Abstract
Integrating nephelometers are widely used for monitoring and research applications related to air pollution and climate. Several commercial versions of the instrument are available and are in wide use in the community. This article reports on results from a calibration and intercomparison workshop where several units of the three most widely used nephelometer models were tested with respect to their CO2 calibration accuracy and stability and non-idealities of their angular illumination function. Correction factors that result from the non-ideal illumination due to truncation of the sensing volumes in the near-forward and near-backward angular ranges and for non-Lambertian illumination from the light sources are presented, in particular for two models that have not previously been tested in this respect. The correction factors ranged from 0.95 to 1.15 depending on the model of nephelometer and aerosol size distribution. Recommendations for operational data analysis in context of these and previous performance tests are presented.
1. INTRODUCTION
The integrating nephelometer provides a means of measuring light scattering coefficients of ambient aerosol particles with a high sensitivity and time resolution in a wide range of monitoring and research applications related to air pollution and climate. Accurate, direct measurements of the scattering and absorption coefficients of atmospheric aerosol particles are of importance for determining how particles affect radiative transfer and visibility in the atmosphere. The integrating nephelometer, as devised by CitationBeuttell and Brewer (1949), measures the particulate scattering coefficient, σ sp , by performing a geometrical integration of the light scattered from a sample volume illuminated by a Lambertian light source that is orthogonal to the axis of the detector. As such, the nephelometer provides a direct measure of scattering coefficient independent of the size, chemical composition, and physical state of the aerosol in an enclosed volume and it can be calibrated by gases with known scattering coefficients. Major assumptions inherent in this design are: (a) that the illumination is truly Lambertian over all angles, (b) that the light source dimension is small compared to length of the sensing volume (integration axis), and (c) that the forward and backward (near 0 and 180 degree) truncation limits of the angular integration are small. To the extent that these assumptions are not met (non-idealities in realizing the design) the measured scattering coefficient may be in error and needs to be corrected. Further description of the assumptions and non-idealities have been given by CitationAnderson et al. (1996) and also by CitationMoosmüller and Arnott (2003). A historical and scientific review of this type of instrument was presented by CitationHeintzenberg and Charlson (1996).
The accuracy and uncertainty of several models of nephelometers has been determined for gas calibrations and also by closure studies using monodisperse aerosols over a range of sizes by CitationAnderson et al. (1996) and CitationHeintzenberg et al. (2006). These results have been evaluated by CitationAnderson and Ogren (1998) and an empirical formula was determined to correct for the truncation and illumination non-idealities of one model of nephelometer (model 3563, TSI Inc, St. Paul, MN, USA). The effect of the non-idealities was that the measured scattering coefficient was generally less than the true value and that this systematic error is strongly dependent on particle size and more significant for particles larger than ca. 1 micrometer in diameter. Previous characterizations of nephelometers have not included direct measurement of the illumination, truncation functions for models other than the TSI 3563. CitationHeintzenberg et al. (2006) modeled the function for two other models and estimated their illumination functions via an optimization of closure with the TSI units.
An intercomparison and calibration workshop was conducted in March of 2007 at the World Calibration Centre for Aerosol Physics (WCCAP) of the World Meteorological Organization—Global Atmospheric Watch (WMO-GAW) at the Leibniz Institute for Tropospheric Research in Leipzig, Germany, to further characterize nephelometers from three manufacturers. Our goals were: (a) to test the standard calibration and calibration procedures and (b) to test the light source's angular illumination functions and determine correction functions for the non-idealities.
2. METHODS
2.1. Instruments
Nephelometers from three manufacturers were investigated during the workshop (subsequently referred to as WCCAP2007) including the TSI model 3563, the Radiance Research model M903, and the Ecotech model 9003. Details of the manufacturer, number of units of each that were tested and their wavelength and angular integration ranges are given in . Multiple instruments were included in this study; the TSI units included manufacturing dates from 1996 to 2006 including one instrument used in the study by CitationAnderson et al. (1996).
TABLE 1 Nephelometer models and specifications
2.2. Light Source Characterization
The light sources were removed from the instruments and mounted on an optical bench for measurement of the angular distribution of light intensity, the illumination function. The light sources were operated identically to normal with respect to voltage and current and voltage pulse duty cycle as appropriate to the model.
