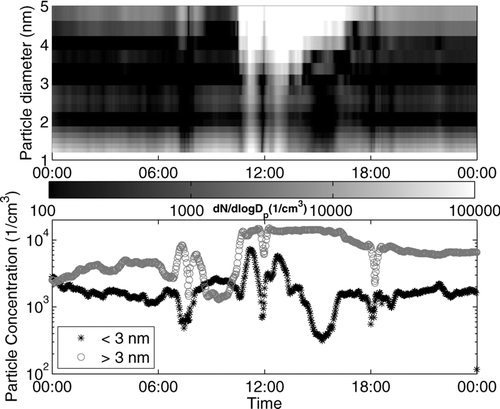
We were not able to apply measured kernel functions and EVE to field data. Reason for that is that our measured functions do not extend far enough to small sizes. Further calibration experiments with still smaller (<1.5 nm) particle sizes, preferably with neutral particles, are needed before the measured kernel functions can be used.
3.3. Limitations of the Method and Sources of Error in Field Studies
The reproducibility of the calibration experiments was good, but since we used atmospherically irrelevant materials and charged particles, the uncertainty in the detection efficiency, e.g., for 1.5 nm particles can exceed several percents (in absolute units) as can be seen in . Another source for error arises from the fluctuation of the pulse counts from homogeneous nucleation, in case the PH-CPC is operated in the homogeneous nucleation regime. With the saturator temperature of 44°C and the condenser temperature of 10°C the error estimation for 1.5–2 nm cluster concentration due to fluctuations is of the order of ±100 cm−3. Ambient concentrations of sub-3nm particles, e.g., during nucleation events usually should exceed several thousands in order to explain the observations of super-3nm concentrations (see, e.g., CitationKulmala et al. 2007a). Therefore error arising from the fluctuations can be considered acceptable.
The calibration measurements were performed with the background concentration of the order of ∼100 cm−3. Pulse heights are lowered due to increasing background concentration. As shown in the detection efficiency is dependent on the relative channel and therefore effect of increased background concentration on detection efficiency can be accounted for. However, also the relation between particle diameter and relative channel changes with changing background concentration. Thus the fact that the data, e.g., in the and are measured with low background concentration creates uncertainty mainly in the particle diameter, i.e., the inverted spectrum could be shifted a fraction of nanometer to smaller particle sizes with increasing total number concentration. We would, however, look askance the field data taken with the large particle number concentration exceeding several thousands, both because we have no experimental data with concentrations higher than ∼4000 cm−3, and because the coincidence starts to affect the measurement.
The kernel functions we used in the data inversion are fixed regarding to diameter. In section 3.1 we showed that the detection efficiency and relative channel are dependent on several factors including the saturator and the condenser temperatures, and the background particle concentration. To improve the accuracy of the inversion there should be a range of kernel function matrices for all possible ambient situations. Production of these matrices will require a vast amount of calibration experiments and that will be the topic of a future study.
4. CONCLUSIONS
In extensive and accurate laboratory examination the pulse-height condensation particle counter showed great potential for atmospheric nanocluster detection. The instrument was able to detect charged clusters down to molecular sizes. The counting efficiency was optimized by adjusting the saturator and the condenser temperatures, and the aerosol flow rate through the sampling capillary.
Background aerosol number concentration affected the detection efficiency of the PH-CPC. In order to increase the measurement accuracy, active control of the supersaturation should be implemented. The counting efficiency was affected by the polarity of the calibration particle charge as well as the particle composition. The negative WOx particles were detected with higher efficiency than the positive ones of similar size whereas an opposite behavior was observed for the charger generated ions. A clear effect of the particle composition was observed in favor of the WOx particles when compared to the charger ions or NiCr particles, whereas a not so clear difference was observed between THAB and the WOx particles. Since the chemical composition of atmospheric neutral or ion clusters is not known, the detection efficiencies determined using artificial nanoparticles cannot be directly applied to field data. The detection efficiency as a function of relative MCA channel was, however, observed to be reasonably constant and independent of the supersaturation. Thus, by using this relation for estimating the detection efficiency and, e.g., the diffusion battery, constructed and calibrated for nano-sized particles, for determination of particle size, reasonably reliable results on both the particle concentration and size could be obtained.
