Abstract
The Los Angeles Ports complex consists of the port of Long Beach and the port of Los Angeles. Due to the high levels of particulate matter (PM) emitted from many sources in the vicinity of these ports and to their projected massive expansion, the Harbor area will be the focus of future governmental regulations. This study aims to characterize the physicochemical properties of PM at locations influenced by port-affiliated sources. PM samples were collected concurrently at six sites in the southern Los Angeles basin for a 7 week period between March and May 2007. Four sites were set-up within the communities of Wilmington and Long Beach; one site was located at a background location near the harbors of the Los Angeles port; the sixth site, near downtown Los Angeles, was chosen to represent a typical urban area. Coarse (PM 2.5 − 10 ), accumulation (PM 0.25 − 2.5 ), and quasi-ultrafine (PM 0.25 ) mode particles were collected at each site. Samples were analyzed for organic and elemental carbon content (OC and EC, respectively), organic species, inorganic ions, water soluble and total elements. The carbon preference index (CPI) for quasi-UF and accumulation mode particles varied from 0.65 to 1.84 among sites, which is in the range of previous findings in areas with high influence of anthropogenic sources. The ratio of hopanes to EC and hopanes to OC over all the sites were in the range of previous roadside measurements near freeways with variable volumes of diesel truck traffic. High overall correlation of vanadium with nickel (R = 0.9) and a considerable gradient of vanadium centrations with distance to the port, suggest marine vessels as the major sources of these elements.
INTRODUCTION
Epidemiological studies have shown significant exposure-response relationships for the adverse health effects in association with particulate mass concentrations (CitationSamoli et al. 2005; CitationAtkinson et al. 2001). Fine particles (aerodynamic diameter, Dp < 2.5 μ m; PM2.5) have been more strongly associated with mortality and morbidity than coarse particles (2.5 μ m < Dp < 10 μ m; PM2.5–10) (CitationWHO 2003) whereas coarse particles have been associated more strongly with respiratory hospital admissions (CitationBrunekreef and Forsberg 2005). Ultrafine particles (Dp < 0.1 μ m; PM0.1) are of particular interest in health-related studies due to their high number concentration in urban environments and ability to penetrate deep into the alveolar region of the lung (CitationDelfino et al. 2005). Recent toxicological studies suggest that adverse responses per unit mass are associated more strongly with ultrafine particles than fine or coarse particles (CitationDonaldson et al. 2002; CitationLi et al. 2003; CitationOberdörster et al. 2001; CitationXia et al. 2004).
Along with the particle size, the chemical composition influences the toxicity of particulate matter (CitationOberdörster 1996; CitationAnderson 2000). There are several types of highly toxic organic compounds in the atmosphere including quinones, polycyclic aromatic hydrocarbons (PAHs), polychlorinated biphenyls, and other organochlorine compounds, which may have acute effects (USEPA 2004; CitationLi et al. 2003). Pollen spores and proteins (in the super micron fraction) are known allergens, whereas some components of bacteria and viruses are biologically generated toxins (CitationLighty et al. 2000). Carbonaceous matter and transition metals such as iron, copper, chromium, and vanadium have been shown to generate reactive oxygen species and can contribute to oxidative DNA damage (CitationDreher et al. 1997). Secondary inorganic ions and sea salt as well as soil-related material are thought to be relatively benign, but they may affect the toxicity or bioavailability of other particulate components. In addition, endotoxins (mostly in coarse PM) are believed to play an important role in the development of organic-dust related diseases (e.g., CitationSalonen et al. 2004). Because the atmospheric aerosols form a highly multi-component system, it is very difficult to discern a clear association between adverse health effect and specific chemical components. If health effects can be linked to certain sources of particulate matter, such information would be highly valuable for targeting control strategies.
The Los Angeles Basin is a megalopolis of about 15 million inhabitants, and has one of the most polluted atmospheres in the United States due to the contributions of a multitude of traffic and other combustion sources. Studies in Los Angeles Basin examining atmospheric aerosols at multiple locations across the basin have been conducted since the early 1970s (CitationCass et al. 2000; CitationChristoforou et al. 2000; CitationHughes et al. 1999; CitationRussell and Cass 1986). Most of these campaigns in Los Angeles have included only a few days or a week or two of sampling. Recently, a more comprehensive, longer term campaign was operated by the Southern California Particle Center and Supersite (SCPCS), and the results are summarized by CitationSardar et al. (2005a). The focus of that campaign was to determine the size-fractionated and chemically speciated PM concentrations in the so-called “source” and “receptor” regions of the Los Angeles basin. The former are the central and western parts of the basin. In these regions, aerosols are mainly produced by primary emissions. By contrast, aged aerosols transported from the “source” regions dominate the eastern part of the basin, i.e., the “receptor” areas. PM samples in that campaign were not collected concurrently at the different sites, but were often apart by several years. To a certain degree, the consistent meteorology in Southern California may allow for seasonal comparisons between sites even if samples have been collected in different years. However, concurrent sampling in such a complex urban air basin would be highly desirable.
The focus of the present study is the area of the Los Angeles–Long Beach harbor. This is a unique area and quite disparate from the overall description of source and receptor sites of the basin that were discussed in the previous section. The Los Angeles port complex consisting of the port of Long Beach and port of Los Angeles is the busiest harbor in the United States and ranks fifth in the world. In addition to the harbor activities (e.g., Marine vessels, heavy-duty trucks, locomotives, cargo handling equipments and harbor crafts), the local PM sources include some of the most heavily traveled freeways in southern California (CA-110, I710 plus local street traffic) and multiple petroleum refineries and other industrial facilities. Many smaller industrial and commercial businesses are also located within the community. The Alameda Corridor runs through the eastern portion of the community. Thus, the surrounding area of the Los Angeles–Long Beach harbor constitutes arguably the most complex emission source scenario in California, and provides the potential for complex pollutant concentration gradients and high exposure conditions that cannot be identified by conventional monitoring approaches. Accordingly, it is crucial to assess the exposure gradient of the community in the surrounding environment. Nonetheless, there are not many studies on the micro-environmental spatial variations of chemical components and physical characteristics of particles in such complex environments.
The objective of this study is to characterize the chemical composition of ultrafine, accumulation mode and coarse particles across this community. Results from the gravimetric and chemical analysis are verified by means of chemical mass closure (CMC) (CitationSillanpää et al. 2006). Subsequently, the article focuses on organic species and elemental components and their distribution in PM size fractions among the sites. These results provide new insight into the variation of size-segregated chemical composition of PM over the studied area.
EXPERIMENTAL METHODS
Sampling Sites
Size-segregated PM samples were collected concurrently at five sampling sites in the LA port area and one additional site further north (at USC), serving as a representative site of the urban Los Angeles air quality, sufficiently far from the harbor, thus not immediately impacted by the local sources of that area. Sampling was conducted daily from Monday to Friday over a 7 week period from March to May of 2007. The sampling site locations are shown in and their specifications and major potential emission sources are described in detail in subsequent paragraphs.
Site 1 and 2 were located in the communities of Wilmington to the west of Long Beach area. Site 1 was about 1.5 km east (mostly downwind) of a major freeway (CA-110) and about 1.5 km north of Pacific Ocean coast. The site was situated in an open field, at the intersection of a major street and a local residential street.
Site 2 was about 3 km north of the coast. It was located in the backyard of a single-family house in a residential area, at the intersection of two busy major streets. It was also next to the Alameda corridor, a 20 mile (32 km) freight rail “expressway,” directly connecting the national rail system near downtown Los Angeles, to the ports of Los Angeles and Long Beach, running parallel to Alameda Street.
Site 3 and 4 were located within the communities of Long Beach. Site 3 was about 3 km north of the coast with a major highway (CA-1) in less than 1 km to the south (hence upwind) of the site. The sampling site was located inside a semi-industrial area with one and two-story buildings around it.
Site 4 was about 7 km north of the coast and 1 km downwind (east) of a major freeway (I-710), which has the highest ratio (up to 25%) of heavy-duty diesel vehicles in the Los Angeles highway network (CitationNtziachristos et al. 2007a). Another major freeway (I-405) was located about 1 km to the south of this site. The samplers were placed on the rooftop of a one-story building right beside a major street. Industrial, commercial and residential one- and two-story buildings were surrounding the site. This site was thus influenced by an urban and industrial mix of sources.
Site 5 was located in Long Beach at a pier extending about 1.5 km toward Pacific Ocean upwind of Long Beach harbor. The sampling site was located in an open area at the end of the pier, which was surrounded by ocean at three sides, and a small tree-planted area on one side. This site served as the background area of Long Beach harbor. The maximum distance between Sites 1 to 5 was about 8 km.
Site 6 was located on the University of Southern California campus at the Southern California Supersite Particle Instrumentation Unit (PIU) trailer near downtown Los Angeles. The PIU is about 40 km north of the coast, 150 m east of a major freeway (CA-110) and adjacent to a six level parking structure and various construction sites. This site represents a typical urban mix of downtown Los Angeles (CitationArhami et al. 2006) and was used for comparison to the harbor sites 1–5.
Sites 1–5 were located between latitudes of N33′ 44′ to N33′ 48′ and between longitudes of W118′ 13′ to W118′ 15′ and Site 6 were located at N34′ 1′ and W118′ 17′.