A goniometer (positioning goniometer or goniometric stage) is a device used to rotate an object precisely about a fixed axis. In this case the nephelometers' light sources were fixed on an optical bench and a light collection optics on an arm fixed to the bench rotated through an angular range of 180 degrees as shown schematically in . The angular intensity function of the light sources was measured in steps of 5 degrees that were reduced to 2 degrees near 0 and 180 degrees. The goniometer arm was 24 cm in length and held a focused collection optics and light pipe connected to a spectrophotometer (model HR2000, Ocean Optics, Inc. Dunedin, FL) with a wavelength range of 400 nm to 900 nm. The full field of view of the collection optics was 8.3 degrees, which fully included the surface of the light sources in the case of the TSI and Radiance Research units, but only the most intensely illuminated, center of the Ecotech units. The illumination plane of the light source and the goniometer axis were aligned mechanically and also optically by directing a laser beam through the optical fiber (without collection lens) to the surface of the light source. The plane of motion of the arm was horizontal and perpendicular to the illumination plane of the light source. At 0 and 180 degrees the axis of the field of view of the goniometer optics was parallel to and coincident with and vertically centered on the plane of the illumination surface. The overall alignment and measurement uncertainty is estimated to be less than one degree.
2.3. Calibration Checks
Calibration checks of 15 TSI, two Radiance Research and two of the three Ecotech nephelometers were performed using measurements of filtered (i.e., particle free) air and filtered CO2. For the TSI nephelometers, flow rates for the zero air measurements were done with the internal blower or equivalent flow at a nominal 30 l/m; CO2 flow rate was approximately 5 l/m. The nephelometers were purged for 5 min before each measurement, followed by either a 10 min air measurement or a 5 min CO2 measurement. The measured average values of the six scattering coefficients (450, 550, and 700 nm for total scattering and hemispheric backscattering channels) were adjusted from instrument conditions of temperature and pressure to STP conditions (standard conditions are defined here as 1013.25 mb and 273.15 K) and compared with theoretical values. Calibration checks such as these determine whether the previous instrument calibration is still appropriate or whether something has changed in the interim. For the other nephelometers, similar calibration checks were performed. For the TSI nephelometers, as received at the start of WCCAP2007 the initial calibration check results deviated from CO2 theoretical values by –0.7% and 0.8%, average and standard deviation, respectively, with the exception of two instruments that required maintenance. The range was within ± 7%, consistent with the calibration results reported by CitationHeintzenberg et al. (2006). Subsequent to the initial check, the instruments were all cleaned and leak checked, their lamps and photomultiplier tubes checked and replaced if necessary, and the calibrations were optimized before the intercomparisons with atmospheric aerosol. Post-optimization calibration checks on the TSI nephelometers yielded a range of average deviations from theoretical values of within ± 3%. A similar evaluation of the other models was not possible given the limited number of units.
2.4. Mie Calculations for Generic and Size Distribution Specific Corrections
We followed the methods of CitationAnderson and Ogren (1998) and CitationBond et al. (2009) in which light scattering coefficients are calculated for both the Lambertian (true) and measured (neph) angular illumination functions, σ sp,true and σ sp,neph via Mie theory,
FIG. 2 Angular intensity function, f(θ), including forward and backward truncation, for the TSI 3563, Radiance Research M903 and Ecotech 9003 nephelometers at green wavelengths. For comparison purposes, each function has been normalized to the area under the reference sine curve over the truncation angle range of the TSI instrument, 7 to 170 degrees.
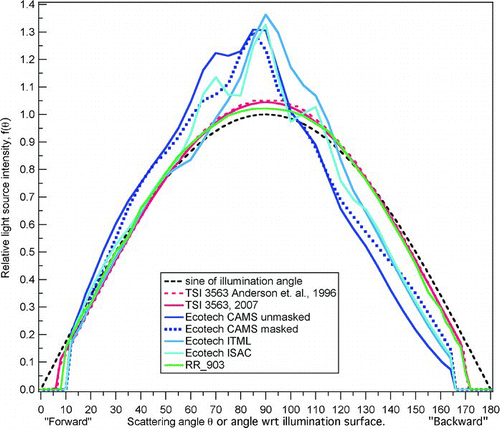
Subsequently, a generic and size dependent correction factor, C ts , was calculated for each of the nephelometers and wavelengths for the above parameter ranges via Equation (Equation2). Also, an Ångström exponent, å sp , was calculated from the three wavelength data of the TSI nephelometers via Equation (Equation3).