We developed a simple inversion for transforming the pulse height spectra to particle size distributions in the mobility diameter range ∼1.3–5 nm. Performance of the inversion was tested by simulations and by comparison to inversion using measured kernel functions and extreme value estimation (EVE) method. We also applied the inversion to the field data. The field results agree with the earlier findings (CitationKulmala et al. 2007a; CitationSipilä et al. 2008) that there seem to be constant presence of sub-3nm objects (clusters or very large molecules) in boreal forest environment. The pulse height analysis method was found to be a powerful tool for getting an insight in the first steps of atmospheric particle formation.
This work has been partially funded by European Commission 6th Framework programme project EUCAARI, contract no 036833-2 (EUCAARI). Maj and Tor Nessling foundation is acknowledged for financial support. Financial support from Academy of Finland is also acknowledged.
REFERENCES
- Brock , C. A. , Schröder , F. , Kärcher , B. , Petzold , A. , Busen , R. and Fiebig , M. 2000 . Ultrafine Particle Size Distributions Measured in Aircraft Exhaust Plumes . J. Geophys. Res. , 105 : 26555 – 26567 .
- Dick , W. D. , McMurry , P. H. , Weber , R. J. and Quant , R. 2000 . White-light Detection for Nanoparticle Sizing with the TSI Ultrafine Condensation Particle Counter . J. Nanoparticle Res. , 2 : 85 – 90 .
- Gamero-Gastaño , M. and Fernández de la Mora , J. 2002 . Ion-induced Nucleation: Measurements of the Effect of Embryo's Size and Charge State on the Critical Supersaturation . J. Chem. Phys. , 117 : 3345 – 3353 .
- Herrmann , W. , Eichler , T. , Bernardo , N. and Fernández de la Mora , J. 2000 . Turbulent Transition Arises at Reynolds Number 35,000 in a Short Vienna Type DMA with a Large Laminarization Inlet . Abstract AAAR Conference , 15B5
- Hämeri , K. , Augustin , J. , Kulmala , M. , Vesala , T. , Mäkelä , J. , Aalto , P. P. and Krissinel , E. 1995 . Evaluation of Homogeneous Droplet Formation inside UCPC (TSI model 3025) . J. Aerosol Sci. , 26 : 1003 – 1008 .
- Iida , K. 2008 . Atmospheric Nucleation: Development and Application of Nanoparticle Measurements to Assess the Roles of Ion-Induced and Neutral Processes , University of Minnesota: Ph.D. Thesis
- Kim , C. S. , Okuyama , K. and Fernández de la Mora , J.F. 2003 . Performance Evaluation of an Improved Particle Size Magnifier (PSM) for Single Nanoparticle Detection . Aerosol Sci. Technol. , 37 : 791 – 803 .
- Kulmala , M. 2003 . How Particles Nucleate and Grow? . Science , 302 : 1000 – 1001 .
- Kulmala , M. , Vehkamäki , H. , Petäjä , T. , Dal Maso , M. , Lauri , A. , Kerminen , V.-M. , Birmili , W. and McMurry , P. H. 2004 . Formation and Growth Rates of Ultrafine Atmospheric Particles: A Review of Observations . J. Aerosol Sci. , 35 : 143 – 176 .
- Kulmala , M. , Lehtinen , K. E. J. , Laakso , L. , Mordas , G. and Hämeri , K. 2005 . On the Existence of Neutral Atmospheric Clusters . Boreal Environ. Res. , 10 : 79 – 87 .
- Kulmala , M. , Petäjä , T. , Mönkkönen , P. , Koponen , I. K. , Dal Maso , M. , Aalto , P. P. , Lehtinen , K. E. J. and Kerminen , V.-M. 2005 . On the growth of nucleation mode particles: source rates of condensable vapour in polluted and clean environments . Atmos. Chem. Phys. , 5 : 409 – 416 .