Sampling Description
Two collocated Sioutas™ impactors (SKC Inc., Eighty-Four, PA), operated at a flow rate of 9 lpm (CitationMisra et al. 2002; CitationSingh et al. 2003), were implemented at each site to collect size fractionated PM samples. Zefluor filters (3 μ m pore, Pall Life Sciences, Ann Arbor MI) were used in one impactor and quartz fiber filters (Pall Life Sciences, Ann Arbor MI) in the other impactor. Coarse (aerodynamic diameter, D P ≥ 2.5 μ m; PM2.5–10), accumulation mode (Dp = 0.25–2.5 μ m; PM0.25–2.5) and quasi-ultrafine (Dp < 0.25 μ m; PM0.25) particles were collected at each site. The impactors were placed inside temperature-controlled enclosures. The sampling inlet for each impactor was about 3 m in length (above the enclosure) and 0.95 cm in diameter and the vertical height of the inlet point from ground was at least 5 m. All sampling stations were mounted to the ground, except for Site 4, where the sampler enclosure was placed on the rooftop of a one-story building. Due to technical and weather-related problems, samples were not collected or were disregarded for week 7 at Sites 2, 4, and 5.
Local weather data, including temperature, relative humidity, wind speed and direction, were measured every minute at all sampling sites using a weather station (Vantage Pro2, Davis Instruments Corp., Hayward, CA). The meteorological data were not available for the weeks 1–5 at Site 1.
Prior to sampling, quartz filters were baked at 550°C for a minimum of 12 h and Zefluor filters were cleaned using sequential flow through leaches of 2N HCl, 2N HNO3 and high purity water. After cleaning the filters were air dried in a laminar flow hood in a trace metals clean room. At the end of each sampling period, all the filters and substrates were placed in petri dishes and quartz fiber filters were wrapped with aluminum foil. All the samples were kept frozen until chemical analysis.
Gravimetric and Chemical Analysis
Aerosol mass was determined by weighing the Zefluor filters before and after sampling with a microbalance (MT 5, Mettler-Toledo Inc., Highstown, NJ) having a sensitivity of 0.001 mg. The samples were allowed for equilibration in the weighing room at a relative humidity of 40–45% and a temperature of 22–24°C for about 24 h before weighing. The stability of mass readings was verified by weighting the laboratory blank filters before, after and during each weighing session. The electrostatic charges of substrate and filter materials were eliminated with a static neutralizer (500uCi Po210, NRD LLC, Grand Island, NY) before each weighing.
The chemical components of PM samples were analyzed at Wisconsin State Lab of Hygiene at University of Wisconsin—Madison. Daily (24 h) samples from the each impactor were combined into weekly composites and analyzed for chemical speciation. Both the Zefluor and quartz fiber filters were cut into four equal parts. One quarter of the quartz fiber filters was analyzed by an Ion Chromatography (IC) for inorganic ions including chloride, nitrate, phosphate, sulfate, sodium, ammonium and potassium (CitationKerr et al. 2004). The second quarter of the quartz fiber filters was analyzed by the Thermal Evolution/Optical Transmittance (TOT) analysis (CitationSchauer et al. 2003; CitationBirch and Cary 1996) to determine the elemental and organic carbon (EC and OC) levels. The third set of the quartz fiber filters was composited for the whole 7 week period at each site and analyzed by a Gas Chromatography/Mass Spectrometry (GC/MS) for 92 different organic compounds (CitationZheng et al. 2002; CitationChowdhury, 2007; CitationSchauer et al. 1999). The remaining quarter of quartz filter samples was archived for future analysis.
Similarly, one of the four sets of Zefluor filters were composited for the whole 7 week period at each site and for each PM range and analyzed by Inductively Coupled Plasma Mass Spectrometer (ICP-MS) to determine 52 trace elements (CitationHerner et al. 2006). The second set of Zefluor filters was also composited for the whole 7 week period at each site and analyzed for water-soluble elemental content. Samples were leached in high purity water and analyzed using a magnetic sector inductively coupled plasma mass spectrometer (HR-ICPMS, Finnigan Element 2). Three internal standards, gallium, indium, and bismuth were used for the HR-ICPMS analysis along with authentic standards for each quantified elements. The remaining samples were archived for future toxicity analysis.
Chemical Mass Closure (CMC)
The sum of mass concentrations obtained from nine chemical components was used to assess the extent to which the gravimetrically measured particulate mass could be reconstructed from the sum of the measured chemical components (CitationSillanpää et al. 2006). The chemical constituents were grouped into nine components as follows: ammonium (NH4 +), nitrate (NO3 –), non-sea salt sulfate (nss-SO4 2–), soil-derived compounds (crustal soil), sea salt, other elements, elemental carbon (EC), particulate organic matter (POM) and unidentified matter (UM). summarizes the formula for calculating the grouped chemical components. The nss-SO4 2– was calculated from the measured SO4 2–, Na+ and a standard seawater parameter; Sea salt concentrations were also estimated by multiplying Na+ concentrations by a standard seawater parameter (3.25) (CitationBrewer 1975). Si, Al, Ca, Fe, and K, appear predominantly as oxides, used to calculate the soil-derived components (CitationBrook et al. 1997). Sum of other elements, which include all the trace elements except major soil and sea salt elements, represents mostly the metal content of the particles. These elements are generated by a great diversity of sources, including metal industry, automotive vehicles (by abrasion of brakes, clutch, etc.) and dust resuspension (containing minor soil elements). The POM was obtained by multiplying the thermo-optically measured OC by a factor of 1.4 (CitationTurpin and Lim 2001; CitationRussell 2003), which roughly converts the carbon mass of organic compounds to its total mass by including other elements. It should be noted that there are uncertainties associated with use of 1.4 as a multiplier for converting measured carbon to organic carbon, leading to uncertainties in estimating the total PM mass by means of CMC. The average organic molecular weight per carbon weight is subject to change with location, season, and time, due to changes in the particular organic mixture (CitationTurpin and Lim 2001). A higher multiplication factor than 1.4 is expected for more oxygenated organic compounds (CitationSardar et al. 2005b), which would be more prevalent in areas impacted by aged aerosols. In a previous study by Turpin and Lim (CitationTurpin and Lim 2001), the range of 1.6 ± 0.2 was recommended to be used for urban areas, including that of Los Angeles. Thus, the factor of 1.4, which was used in this study, lies in the suggested range and has been used in previous recent studies in Los Angeles basin (e.g., CitationSardar et al. 2005b) as well as other urban areas (e.g., CitationSillanpää et al. 2006). The UM was obtained by subtracting the reconstructed mass (i.e., the sum of calculated chemical components) from the gravimetrically measured aerosol mass.
TABLE 1 The chemical components used in the mass closure studies
RESULTS AND DISCUSSION
Overview of the Data
Weather station data collected during the sampling campaign at each site are presented in . Site 5, which was located at the pier, was somewhat colder (average T = 14.7°C), windier (average WS = 3.0 m/s) and more humid (average RH = 75%) than the other sites, as one would expect. Averaged meteorological data over the sampling period were similar across the other sites, with the average temperature, relative humidity and wind speed varying in the ranges of 16.6–19.1°C, 52–63%, and 0.8–2.3 m/s, respectively. These meteorological data reaffirm the overall climatological stability of Los Angeles and show that weather conditions did not have a considerable effect on differences of the PM and its components between the sampling sites. In most of the sites, the prevailing wind was from the southwest, except at Sites 1 and 3, where a major part of wind directed from north and northwest, respectively. It should be mentioned that meteorological data was available for only the weeks 6–7 of sampling at Site 1.
TABLE 2 Meteorological data during the sampling campaign at sampling sites
The mean mass concentrations of three PM size ranges measured at the sampling sites are presented in . The campaign mean PM10 concentrations varied in the range of 13.9-26.8 μ g/m3. The lowest PM10 concentrations were measured at Site 1 and Site 5 whereas the concentrations at the other sites were ≥ 22.8 μ g/m3. The mass distribution between three measured size ranges was relatively different among sampling sites. Quasi-UF particles were the dominant PM fraction (49%) at Site 1, whereas Site 6 was dominated by accumulation mode particles (37%). The coarse particles had the highest contribution to PM10 in the other four sites (37% to 50%).
Chemical Mass Closure (CMC)
The mass closure results of different particle size ranges at each sampling site are shown in . The major components of each size fraction and their contributions are described below.
FIG. 3 The contributions of nine chemical components to the mass of quasi-UF, accumulation mode and coarse particles measured at six sampling sites.