3. RESULTS AND DISCUSSION
3.1. Illumination Function
The illumination intensity functions with respect to angle from the plane of the light source, f(θ), are shown in for the three models of nephelometer that were tested in the workshop. For comparison, the ideal, Lambertian, or sine function and the TSI light source function reported by CitationAnderson et al. (1996) are included. The area of each of the measured functions was normalized to the sine function over the integration range 7 to 170 degrees to match the angular truncation of the TSI nephelometer for comparison. For the TSI model 3563, the WCCAP2007 result is slightly different than that of CitationAnderson et al. (1996). The inter-instrument variability and the standard deviation of repetitive measurements on one TSI light source (not shown) were less than 1%, within the width of the plotted line. The percent deviation from ideal is within ± 5% in the angular range 20 to 160 degrees but decreases to −20% close to the truncation angle limits. The illumination functions at the other TSI wavelengths, λ 450 and 700 nm, were measured and were identical to that at 550 nm in comparison to the sine function but are not shown.
The illumination function for the Radiance Research model M903 light source is relatively symmetric and close to the TSI function and the ideal sine function. Between 35 and 125 degrees the Radiance Research function has a positive deviation of about 3%; in the forward direction it is very close to sine up to the truncation limit; it deviates increasingly negatively with angle to –30% at the backward truncation angle of 170 degrees.
The illumination functions of the light sources from the three Ecotech nephelometers, shown separately, deviate from the sine function much more than those of the TSI or Radiance Research nephelometers. Due to the discrete nature of the multiple LED sources and the relatively transparent, ground-glass diffuser plate there is significant structure in the angular distribution of the light and a relatively large inter-instrument variability. The function is not symmetric and there is a significant positive deviation from sine over the angular range 15 to 100 degrees and a negative deviation over the backward scattering range. Because of the length of the Ecotech 9003 light source the entire diffuser plate could not reasonably be included in the field of view of the goniometer but the area of most intense illumination by the LEDs was included. To test the effect of this inaccuracy, the diffuser plate was masked to the field of view of the goniometer and measured separately as shown in the figure. The resulting, masked illumination function was different but not significantly so, i.e., it was not outside the range of the unit to unit variability of the Ecotech nephelometers that were tested.
3.2. Corrections to Account for Non-Ideal Illumination and Truncation
3.2.1. Generic Correction
C ts was calculated as described earlier from the measured angular illumination functions shown above and plotted vs. D gv in with the same format as in CitationAnderson and Ogren (1998) for green wavelengths, a log normal size distribution, σ g = 1.8 and m = 1.46 + 0.0i and truncation limits from . This generic correction shows the small effect of the new illumination function on the correction for the TSI nephelometer and presents the previously undetermined correction factors for the Radiance Research M903 and Ecotech 9003 nephelometers.
FIG. 3 Calculated generic correction factor, C ts , vs. D gv for three models of nephelometers accounting for truncation and angular illumination function of the light sources. The result of CitationAnderson and Ogren (1998) from the previous illumination function for the same aerosol parameters is shown for comparison.
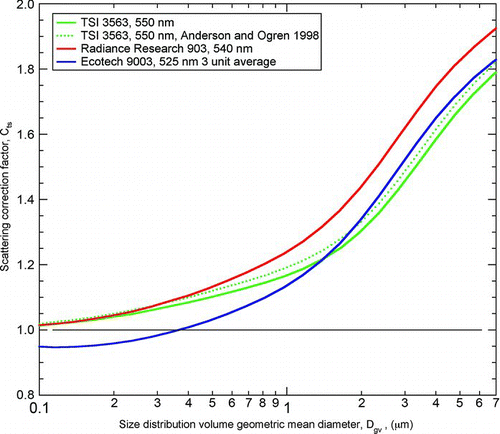
For the TSI and Radiance Research nephelometers, the correction factors, C ts , are near 1.0 at the smallest particle size, increase to 1.16 and 1.23, respectively, at a D gv of 1 μ m and increase monotonically to larger values in the super-micrometric range. The sense of the newly measured illumination function for the TSI nephelometers was to reduce the correction factor only minimally from 1.07 to 1.06 at a D gv of 0.3 μ m. The Radiance Research nephelometer has a slightly larger correction that is likely due to its larger forward truncation angle of 10° cf. the TSI truncation angle of 7°. The Ecotech nephelometer has a correction of 0.95 at D gv of 0.2 μ m and a negligible correction at 0.3 to 0.4 μ m due to a fortuitous interaction of its truncation and non-ideal angular illumination. The illumination intensity in the forward scattering angular range from 20 to 90 degrees is greater than the ideal sine function and compensates to a large extent for the lack of intensity between 0 and 12 degrees. As with the other two instruments the correction increases at larger D gv when the Ecotech nephelometer's forward truncation and insensitivity to forward scattering dominates.