- Kulmala , M. , Lehtinen , K. E. J. and Laaksonen , A. 2006 . Cluster Activation Theory as an Explanation of the Linear Dependence between Formation Rate of 3 nm Particles and Sulphuric Acid Concentration . Atmos. Chem. Phys. , 6 : 787 – 793 .
- Kulmala , M. , Riipinen , I. , Sipilä , M. , Manninen , H. , Petäjä , T. , Junninen , H. , Dal Maso , M. , Mordas , G. , Mirme , A. , Vana , M. , Hirsikko , A. , Laakso , L. , Harrison , R. M. , Hanson , I. , Leung , C. , Palmer , R. , Lehtinen , K. E. J. and Kerminen , V-M. 2007a . Towards Direct Measurement of Atmospheric Nucleation . Science , 318 : 89 – 92 .
- Kulmala , M. , Mordas , G. , Petäjä , T. , Grönholm , T. , Aalto , P. P. , Vehkamäki , H. , Hienola , A. I. , Herrmann , E. , Sipilä , M. , Riipinen , I. , Manninen , H. E. , Hämeri , K. , Stratmann , F. , Bilde , M. , Winkler , P. M. , Birmile , W. and Wagner , P. E. 2007b . The Condensation Particle Counter Battery (CPCB): A New Tool to Investigate the Activation Properties of Nanoparticles . J. Aerosol Sci. , 38 : 289 – 304 .
- McMurry , P. H. 2000 . The History of Condensation Particle Counters . Aerosol Sci. Technol. , 33 : 297 – 322 .
- Mirme , A. , Tamm , E. , Mordas , G. , Vana , M. , Uin , J. , Mirme , S. , Bernotas , T. , Laakso , L. , Hirsikko , A. and Kulmala , M. 2007 . A Wide-range Multi-channel Air ion Spectrometer . Boreal Environ. Res. , 12 : 247 – 264 .
- Mordas , G. , Kulmala , M. , Petäjä , T. , Aalto , P. P. , Matulevicius , V. , Grigoraitis , V. , Ulevicius , V. , Grauslys , V. , Ukkonen , A. and Hämeri , K. 2005 . Design and Performance Characteristics of a Condensation Particle Counter UF-02proto . Boreal Environ. Res. , 10 : 543 – 552 .
- Mordas , G. , Sipilä , M. and Kulmala , M. 2008a . Nanometer Particle Detection by the Condensation Particle Counter UF-02 proto. Accepted for publication in . Aerosol Sci. Technol. ,
- Mordas , G. , Manninen , H. , Petäjä , T. , Aalto , P. P. , Hämeri , K. and Kulmala , M. 2008b . On Operation of the Ultra-fine Water-based CPC TSI 3786 and Comparison with Other TSI Models (TSI 3776, TSI 3772, TSI 3025, TSI 3010, TSI 3007) . Aerosol Sci. Technol. , 42 : 152 – 158 .
- Mordas , G. , Sipilä , M. and Kulmala , M. 2008 . Nanometer Particle Detection by the Condensation Particle Counter UF-02 proto . Aerosol Sci. Technol. , 42 : 521 – 527 .
- O'Dowd , C. D. , Aalto , P. , Hämeri , K. , Kulmala , M. and Hoffmann , T. 2002 . Atmospheric Particles from Organic Vapours . Nature , 416 : 497 – 498 .
- O'Dowd , C. D. , Aalto , P. P. , Yoon , Y. J. and Hämeri , K. 2003 . The Use of the Pulse Height Analyzer Ultrafine Condensation Particle Counter (PHA-UCPC) Technique Applied to Sizing of Nucleation Mode Particles of Differing Chemical Composition . J. Aerosol. Sci. , doi:10.1016/j.jaerosci.2003.08.003
- Okuyama , K. , Kousaka , Y. and Motouchi , T. 1984 . Condensational Growth of Ultrafine Aerosol Particles in New Aerosol Size Magnifier . Aerosol Sci. Technol. , 3 : 353 – 366 .