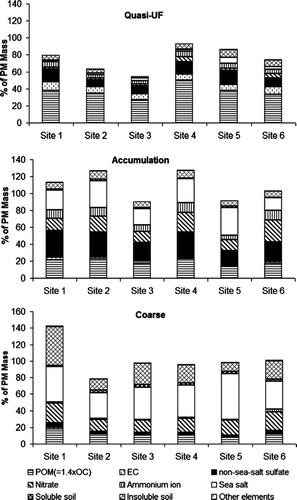
Quasi-Ultrafine Mode
The POM was the dominant component of quasi-UF particles, followed by nss-sulfate and EC. The highest POM contribution was observed at Site 4 (50%) whereas its contribution was lowest at Site 3 (27%). The organic carbon consists of both primary OC and secondary OC. The primary OC, emitted mainly from various combustion sources, is dominated by a mode around 0.1 to 0.2 μ m (CitationMaricq 2007; CitationHildemann et al. 1991), which makes it fall mostly in the quasi-UF particle range of our study. The generally high correlation between OC and EC (R2 = 0.66) in quasi-UF mode indicates that the majority of OC in that range is attributed to primary sources. The nss-sulfate is a secondary aerosol component that is predominantly formed in the atmosphere through the oxidation of sulfur dioxide (CitationRodhe 1999). The nss-sulfate contribution to total mass of quasi-UF varied in the range of 8.3–17%. Elemental carbon (EC) consists of graphite-like species and is formed by incomplete combustion of organic material (CitationSeinfeld and Pandis 1998). The EC contributed 7.0–11% to the quasi-UF particle mass. Insoluble soil elements, ammonium, sea salt, and nitrate contributed 1.6–9.0% to the quasi-UF particle mass. The contribution of sea salt (7.4%) at Site 5 was more than two times higher than that at the other sites. Recent studies have shown that sea spray can generate primary particles even in the ultrafine range (CitationO'Dowd and De Leeuw 2007). Elements associated with soluble soil had only a small impact (< 0.60%) on the quasi-UF particle mass. The gravimetric mass was somewhat higher than the reconstructed PM0.25 mass in each sampling site. The unidentified matter in Sites 1, 4, 5, and 6 ranged from 6.7% to 26%, which is within the methodological uncertainties as well as the uncertainties associated with the multiplication factor for OC. A higher fraction of unidentified matter was found in Site 2 (36%) and Site 3 (45%). The discrepancy may be due to the following factors: (1) the actual OC-to-POM ratio may differ from the applied factor (1.4) and it can vary between the sites and different size ranges; (2) as discussed earlier, the sample collected on Zefluor filters was used for mass measurement, while the major chemical components of reconstructed mass were quantified from the samples collected on quartz fiber filters. The cutoff size (D50) between quasi-UF and accumulation mode may also be slightly lower for the quartz fiber filter than the Zefluor filter, since other collection mechanisms in addition to inertial impaction play a role while using the porous impaction substrates (CitationSaarikoski et al. 2008).
It should be noted that positive artifact due to adsorption of gaseous organic vapors on the quartz after-filter used to collect quasi-UF particles might have occurred during our sampling. In previous studies in the Los Angeles basin (CitationKim et al. 2001; CitationSardar et al. 2005), it was shown that the positive organic artifacts approached a nearly constant saturation value of 1–1.5 μ g/m3 of OC for 24 h sampling periods at similar flow rates to those of our impactors, suggesting that these artifacts may be more important at lower PM mass concentrations than those reported in our study. Moreover, possible negative artifacts caused by volatilization of these organic vapors over a 24 hr sampling period may compensate for some of the adsorption artifacts (CitationSardar et al. 2005). Given the likely occurrence of these positive artifacts, however, the statements made in previous paragraphs regarding quasi-ultrafine OC (and POM) concentrations need to be qualified and put into perspective.
Accumulation Mode
The major chemical components found in the accumulation mode were nss-sulfate, sea salt, POM and nitrate—each of them accounted for 15–30% of the mass. The contributions of these major components were rather evenly distributed over the studied area. In contrast to quasi-UF and coarse particles, the accumulation mode particles remain longer in the atmosphere due to weak removal mechanics, which enables them to transport far from their sources and to disperse evenly through an urban area (CitationSeinfeld and Pandis 1998). Both ammonium and insoluble soil accounted roughly for 10% of the accumulation mode mass. The contributions of EC and soluble soil were in the magnitude of a few percent, whereas that of other elements was less than half percent. In the site located at the pier (Site 5), the EC contribution was about half compared to that measured at the other sites. This site can be influenced by some primary EC emissions, mainly in quasi-UF mode, from nearby ships. The reconstructed mass was close to or higher than the measured mass (92–128%) in accumulation mode, contrary to quasi-UF mode. The relatively higher reconstructed mass may be explained by the differences in cutoff sizes mentioned above hence a part of large ultrafine particles were collected on quartz fiber substrate while bypassed this stage in Zefluor sampling. In addition the actual OC-to-POM ratio may differ from the applied factor (1.4), which results in the under- or overestimation of the particulate organic matter.
Coarse Particles
Contrary to fine particles, coarse particles are typically composed of windblown dust, large sea salt particles from sea spray and mechanically generated anthropogenic particles, as well as biogenic particles (e.g., pollen, fungal spores, and bacteria). The major contributors to coarse particles mass were sea salt (31–55%) and insoluble soil (11–47%), followed by nitrate (14–23%), and POM (8–18%). The coarse particles in Site 5 were heavily dominated by sea salt (55%). Due to their size, these larger sea salt particles cannot be transported far from the source, which can be seen from the low sea salt concentration at Site 6. Among secondary inorganic ions, nitrate had clearly the highest contribution to coarse PM. Unlike the case of nitrate in fine particles, coarse particulate nitrate is predominantly formed in the reactions between nitric acid and sea salt or mineral compounds (CitationKerminen et al. 1998; CitationPio and Lopes 1998). Soluble nss-sulfate, soluble soil, ammonium, EC, and other elements had a minor contribution to this PM size fraction (< 3%). Excluding Site 1, the fraction of unidentified matter ranged from –0.9 % to 22%, which is within the methodological uncertainties. An underestimation in the reconstructed mass calculations is partly due to the assumption that the soil-related elements exist only as oxides, but other mineral compounds are likely present in the coarse PM fraction. The reconstructed coarse mass for Site 1 samples was 43% higher than the gravimetrically measured mass. This bias is likely due to the very low mass concentration of coarse particles (2.2 μ g/m3) at Site 1, which causes relatively high uncertainty in the result.
Organic Species Concentrations
presents the size-fractionated concentrations of n-alkanes. n-Alkanes concentrations varied from 6.4 to 43.3 ng/m3 in quasi-UF and from 2.3 to 27.4 ng/m3 in accumulation mode particles at all six sites. CitationFraser et al. (1997) has shown that n-alkanes are emitted from both anthropogenic sources (mainly combustion process) and biogenic sources (e.g., plants and bacterial activities). Site 5 had substantially lower levels of fine particulate n-alkanes (8.7 ng/m3) than Sites 1 to 4 (26.0–63.8 ng/m3). Site 5 was located upwind of local anthropogenic sources (e.g., vehicular sources), while Sites 1 to 4 were in the same vicinity as Site 5, but downwind of local anthropogenic sources. Site 2 had the highest particulate n-alkanes levels compare to other sites (63.8 ng/m3 in fine mode), which indicates a significant influence of local combustion sources (likely surface street traffic, with frequent vehicle acceleration-deceleration) on this site.
TABLE 3 Measured n-alkanes and calculated carbon preference index (CPI) for quasi-UF and accumulation mode particles at all sampling sites
Carbon preference index (CPI), i.e., the ratio of the concentrations of odd-carbon-to-even-carbon n-alkanes, is an indicator to differentiate between anthropogenic and biogenic source contribution to PM (CitationSimoneit 1986). Previous studies have shown that n-alkanes originating from anthropogenic sources have a CPI close to unity, whereas the CPI is generally higher (≥2) when the biogenic sources are dominant (CitationSimoneit 1986). The calculated CPIs at the six sampling sites are presented in . The CPIs varied from 0.74 (Site 2) to 1.36 (Site 6) in quasi-UF mode particles, and from 0.65 (Site 1) to 1.84 (Site 5) in the accumulation mode. These values confirm that anthropogenic emissions are the dominating sources of fine particulate matter emissions in the studied area. The relatively high CPI in accumulation mode at Site 5 (background site) implies a lower influence of anthropogenic sources. However, the low CPI of 0.9 in quasi-UF mode at this site could be due to primary emission of quasi-UF particles from ships located upwind of that site.
PAHs are also products of incomplete combustion of different organic matter and their composition and emission rate are dependent on combustion processes and atmospheric conditions (CitationManchester-Neesvig et al. 2003). The PAH concentrations in quasi-UFP and accumulation mode are shown in . In general, PAH concentrations were higher in quasi-UFP mode compared to accumulation mode, since they are species of primarily emitted aerosols, as it was shown in previous studies (CitationFine et al. 2004; CitationPhuleria et al. 2007). Tunnel studies suggested that heavy molecular weight PAHs (e.g., coronene) are primarily originated from poorly operated gasoline-powered vehicles (Miguel 1998; CitationLough et al. 2006; CitationPhuleria et al. 2006). The highest coronene concentration was observed at Site 2 (0.07 ng/m3), suggesting a possible impact from poorly operating gasoline powered engines associated with the local street traffic in that site. The transient operation of the vehicle engines, which is characteristic of urban surface street driving conditions (compared to the more even cruise-mode driving conditions of the freeways) may have also contributed to the higher concentrations of almost all organic species coming from combustion in that site. It should be noted that coronene was close to or under the detection limit of the GC/MS method in quasi-ultrafine particles at Sites 4, 5, and 6 and in accumulation mode at all the sites. Site 5 had the lowest level of particulate PAHs (0.1 ng/m3) since it was upwind of most of combustion sources. The low, but still measurable levels of PAHs at this site may originate from ship and boat traffic upwind of this site. The fine particulate PAHs concentrations at the studied sites ranged from 0.1 to 0.4 ng/m3, and were close to but somewhat lower than the range reported in previous studies (CitationManchester-Neesvig et al. 2003; CitationNing et al. 2007; Lough et al. 2006). It should be noted the PAH levels in this study represent concentrations averaged over an entire day. However, diurnal variation of different molecular weight PAHs are generally substantial, especially during the morning rush hour, when they can be up to 5–6 times higher than the rest of the day (CitationNing et al. 2007).