3.2.2. Size, Ångström Exponent, Dependent Correction
As previous analyses and the above plots show, the correction is strongly size-dependent. Furthermore, previous analysis has shown that the Ångström exponent is also size dependent and that these two size-dependent parameters, C ts and å sp , are correlated to some extent over certain size ranges such that a self-determined correction factor can be estimated if wavelength dependent scattering is measured as in the TSI 3563 nephelometer. As in CitationAnderson and Ogren (1998) and CitationBond (2009), we have plotted C ts vs. å sp in . For the TSI and Radiance Research nephelometers, C ts ranges from 1.02 to 1.11 for a range of Ångström exponent from 2.5 to 1.0, respectively. For the Ecotech, C ts ranges from 0.95 to 1.04 for the same range of Ångström exponent.
FIG. 4 Calculated generic correction factor, C ts , versus Ångström exponent for the TSI 3563, Radiance Research M903 and Ecotech 9003 nephelometers based on the WCCAP2007 angular illumination function. Results based on CitationAnderson and Ogren (1998) are shown for comparison. The horizontal bars show ranges of Ångström exponent from several regions of the globe as measured with a TSI three wavelength nephelometer (CitationAndrews 2004; CitationCarrico 2000; CitationDoherty 2005; CitationQuinn 2000; CitationSierau 2006).
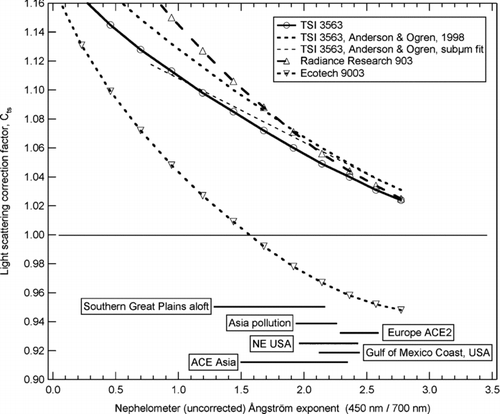
4. CONCLUSIONS
The precision of repeated CO2 calibration measurements at this workshop and in longer term practice among the several authors is, as stated, within 3% with a mean of 1.6% and std. dev. of 0.7%. We have no explanation for this other than to propose that it is a combination of reasonable experimental errors in the calibration and the subsequent calibration check procedures including signal noise, inconsistent purging of the sensing volume of aerosol prior to the particle free zero measurement, and of air prior to the CO2 span measurements, errors in internal temperature values due to gradients through the nephelometer.
The correction factor resulting from the non-ideal angular truncation and illumination intensity of the TSI nephelometer remains unchanged from previous estimates within the limits of experimental error and can be estimated by the empirical, Ångström exponent-based equation of CitationAnderson and Ogren (1998). The illumination function for the Radiance Research nephelometer is similar to that of the TSI but the correction factor is larger by a difference of 1 to 2% for submicrometric aerosol and 8 to 15% for aerosol in the coarse mode range. The Ecotech nephelometer illumination function deviates significantly from the sine function and has a correction factor of 0.95 to 1.04 depending on the size distribution of the aerosol measured. The unit to unit variability of the correction factor for the Ecotech nephelometer is of similar magnitude and without specific measurement of the particular instrument's intensity function this must be taken as an uncertainty in the measurement.
As a consequence of these results, Ecotech's Aurora model nephelometer light source has been updated to produce an angular intensity distribution function that is closer to Lambertian and more reproducible. This light source has an increased number of LEDs and a shorter axial dimension. Ecotech is currently further developing the light source to feature a diffusion plate similar to the TSI 3563.
The correction factor for the TSI instrument can be estimated by the Ångström exponent measured internally as the wavelength dependence of scattering coefficient but the correction factor for the other units must be estimated based on some knowledge of the aerosol size distribution. As explained by CitationBond et al. (2009) the best correction factor can be obtained by application of a measured size distribution and refractive index via Mie calculations and in the case of strongly absorbing aerosol, inclusion of the complex refractive index.