- Paatero , P. 1990 . The Extreme Value Estimation Deconvolution Method with Applications in Aerosol Science . Univ. of Helsinki, Report Series in Physics , 250 : 1 – 41 . HU-P-
- Peineke , C. , Attoui , M. B. and Schmidt-Ott , A. 2006 . Using a Glowing wire Generator for Production of Charged, Uniformly Sized Nanoparticles at High Concentrations . J. Aerosol Sci. , 37 : 1652 – 1661 .
- Seto , T. , Okyama , K. , de Juan , L. and Fernández de la Mora , J. 1997 . Condensation of Supersaturated Vapors on Monovalent and Divalent Ions of Varying Size . J. Chem. Phys. , 107 : 1576 – 1585 .
- Saros , M. , Weber , R. J. , Marti , J. and McMurry , P. H. 1996 . Ultra Fine Aerosol Measurement Using a Condensation Nucleus Counter with Pulse Height Analysis . Aerosol Sci. Technol. , 25 : 200 – 213 .
- Sgro , L. A. and Fernández de la Mora , J. 2004 . A Simple Turbulent Mixing CNC for Charged Particle Detection Down to 1.2 nm . Aerosol Sci. Technol. , 38 : 1 – 11 .
- Sipilä , M. , Lehtipalo , K. , Kulmala , M. , Petäjä , T. , Junninen , H. , Aalto , P. P. , Manninen , H. E. , Vartiainen , E. , Riipinen , I. , Kyrö , E.-M. , Curtius , J. , Kürten , A. , Borrmann , S. and O'Dowd , C. D. 2008 . Applicability of Condensation Particle Counters to Measure Atmospheric Clusters . Atmos. Chem. Phys. , 8 : 4049 – 4060 .
- Stoltzenburg , M. R. and McMurry , P. H. 1991 . An Ultrafine Aerosol Condensation Nucleus Counter . Aerosol Sci. Technol. , 14 : 48 – 65 .
- Schröder , F. and Ström , J. 1997 . Aircraft Measurements of Sub Micrometer Aerosol Particles (>7 nm) in the Midlatitude Free Toposphere and Tropopause Region . Atmos. Res. , 44 : 333 – 356 .
- Ude , S. and Fernández de la Mora , J. 2005 . Molecular Monodisperse Mobility and Mass Standards from Electrosprays of Tetra-alkyl Ammonium Halides . J. Aerosol Sci. , 36 : 1224 – 1237 .
- Weber , R. J. , McMurry , P. H. , Eisele , F. L. and Tanner , D. J. 1995 . Measurement of Expected Nucleation Precursor Species and 3-500-nm Diameter Particles at Mauna Loa Observatory, Hawaii . J. Atmos. Sci. , 52 : 2242 – 2257 .
- Weber , R. J. , Stolzenburg , M. R. , Pandis , S. N. and McMurry , P. H. 1998 . Inversion of Ultrafine Condensation Nucleus Counter Pulse Height Distributions to Obtain Nanoparticle (∼3–10 nm) Size Distributions . J. Aerosol Sci. , 29 : 601 – 615 .
- Wiedensohler , A. , Aalto , P. , Covert , D. , Heintzenberg , J. and McMurry , P. H. 1994 . Intercomparison of Four Methods to Determine Size Distributions of Low-Concentration (∼100 cm−3), Ultrafine Aerosols (3 < Dp< 10 nm) with Illustrative Data from the Arctic . Aerosol Sci. Technol. , 21 : 95 – 109 .
- Winkler , P. M. , Steiner , G. , Vrtala , A. , Vehkamäki , H. , Noppel , M. , Lehtinen , K. E. J. , Reischl , G. P. , Wagner , P. E. and Kulmala , M. 2008 . Heterogeneous Nucleation Experiments Bridging Scale from Molecular ion Clusters to Nanoparticles . Science , 319 : 1374 – 1377 .