FIG. 4 Concentration of PAHs (classified by molecular weight), hopanes, steranes, and Levoglucosan in (a) quasi-UF and (b) accumulation mode. Error bars represent uncertainties in organics concentrations.
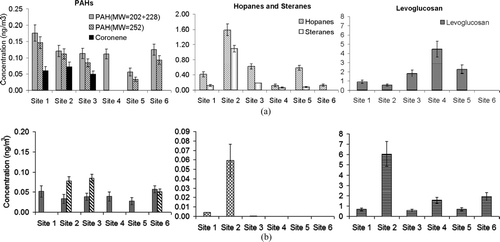
Hopanes and steranes appear in the particulate emissions of gasoline- and diesel-powered vehicles due to their presence in engine lubricating oil (CitationRogge et al. 1993, Citation1996; CitationSchauer et al. 1996, Citation2002). These compounds are used as organic markers of vehicular emissions (CitationFine et al. 2004; CitationSchauer et al. 1996, Citation1999, Citation2000, and Citation2002). Nonetheless, marine vessels may contribute to the urban concentrations of hopanes and steranes (CitationPeter et al. 1992). More than 96% of the detected hopanes and all the detected steranes were contained in quasi-UFP mode (). Sites 1, 2, 3, and 5, which were located within 3 km of the coast, had higher hopanes and steranes levels than site 4 (∼ 7 km from the coast) and Site 6 (∼ 40 km from the coast). This gradient of hopanes and steranes concentrations may be due to impact of marine vessels in addition to that of vehicular emissions in each site. For example, non-negligible hopanes and steranes were measured at Site 5 (0.7 ng/m3 of fine hopanes + steranes), although this site was clearly located upwind of most known vehicular sources in that area. The higher concentrations of hopanes and steranes were measured at Site 2 than all other sites (3.6 ng/m3 of fine hopanes + steranes), which indicates a high vehicular emission from either gasoline or diesel-powered vehicles in addition to marine vessels emissions affecting this site. The total fine particulate hopanes and steranes concentrations at the studied sites ranged from 0.1 to 3.6 ng/m3 and were consistent with the measured concentrations in previous studies in the Los Angeles area (CitationManchester-Neesvig et al. 2003; CitationFine et al. 2004).
The relative ratio of sum of hopanes to EC has been used in previous studies to distinguish between the influence of diesel- and gasoline-powered vehicles (Manchester-Neesving et al. 2003; CitationFine et al. 2004). Diesel powered vehicles contribute generally to hopanes and steranes concentrations and higher amounts of EC, whereas gasoline powered vehicles contribute to hopanes and steranes and smaller amounts of EC (CitationManchester-Neesvig et al. 2003; CitationSchauer et al; 1999; CitationFraser et al. 1997). shows the measured hopanes and EC levels (points) at all the sites compared to average levels measured near I-710 freeway (with the highest ratio of diesel powered vehicles in the entire state of California), shown as a solid line, and average levels measured near I-110 freeway (less than 3% diesel-powered vehicles), shown as a dashed line (CitationPhuleria et al. 2007). The plotted data points represent the fine fraction particles (i.e., the sum of quasi-ultrafine and accumulation mode concentrations). Site 5, which is the background site, had the lowest level of EC compare to the other sites, as it was anticipated. For the rest of the sites located close to harbor, the data points lie closer to the previously measured EC-to-hopanes ratio for diesel vehicle emissions, except for Site 2. Site 2 had the highest hopanes level accompanied with a relative high EC level. As it was discussed earlier, Site 2 is not situated in an area with an obvious high diesel vehicle impact, unlike Sites 1, 3, 4, and 6. The high hopane, sterane and EC levels maybe attributed to poorly maintained gasoline vehicles impacting that site as well as to the transient (frequent acceleration and abrupt stops as well as accelerating from stop lights) driving on the busy arterial roads that surround this site. When gasoline-powered vehicles perform hard accelerations, they temporarily reduce the efficiency of the catalytic converter, due to deviations from the stoichiometric fuel–air ratio (CitationMaricq et al. 1999). Under the more steady-state conditions such as freeway driving, catalytic converters remove CO, NO, volatile organic compounds, and UFP with high efficiency.
FIG. 5 Relationship between hopanes and elemental carbon; straight lines represent measured ratios in a previous study of urban PM2.5 in the proximity of freeways (CitationPhuleria et al. 2007).
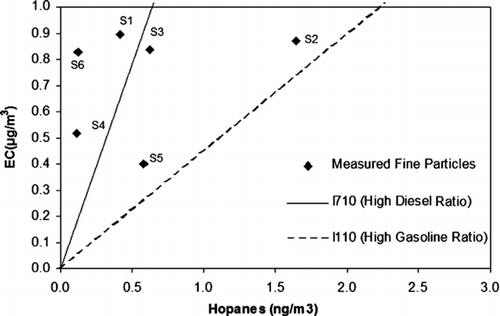
The average ratio of hopanes/OC over all the sampling sites was 0.21 ± 0.17 (ng/μ g), which agrees reasonably well with data measured near highways with either highly impact from heavy-duty vehicles (I-710, hopanes/OC = 0.42 ng/μ g), or from gasoline vehicles (I-110, hopanes/OC = 0.35 ng/μ g) (CitationPhuleria et al. 2007). These ratios support the notion that vehicular sources are the major contributor to OC. The OC/EC ratios varied from 3.4 (Site 3) to 6.1 (Site 1), which lie within the ranges of those measured near the I-710 (OC/EC = 2.0) and near the I-110 freeway (OC/EC = 7.7) (CitationPhuleria et al. 2007), inferring impacts from both diesel powered vehicles and gasoline vehicles.
Levoglucosan is a pyrolysis product of cellulose and it is regarded as a good tracer for wood burning emissions (CitationSchauer et al. 2000; CitationSimoneit et al. 1999; CitationFraser et al. 1997). The quasi-UF-to-accumulation mode ratio of levoglucosan varied substantially across the sites, which suggests that local wood burning dominates the levels of levoglucosan in the investigated area (). Sites 2 and 4 had substantially higher levoglucosan levels than the other sites; however, levoglucosan at Site 2 mainly appeared in the accumulation mode (6.1 ng/m3 in accumulation and 0.6 ng/m3 in quasi-UF mode), while at Site 4 it was measured in quasi-UFP mode (4.5 ng/m3 in quasi-UF and 1.6 ng/m3 in the accumulation mode). The levels of the fine PM levoglucosan during the sampling campaign (1.6 to 6.7 ng/m3) were comparable to those reported by CitationManchester-Neesvig et al. (2003) and CitationFine et al. (2004) in Los Angeles. The levoglucosan-to- OC ratios for PM2.5 varied from 0.6 ng/μ g (Site 1) to 2.0 ng/μ g (Site 2 and 4), which are far below the ratio reported for PM2.5 influenced by biomass burning (about 44.0 ng/μ g; Sheesley et al. 2007). These low ratios suggest that biomass burning is not a major source for particulate organic matter for the harbor communities.
Spatial Variance of Size Fractionated PM and its Components
The coefficient of variance (CV = standard deviation/mean) was determined for several measured species within each PM size range to investigate the spatial variation of these chemical components. To that end, during each week, mean concentrations across the six sampling sites (and standard deviations) were determined for PM, OC, EC, and ions data where the concentrations were available concurrently at all the sites. The PAHs were classified into three groups on the basis of their molecular weight (MW) (CitationPhuleria et al. 2007). The crustal material consisted of Al, Ca, Ti, and Fe. Organic species with more than one missing data point (mainly due to concentrations lower than the detection limit) were excluded from the CV analysis.
shows the CV of species for the three PM size ranges. PM mass in accumulation mode showed a relatively lower spatial variability (CV = 0.25 ± 0.06) compared to the quasi-UFP mode (CV = 0.47 ± 0.16) and the coarse mode (CV = 0.46 ± 0.01). The accumulation mode particles have higher residence times in the atmosphere, are subject to more intense atmospheric mixing and are thus more homogeneously dispersed over a large area. Conversely, the ultrafine and coarse mode PM tend to have local maxima closer to their emission sources and are removed faster from the atmosphere by diffusion and gravitational deposition (CitationSeinfeld and Pandis 1998).
FIG. 6 Coefficient of variances (CV) with standard deviation (SD) of selected chemical components at three size fractions: (a) quasi-UF mode, (b) accumulation mode, and (c) coarse mode. Error bars represent standard deviations.
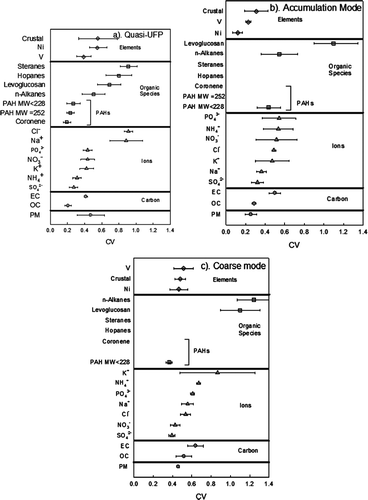
“In the quasi-UF particles, sodium and chloride are the most heterogeneously dispersed ions, with CV of 0.88 and 0.91, respectively. The high CV is mainly driven by the substantially higher concentration of these species at the background site (Site 5), which corresponds to high impact of sea salt generated by bubble bursting processes. EC, which is mainly emitted from diesel-powered vehicles, displayed relative high CV, which is indicative of the high variation of the influence of diesel vehicles on the six sites. By contrast, OC, which originates from most combustion sources (e.g., gasoline and diesel engine vehicles and wood smoke) showed relatively low CV values. Moreover, a fraction of OC can also be attributed to secondary formation processes in the atmosphere, which are regional in nature and result in a relatively homogenous dispersion of OC. Sulfate had the lowest CV values (hence the most homogeneous distribution over the investigated area) due to its mostly secondary origin. Steranes and hopanes showed also a high spatial variance (CV = 0.80 and 0.91, respectively), indicating a large variation in the impact of local traffic and marine sources on the different sites. The relative higher CV for these species was driven by the levels measured at Site 2, where the concentrations of hopanes and steranes were about 2–3 times higher than the rest of the sampling sites. PAHs had a low CV (0.2–0.3), at the same level with the CV of OC, and unlike the rest of the organic species in that size range.
In the accumulation mode particles, levoglucosan was the most spatially heterogeneous species, with a CV of 1.1. The high variation was mainly due to substantially higher concentration at Site 2 than the other sites, probably due to the presence of a local wood-burning source near Site 2. The majority of the other species in accumulation mode are rather evenly distributed over the sites compared to other modes, with CV values varying from 0.2–0.5.
In the coarse mode particles, levoglucosan and n-alkanes are the most spatially heterogeneous species with (CV = 1.1 and 1.2, respectively). Potassium and EC also showed relatively high spatial variances (0.9 and 0.7, respectively). Crustal elements and inorganic ions in that size range had CV values varying from 0.5–0.7, indicating moderate spatial heterogeneity
Elemental Constituents of PM
The concentration ranges of selected elements and their coefficient of variance (CV) over all the sites and for different size fractions are shown in . Na and S were the most abundant elements in all three size fractions, followed by Ca, Mg, K, Fe, and Al. The calculated CV in the quasi-UF particles varied from 0.22 (P) to 1.63 (Cr); in the accumulation mode, they varied from 0.12 (Ni) to 0.81 (Cd); and in the coarse mode, they varied from 0.40 (Zn) to 0.79 (Sb). presents the size fractionated Upper Continental Crust (UCC) enrichment factors (EFs) (CitationNtziachristos et al. 2007b) for selected elements. The concentration of each element was normalized to Al and then was divided by the relative abundance of the same element over Al in UCC (CitationTaylor and McLennan 1985). EFs in are averaged over the six sites; the error bars, denoting one standard deviation, suggest that the variability in EFs among sites was rather small. Trace elements were sorted in a decreasing order of their EF in fine PM mode. EFs close to 1 indicate crustal origin, while higher EFs indicate anthropogenic origin for a given element. For almost all the elements, the EF values were higher in the fine mode compare to the coarse mode, as the fine PM mostly originates from the anthropogenic sources. The lower coarse mode EFs were observed for Al, Fe, Ti, K, Mn, Cs, and K, indicating that these airborne species are products of resuspended soil dust. Even the fine PM fraction of these species had generally lower EFs compare to other elements, indicating again a crustal origin.
FIG. 7 Size fractionated results of (a) concentration ranges and coefficient of variances (CV) and (b) crustal enrichment factor for selected elements. Error bars represent standard deviations.
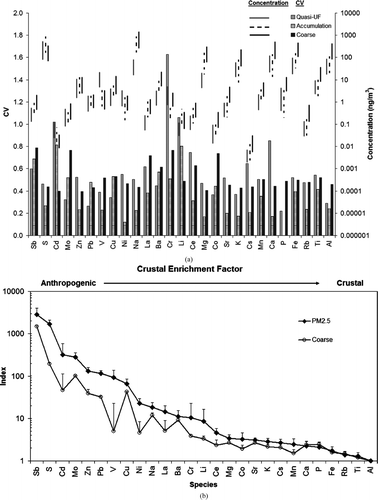
High EFs for Sb, S, Cd, Mo, Zn, Pb, and Cu were found in all the size fractions with higher values in fine mode (both quasi-ultrafine and accumulation mode). Most of these elements are generated from vehicular sources and some are ingredients of lube oil (CitationNtziachristos et al. 2007b). Cu, Sb, and Ba originate from vehicle brake abrasion (CitationSanders et al. 2003; CitationSternbeck et al. 2002). The concentrations of these three species were highly correlated across all size ranges, with R2 values ranging from 0.90–0.95 for Ba vs. Cu and Sb vs. Ba, respectively, confirming their common source; in fact, EFs for the coarse mode of these species are substantially higher than 1, supporting this argument that these elements are not products of soil dust resuspension. Mo is a component of lube-oil combustion since it is used as additives in oils. Zn is mostly a product of tire attrition (CitationSingh et al. 2002). Pb is attributed to wheel weights and gasoline exhaust in small amount (CitationSternbeck et al. 2002). High EFs of Sb, Mo, S, Sn, Pb, Zn, Cu, Ba, V, and Ni were also reported at other urban locations (CitationNtziachristos et al. 2007b; CitationLin et al. 2005; CitationBirmili et al. 2006). The high EF values for Na can be attributed to sea breeze as it is expected in coastal areas.
High EFs were found for V and Ni, as these elements are generated by fuel oil combustion, mainly by marine vessels (CitationIsakson et al. 2001; CitationLu et al. 2006; CitationCass and McRae 1983). Vanadium-to-nickel ratio has been used to distinguish between different emission sources so that the ratios higher than 1.5 mainly indicate fuel oil combustion, ratios around 1 indicate industrial sources (CitationIsakson and Persson et al. 2001) and smaller ratios indicate diesel and gasoline engine emissions (CitationLin et al. 2005). presents the scatter plot of vanadium versus nickel in the fine particle modes. Excluding Site 6 (at USC) a strong association between the two species was obtained across all the sites (r = 0.90). This association suggests a common dominant source for vanadium and nickel. A high slope of regression line (V/Ni = 4.2) indicates a fuel oil combustion source, which is likely from marine vessel emission in the region. The distinctly lower ratio at Site 6 (V/Ni = 1.9) suggests the influence of other important local Ni source(s) (e.g., local industries or vehicular sources) at this site.
FIG. 8 Vanadium concentrations (a) plotted versus nickel concentrations and (b) measured in quasi-ultrafine and accumulation mode at all the sites.
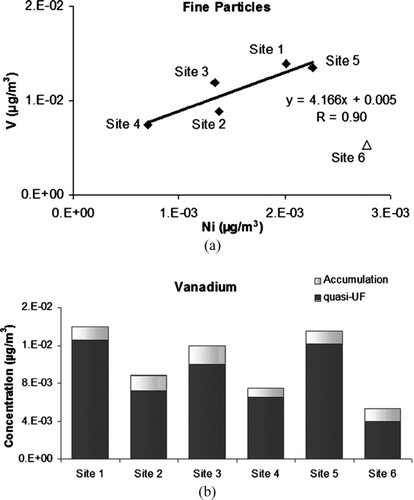
presents the variation of the vanadium concentrations measured over all the sampling sites. More than 80% of vanadium concentrations were in the quasi-UF mode. Site 5 and Site 1 showed the highest vanadium concentrations, followed by Site 3 and Site 2—all these sites were located within 3 km from the coast. Relative to the vanadium concentration measured at the coastal site (Site 5), the concentrations dropped to about 62% at Site 4 (7 km from the coast) and 49% at Site 6 (40 km from the coast). This gradient further confirms the major contribution of marine vessels to vanadium concentrations. presents the scatter plots and correlation coefficients of vanadium versus sulfur at all sites. Vanadium and sulfur were highly correlated in the quasi-UF mode (r = 0.72), whereas there was lack of correlation in the accumulation mode (r = 0.03). These results suggest that sulfur in quasi-UF mode mainly comes from similar sources to vanadium, i.e., mainly bunker-fuel combustion from marine vessels (CitationLin et al. 2005). By contrast, sulfur in accumulation mode is mainly in the form of ammonium sulfate which is produced by secondary reactions, hence the low correlation with V.
FIG. 9 Relationships between vanadium and sulfur concentrations for (a) quasi-UF and (b) accumulation fractions.
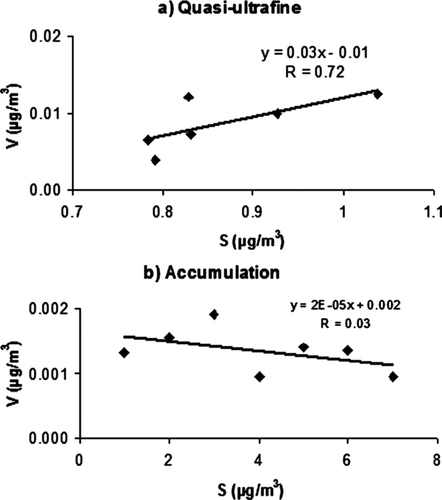
There was no significant correlation (p < 0.05) between V and Ni with OC and EC in different PM size fractions (). The weak association further supports the argument that marine vessels are probably not the major contributor to particulate EC and OC; even in areas proximal to the largest US harbor, the carbonaceous content of PM is generally emitted from vehicular sources. The V/OC ratio varied from 1.8 ng/μ g (Site 6, Los Angeles Downtown Site) to 7.1 ng/μ g (at Site 5, the background site, influenced by marine vessels). The Ni/OC ratio varied from 2.0 ng/μ g (Site 5) to 12.0 ng/μ g (Site 4). CitationYuan et al. (2006) reported V/OC and Ni/OC for residual oil combustion of about 160 and 70 ng/μ g, respectively. This corroborates the minor overall contribution of residual oil combustion sources to the measured EC and OC concentrations. Slightly higher, but still not significant correlations were found between Ni and EC-OC in the accumulation mode, which may be due to the contribution of vehicular sources to airborne Ni in addition to marine vessels.
TABLE 4 Pearson number and P-values of correlation between V and Ni with EC and OC in different size fractions of PM
SUMMARY AND CONCLUSIONS
Size fractioned PM and its chemical compositions were investigated in the communities of Los Angeles harbor. The major mass contributions in the quasi-UF fraction were particulate organic matter (POM), nss-sulfate and EC; in the accumulation mode fraction were nss-sulfate, sea salt, POM, and nitrate; and in the coarse fraction were sea salt and insoluble soil. In general, PM and its components in accumulation mode showed relatively lower spatial variability compare to the quasi-UF and the coarse modes. The carbon preference index (CPI) for quasi-UF and accumulation mode particles varied from 0.65 to 1.84 among sites, which is in the range of previous findings in areas with high influence of anthropogenic sources. In sites located close to harbor, the average n-Alkanes and PAHs levels were respectively about 3 and 5 times higher than their corresponding levels at a site located in vicinity of harbor, but upwind of most of local sources. The ratio of hopanes to EC and hopanes to OC over all the sites were in the range of previous roadside measurements near freeways with variable volume of diesel truck traffic. High overall correlations of vanadium with nickel (R = 0.9), as well as a considerable gradient of vanadium concentrations with distance from the coast, suggests marine vessels as the major sources of these elements.
This research was supported by the Southern California Particle Center (SCPC), funded by EPA under the STAR program through Grant RD-8324-1301-0 to the University of Southern California. The research described herein has not been subjected to the agency's required peer and policy review and therefore does not necessarily reflect the views of the agency, and no official endorsement should be inferred. Mention of trade names or commercial products does not constitute an endorsement or recommendation for use. We thank the following staff at the Wisconsin State Lab of Hygiene (WSLH) for conducting EC-OC, ion chromatography, GC/MS, and ICPMS analysis: Brandon Shelton, Jeff DeMinter, Martin Shafer, Pat Gorski, and Joel Overdier. We are thankful for the port of Long Beach, Dinesh Mohda, and the staff at the Long Beach Job Corps Center, Mr. Baltazar Alvarez (and, of course, South Coast AQMD) for the help in the sample collection at the Sites 2, 3, and 5.
Notes
b WSS is based on ICP-MS extraction of the soluble portion of these species.
c WIS is based on ICP-MS extraction of the total-soluble portion of these species.
d [Si] has been estimated from [Al], [Si] = 3 x [Al] (CitationSillanpää et al., 2006).
a CPI = ΣC (2n + 1)/ΣC(2n), n = 7–20.
REFERENCES
- Allen , J. O. , Hughes , L. S. , Salmon , L. G. , Mayo , P. R. , Johnson , R. J. and Cass , G. R. 2000 . Characterization and Evolution of Primary and Secondary Aerosols During PM2.5 and PM10 Episodes in the South Coast Air Basin, Report A-22 to the Coordinating Research Council (CRC)
- Anderson , H. R. 2000 . Differential Eidemiology of Ambient Aerosols, . Phil. Trans. Royal Soc. London. Series A, Math. & Phys. Sci. , 1775 : 2771 – 2785 .
- Arhami , M. , Kuhn , T. , Fine , P. M. , Delfino , R. J. and Sioutas , C. 2006 . Effects of Sampling Artifacts and Operating Parameters on the Performance of a Semi-continuous Particulate Elemental Carbon/Organic Carbon Monitor, . Environ. Sci. Technol , 40 : 945 – 954 .
- Atkinson , R. W. , Anderson , H. R. , Sunyer , J. , Ayres , J. , Baccini , M. , Vonk , J. M. , Boumghar , A. , Forastiere , F. , Forsberg , B. , Touloumi , G. , Schwartz , J. and Katsouyanni , K. 2001 . Acute Effects of Prticulate Air Pollution on Respiratory Admissions—Results from APHEA 2 Project. . Amer. J. Resp. Critical Care Med. , 164 ( 10 ) : 1860 – 1866 .
- Birch , M. E. and Cary , R. A. 1996 . Elemental Carbon-Based Method for Monitoring Occupational Exposures to Particulate Diesel Exhaust, . Aerosol Sci. Tech , 25 : 221 – 241 .
- Birmili , W. , Allen , A. G. , Bary , F. and Harrison , R. M. 2006 . Trace Metal Concentrations and Water Solubility in Size-Fractionated Atmospheric Particles and Influence of Road Traffic, . Environ. Sci. Technol. , 40 : 1144 – 1153 .
- Brewer , P. G. 1975 . Minor Elements in Sea Water, . Chem. Oceanog , 1 : 417 – 425 .
- Brook , J. F. , Dann , T. F. and Burnett , R. T. 1997 . The Relationship among TSP, PM10, PM2.5, and Inorganic Constituents of Atmospheric Particulate Matter at Multiple Canadian locations . J. Air & Waste Manage. Assoc. , 47 : 2 – 19 .
- Brunekreef , B. and Forsberg , B. 2005 . Epidemiological Evidence of Effects of Coarse Airborne Particles on Health, . Europ. Resp. J. , 26 ( 2 ) : 309 – 318 .
- Cass , G. R. and McRae , G. J. 1983 . Source-Receptor Reconciliation of Routine Air Monitoring Data for Trace Metals: An Emission Inventory Assisted Approach, . Environ. Sci. Technol. , 17 ( 3 ) : 129 – 139 .
- Cass , G. R. , Hughes , L. A. , Bhave , P. , Kleeman , M. J. , Allen , J. O. and Salmon , L. G. 2000 . The Chemical Composition of Atmospheric Ultrafine Particles, . Philosophical Transactions of the Royal Society of London Series A-Mathematical Physical and Engineering Sci. , 358 ( 1775 ) : 2581 – 2592 .
- Christoforou , C. S. , Salmon , L. G. , Hannigan , M. P. , Solomon , P. A. and Cass , G. R. 2000 . Trends in Fine Particle Concentration and Chemical Composition in Southern California, . J. Air Waste Manag. , 50 ( 1 ) : 43 – 53 .
- Chowdhury , Z. , Zheng , M. , Schauer , J. J. , Sheesley , R. J. , Salmon , L. G. , Cass , G. R. and Russell , A G. 2007 . Speciation of Ambient Fine Organic Carbon Particles and Source Apportionment of PM2.5 in Indian cities, . J. Geophys. Res , 112 : D15303
- Delfino , R. J. , Sioutas , C. and Malik , S. 2005 . Potential Role of Ultrafine Particles in Associations Between Airborne Particle Mass and Cardiovascular Health, . Environ Health Perspec. , 113 ( 8 ) : 934 – 946 .
- Donaldson , K. , Brown , D. , Clouter , A. , Duffin , R. , MacNee , W. , Renwick , L. , Tran , L. and Stone , V. 2002 . The Pulmonary Toxicology of Ultrafine Particles, . J. Aerosol Med.—Deposition Clearance and Effects in the Lung , 15 ( 2 ) : 213 – 220 .
- Dreher , K. L. , Jaskot , R. H. , Lehmann , J. R. , Richards , J. H. , McGee , J. K. , Ghio , A. J. and Costa , D. L. 1997 . Soluble Transition Metals Mediate Residual Oil Fly Ash Induced Acute Lung Injury . J. Tox. Environ. Health , : 285 – 305 .
- Fine , P. M. , Chakrabarti , B. , Krudysz , M. , Schauer , J. J. and Sioutas , C. 2004 . Seasonal, Spatial, and Diurnal Variations of Individual Organic Compound Constituents of Ultrafine and Accumulation Mode Pm in the Los Angeles Basin, . Environ. Sci. Technol , 38 : 1296 – 1304 .
- Fraser , M. P. , Cass , G. R. , Simoneit , B. R. T. and Rasmussen , R. A. 1997 . Air Quality Model Evaluation Data for Organics C2-C36 Non-Aromatic Hydrocarbons, . Environ. Sci. Technol. , 31 : 2356 – 2367 .
- Herner , J. D. , Green , P. G. and Kleeman , M. J. 2006 . Measuring the Trace Elemental Composition of Size-Resolved Airborne Particles, . Environ. Sci. Technol. , 40 ( 6 ) : 1925 – 1933 .
- Hildemann , L. M. , Markowski , G. R. , Jones , M. C. and Cass , G. R. 1991 . Submicrometer Aerosol Mass Distributions of Emissions from Boilers, Fireplaces, Automobiles, Diesel Trucks, and Meat Cooking Operations. . Aerosol Sci. Technol. , 14 : 138 – 152 .
- Hughes , L. S. , Allen , J. O. , Kleeman , M. J. , Johnson , R. J. , Cass , G. R. , Gross , D. S. , Gard , E. E. , Galli , M. E. , Morrical , B. D. , Fergenson , D. P. , Dienes , T. , Noble , C. A. , Silva , P. J. and Prather , K. A. 1999 . Size and Composition Distribution of Atmospheric Particles in Southern California, . Environ. Sci. Technol. , 33 ( 20 ) : 3506 – 3515 .
- Isakson , J. , Persson , T. A. and Lindgren , E. S. 2001 . Identification and Assessment of Ship Emissions and their Effects in the Harbor of Gothenburg, Sweden, . Atmos. Environ. , 35 : 3659 – 3666 .
- Kerminen , V.-M , Teinilä , K. , Hillamo , R. and Pekkanen , T. 1998 . Substitution of Chloride in Sea-Salt Particles by Inorganic and Organic Anions, . J. Aerosol Sci , 29 : 929 – 942 .
- Kerr , S. C. , Schauer , J. J. and Rodger , B. 2004 . Regional Haze in Wisconsin: Sources and the Spatial Distribution, . J. Environ. Eng. and Sci. , 3 : 213 – 222 .
- Kim , B. M. , Cassmassi , J. , Hogo , H. and Zeldin , M. D. 2001 . Positive Organic Carbon Artifacts on Filter Medium during PM2.5 Sampling in the South Coast Air Basin, . Aerosol Sci. Technol. , 34 ( 1 ) : 35 – 41 .
- Kim , S. , Shen , S. , Sioutas , C. , Zhu , Y. F. and Hinds , W. C. 2002 . Size Distribution and Diurnal and Seasonal Trends of Ultrafine Particles in Source and Receptor Sites of the Los Angeles Basin, . J. Air Waste Manag. Assoc. , 52 : 297 – 307 .
- Lin , C. C. , Chen , S. J. , Huang , K. L. , Hwang , W. I. , Chang-Chien , G. P. and Lin , W. Y. 2005 . Characteristics of Metals in Nano/Ultrafine/Fine/Coarse Particles Collected Beside a Heavily Trafficked Road, . Environ. Sci. Technol. , 39 ( 21 ) : 8113 – 8122 .
- Lighty , J. S. , Veranth , J. M. and Sarofim , A. F. 2000 . Combustion Aerosols: Factors Governing Their Size and Composition and Implications to Human Health, . J. Air Waste Manage. Assoc. , 50 ( 9 ) : 1565 – 1618 .
- Li , N. , Sioutas , C. , Cho , A. , Schmitz , D. , Misra , C. , Sempf , J. , Wang , M. Y. , Oberley , T. , Froines , J. and Nel , A. 2003 . Ultrafine Particulate Pollutants Induce Oxidative Stress and Mitochondrial Damage, . Environ. Health Perspec. , 111 : 455 – 460 .
- Lough , G. C. , Schauer , J. J. and Lawson , D. R. 2006 . Day-of-Week Trends in Carbonaceous Aerosol Composition in the Urban Atmosphere, . Atmos. Sci. , 40 : 4137 – 4149 .
- Lu , G. , Brook , J. R. , Alfarra , M. R. , Anlauf , K. , Leaitch , W. R. , Sharma , S. , Wang , D. , Worsnop , D. R. and Phinney , L. 2006 . Identification and Characterization of Inland Ship Plumes Over Vancouver, BC. . Atmos. Environ. , 40 : 2767 – 2782 .
- Manchester-Neesvig , J. B. , Schauer , J. J. and Cass , G. R. 2003 . The Distribution of Particle-Phase Organic Compounds in the Atmosphere and Their Use for Source Apportionment during the Southern California Children's Health Study, . J. Air Waste Manag. Assoc. , 53 : 1065 – 1079 .
- Maricq , M. M. , Podsiadlik , D. H. and Chase , R. E. 1999 . Gasoline Vehicle Particle Size Distributions: Comparison of Steady State, FTP, and US06 Measurements, . Environ. Sci. Technol. , 33 ( 12 ) : 2007 – 2015 .
- Maricq , M. M. 2007 . Chemical Characterization of Particulate Emissions from Diesel Engines: A Review, . J. Aerosol Sci. , 38 ( 11 ) : 1079 – 1118 .
- Misra , C. , Singh , M. , Shen , S. , Sioutas , C. and Hall , P. A. 2002 . Development and Evaluation of a Personal Cascade Impactor Sampler (PCIS). . J. Aerosol Sci. , 33 : 1027 – 1047 .
- Ning , Z. , Geller , M. D. , Moore , K. F. , Sheesley , R. , Schauer , J. J. and Sioutas , C. 2007 . Daily Variation in Chemical Characteristics of Urban Ultrafine Aerosols and Inference of Their Sources, . Environ Sci. Technol. , 41 ( 17 ) : 6000 – 6006 .
- Ntziachristos , L. , Ning , Z. , Geller , M. D and Sioutas , C. 2007a . Particle Concentration and Characteristics Near a Major Freeway with Heavy-Duty Diesel Traffic, . Environ. Sci. Technol. , 41 ( 7 ) : 2223 – 2230 .
- Ntziachristos , L. , Ning , Z. , Geller , M. D. , Sheesley , J. J. , Schauer , J. J. and Sioutas , C. 2007b . Fine, Ultrafine and Nanoparticle Trace Element Compositions Near a Major Freeway with a High Heavy-Duty Diesel Fraction, . Atmos. Environ. , 41 : 5684 – 5696 .
- Oberdörster , G. 1996 . Significance of Particle Parameters in the Evaluation of Exposure-Dose-Response Relationships of Inhaled Particles, . Particulate Sci. Technol. , 14 : 135 – 151 .
- Oberdörster , G. 2001 . Pulmonary Effects of Inhaled Ultrafine Particles, . International Archives of Occupational and Environ. Health , 74 : 1 – 8 .
- O'Dowd , C. D. and De Leeuw , G. 2007 . Marine Aerosol: A Review of the Current Knowledge, . Philosophical Transactions of the Royal Society A-Mathematical Physical and Engineering Sci , 365 ( 1856 ) : 1753 – 1774 .
- Pandis , S. N. , Harley , R. A. , Cass , G. R. and Seinfeld , J. H. 1992 . Secondary Organic Aerosol Formation and Transport, . Atmos. Environ. , 26 : 2269 – 2282 .
- Peters , K. E. , Scheuerman , L. , Lee , C. Y. , Moldowan , J. M. , Reynolds , R. N. and Pena , M. M. 1992 . Effect of Refinery Processes on Biological Markers, . Energy and Fuel , 6 : 560 – 577 .
- Pio , C. A. and Lopes , D. A. 1998 . Chlorine Loss from Marine Aerosol in a Coastal Atmosphere, . J. Geophys. Res. , 103 : 25263 – 25272 .
- Phuleria , H. C. , Geller , M. D. , Fine , P. M. and Sioutas , C. 2006 . Size-Resolved Emissions of Organic Tracers from Light- and Heavy-Duty Vehicles Measured in a California Roadway Tunnel, . Environ. Sci. Technol. , 40 : 4109 – 4118 .
- Phuleria , H. C. , Sheesley , R. J. , Schauer , J. J. , Fine , P. M. and Sioutas , C. 2007 . Roadside Measurements of Size-segregated Particulate Organic Compounds near Gasoline and Diesel-Dominated Freeways in Los Angeles, CA, . Atmos. Environ. , 41 : 4653 – 4671 .
- Rodhe , H. 1999 . Human Impact on the Atmospheric Sulfur Balance, . Tellus , 51 : 110 – 122 .
- Rogge , W. F. , Hildemann , L. M. , Mazurek , M. A. , Cass , G. R. and Simoneit , B. R. T. 1993 . Sources of Fine Organic Aerosol. 2. Noncatalyst and Catalyst-Equipped Automobiles and Heavy-Duty Diesel Trucks, . Environ. Sci. Technol. , 27 : 636 – 651 .
- Rose , D. , Wehner , B. , Ketzel , M. , Engler , C. , Voigtländer , J. , Tuch , T. and Wiedensohler , A. 2006 . Atmospheric Number Size Distributions of Soot Particles and Estimation of Emission Factors. . Atmos. Phys. Chem. , 6 : 1021 – 1031 .
- Rogge , W. F. , Hildemann , L. M. , Mazurek , M. A. , Cass , G. R. and Simoneit , B. R. T. 1996 . Mathematical Modeling of Atmospheric Fine Particle-Associated Primary Organic Compound Concentrations, . J. Geophys. Res , 101 ( D14 ) : 19379 – 19394 .
- Russell , A. G. and Cass , G. R. 1986 . Verification of a Mathematical Model for Aerosol Nitrate and Nitric Acid Formation and its Use for Control Measure Source, . Atmos. Environ. , : 2011 – 2025 .
- Russell , L. M. 2003 . Aerosol Organic-Mass-to-Organic-Carbon Ratio Measurements. . Environ. Sci. Technol. , 37 : 2982 – 2987 .
- Samoli , E. , Analitis , A. , Touloumi , G. , Schwartz , J. , Anderson , H. R. , Sunyer , J. , Bisanti , L. , Zmirou , D. , Vonk , J. M. , Pekkanen , J. , Goodman , P. , Paldy , A. , Schindler , C. and Katsouyanni , K. 2005 . Estimating the Exposure-Response Relationships between Particulate Matter and Mortality within the APHEA Multicity Project, . Environ. Health Perspect. , 113 ( 1 ) : 88 – 95 .
- Saarikoski , S. , Frey , A. , Mäkelä , T. and Hillamo , R. 2008 . Size Distribution Measurement of Carbonaceous Particulate Matter using a Low Pressure Impactor with Quartz Fiber Substrates. . Aerosol Sci. Technol. , In press.
- Salonen , R. O. , Halinen , A. I. , Pekkanen , A. S. , Hirvonen , M. R. , Sillanpaa , M. , Hillamo , R. , Shi , T. M. , Borm , P. , Sandell , E. , Koskentalo , T. and Aarnio , P. 2004 . Chemical and In Vitro Toxicologic Characterization of Wintertime and Springtime Urban-Air Particles with an Aerodynamic Diameter Below 10 μ m in Helsinki, . Scandinavian J. Work and Environ. Health , 30 : 80 – 90 .
- Sanders , P. G. , Xu , N. , Dalka , T. M. and Maricq , M. M. 2003 . Airborne Brake Wear Debris: Size Distributions, Composition, and a Comparison of Dynamometer and Vehicle Tests, . Environ. Sci. Technol. , 37 : 4060 – 4069 .
- Sardar , S. B. , Fine , P. M. , Mayo , P. R. and Sioutas , C. 2005a . Size-Fractionated Measurements of Ambient Ultrafine Particle Chemical Composition in Los Angeles using the NanoMOUDI, . Environ. Sci. Technol. , 39 : 932 – 944 .
- Sardar , S. B. , Fine , P. M. and Sioutas , C . 2005b . Seasonal and Spatial Variability of the Size-Resolved Chemical Composition of Particulate Matter (PM10) in the Los Angeles Basin, . J. Geophys. Res. , 110:D07S08. doi: 10.1028/2004JD004627.
- Schauer , J. J. , Rogge , W. F. , Hildemann , L. M. , Mazurek , M. A. , Cass , G. R. and Simoneit , B. R. T. 1996 . Source Apportionment of Airborne Particulate Matter Using Organic Compounds as Tracers, . Atmos. Environ. , 30 : 3837 – 3855 .
- Schauer , J. J. , Kleeman , M. J. , Cass , G. R. and Simoneit , B. R. T. 1999 . Measurement of Emissions from Air Pollution Sources 1. C1 through C29 Organic Compounds from Meat Charbroiling, . Environ. Sci. Technol. , 33 : 1566 – 1577 .
- Schauer , J. J. and Cass , G. R. 2000 . Source Apportionment of Wintertime Gas-Phase and Particle-Phase Air Pollutants Using Organic Compounds as Tracers, . Environ. Sci. Technol. , 34 : 1821 – 1832 .
- Schauer , J. J. , Kleeman , M. J. , Cass , G. R. and Simoneit , B. R. T. 2002 . Measurement of Emissions from Air Pollution Sources. 5. C1-C32 Organic Compounds from Gasoline-Powered Motor Vehicles, . Environ. Sci. Technol. , 36 : 1169 – 1180 .
- Schauer , J. J. , Mader , B. T. , Deminter , J. T. , Heidemann , G. , Bae , M. S. , Seinfeld , J. H. , Flagan , R. C. , Cary , R. A. , Smith , D. , Huebert , B. J. , Bertram , T. , Howell , S. , Kline , J. T. , Quinn , P. , Bates , T. , Turpin , B. , Lim , H. J. , Yu , J. Z. , Yang , H. and Keywood , M. D. 2003 . ACE—Asia Intercomparison of a Thermal–Optical Method for the Determination of Particle-Phase Organic and Elemental Carbon, . Environ. Sci. Technol. , 37 : 993 – 1001 .
- Schauer , J. J. , Christensen , C. G. , Kittelson , D. B. , Johnson , J. P. and Watts , W. F. 2008 . Impact of Ambient Temperatures and Driving Conditions on the Chemical Composition of Particulate Matter Emissions from Non-Smoking Gasoline-Powered Motor Vehicles, . Aerosol Sci. Technol. , In press.
- Seinfeld , J. H. and Pandis , S. N. 1998 . Atmospheric Chemistry and Physics: From Air Pollution to Climate Change , New York : John Wiley .
- Sheesley , R. J. , Schauer , J. J. , Zheng , M. and Wang , B. 2007 . Sensitivity of Molecular Marker-Based CMB Models to Biomass Burning Source Profiles, . Atmos. Environ. , 41 : 9050 – 9063 .
- Sillanpaa , M. , Hillamo , R. , Saarikoski , S. , Frey , A. , Pennanen , A. , Makkonen , U. , Spolnik , Z. , Van Grieken , R. , Branis , M. , Brunekreef , B. , Chalbot , M. C. , Kuhlbusch , T. , Sunyer , J. , Kerminen , V. M. , Kulmala , M. and Salonen , R. O. 2006 . Chemical Composition and Mass Closure of Particulate Matter at Six Urban Sites in Europe, . Atmos. Environ. , 40 : S212 – S223 .
- Simoneit , B. R. T. 1986 . Characterization of Organic-Constituents in Aerosols in Relation to Their Origin and Transports: A Review, . Int. J. Environ. Anal. Chem. , 23 : 207 – 237 .
- Simoneit , B. R. T. 1999 . A Review of Biomarker Compounds as Source Indicators and Tracers for Air Pollution, . Environ Sci. Poll. Res. , 6 ( 3 ) : 159 – 169 .
- Singh , M. , Jaques , P. A. and Sioutas , C. 2002 . Size Distribution and Diurnal Characteristics of Particle-Bound Metals in Source and Receptor Sites of The Los Angeles Basin, . Atmos. Environ. , 36 : 1675 – 1689 .
- Singh , M. , Misra , C. and Sioutas , C. 2003 . Field Evaluation of a Personal Cascade Impactor Sampler (PCIS). . Atmos. Environ. , 37 : 4781 – 4793 .
- Sternbeck , J. , Sjodin , A. and Andreasson , K. 2002 . Metal Emissions from Road Traffic and the Influence of Resuspension—Results from Two Tunnel Studies, . Atmos. Environ. , 36 : 4735 – 4744 .
- Taylor , S. R. and McLennan , S. M. 1985 . The Continental Crust: Its Composition and Evolution , Oxford, Boston, Palo Alto, Victoria : Blackwell Scientific Publications .
- Turpin , B. J. and Lim , H. J. 2001 . Species Contributions to PM2.5 Mass c Concentrations: Revisiting Common Assumptions for Estimating Organic Mass, . Aerosol Sci. Technol. , 35 : 602 – 610 .
- World Health Organization (WHO) . 2003 . Health Aspects of Air Pollution with Particulate Matter, Ozone and Nitrogen Dioxide , Copenhagen, , Denmark : WHO Regional Office for Europe . Report EUR/03/5042688 of Working Group, Bonn, Germany, 13–15 January 2003.
- Xia , T. , Korge , T. , Weiss , J. , Li , N. , Venkatesan , I. , Sioutas , C. and Nel , A. 2004 . Quinones and Aromatic Chemical Compounds in Particulate Matter Induce Mitochondrial dysfunction: Implications for Ultrafine Particle Toxicity, . Environ. Health. Perspec. , 112 ( 14 ) : 1347 – 1358 .
- Yuan , Z. , Lau , A. K. H. , Zhang , H. , Yu , J. Z. , Louie , P. K. K. and Funge , J. C. H. 2006 . Identification and Spatiotemporal Variations of Dominant PM10 Sources Over Hong Kong, . Atmos. Environ. , 40 : 1803 – 1815 .
- Zheng , M. , Cass , G. R. , Schauer , J. J. and Edgerton , E. S. 2002 . Source Apportionment of PM2.5 in the Southeastern United States Using Solvent Extractable Organic Compounds as Tracers, . Environ. Sci. Technol. , 36 : 2361 – 2371 .