The truncation errors for these nephelometers are significant, and therefore should be accounted for when reporting aerosol light scattering coefficients. They are of similar magnitude to the calibration errors reported in section 2.3. By comparison, the error in the measured light scattering coefficient cf. the ambient or so-called dry scattering coefficient due to variation in hygroscopic growth with chemical composition of the aerosol and an uncertain or uncontrolled relative humidity in the inlet to the nephelometer and in its sensing volume can easily be much larger than the truncation, illumination error (e.g., 10% or more). The errors due to inlet losses of large particles or a poorly defined impactor- or cyclone-based size cut can also be large.
Acknowledgments
The nephelometer workshop at the WMO-GAW World Calibration Centre for Aerosol Physics, Leipzig, Germany, was conducted under the auspices of and with support from the EU-projects Atmospheric Composition Change: The European Network of Excellence, ACCENT (GOCE-CT-2004-505337), European Integrated Project on Aerosol Cloud Climate Air Quality Interactions, EUCAARI (036833-2), and European Supersites for Atmospheric Aerosol Research, EUSAAR (RII3-CT-2006-026140) as well by the German Federal Environment Agency (FKZ: 351 01 046). This work was also supported by the National Science Foundation's Atmospheric Chemistry Program under grant ATM-0337853 (DSC).
Notes
*The wavelength of the Radiance M903 nephelometer with a Corion CA 550S interference filter is given as 545 nm in the manufacturer's manual. The value of 540 nm in is from a measurement of the combined effective wavelength of light source, filter, and photomultiplier detector (CitationAnderson et al. 2003).
REFERENCES
- Anderson , T. L. , Covert , D. S. , Marshall , S. F. , Laucks , M. L. , Charlson , R. J. , Waggoner , A. P. , Ogren , J. A. , Caldow , R. , Holm , R. L. , Quant , F. R. , Sem , G. J. , Wiedensohler , A. , Ahlquist , N. A. and Bates , T. S. 1996 . Performance Characteristics of a High-Sensitivity, Three-Wavelength, Total Scatter/Backscatter Nephelometer . J. Atmos. Ocean. Tech. , 13 : 967 – 986 .
- Anderson , T. L. and Ogren , J. A. 1998 . Determining Aerosol Radiative Properties Using the TSI 3563 Integrating Nephelometer . Aerosol. Sci. Technol. , 29 : 57 – 69 .
- Anderson , T. L. , Masonis , S. J. , Covert , D. S. , Ahlquist , N. C. , Howell , S. G. , Clarke , A. D. and McNaughton , C. S. 2003 . Variability of Aerosol Optical Properties Derived From In Situ Aircraft Measurements During ACE-Asia . J. Geophys. Res. , 108 ( D23 ) : 8647 doi:10.1029/2002JD003247
- Andrews , E. , Sheridan , P. J. , Ogren , J. A. and Ferrare , R. 2004 . In Situ Aerosol Profiles Over the Southern Great Plains Cloud and Radiation Test Bed Site: 1. Aerosol Optical Properties . J. Geophys. Res. , 109 D06208, doi:10.1029/2003JD004025
- Beuttell , R. G. and Brewer , A. W. 1949 . Instruments for the Measurement of the Visual Range . J. Sci. Instrum. , 26 : 357 – 359 .
- Bond , T. C. , Covert , D. S. and Müller , T. 2009 . Truncation and Angular-Scattering Corrections for Absorbing Aerosol in the TSI 3563 Nephelometer . Aerosol Sci. Technol. , Submitted Feb. 2009
- Carrico , C. M. , Rood , M. J. , Ogren , J. A. , Neusuess , C. , Wiedensohler , A. and Heintzenberg , J. 2000 . Aerosol Optical Properties at Sagres, Portugal During ACE-2 . Tellus , 52B : 694 – 715 .
- Doherty , S. J. , Quinn , P. K. , Jefferson , A. , Carrico , C. M. , Anderson , T. L. and Hegg , D. 2005 . A Comparison and Summary of Aerosol Optical Properties as Observed In Situ From Aircraft, Ship, and Land During ACE-Asia . J. Geophys. Res. , 110 D04201, doi:10.1029/2004JD004964
- Heintzenberg , J. and Charlson , R. J. 1996 . Design and Applications of the Integrating Nephelometer: A Review . J. Atmos. Ocean. Tech. , 13 : 987 – 1000 .
- Heintzenberg , J. , Wiedensohler , A. , Tuch , T. M. , Covert , D. S. , Sheridan , P. , Ogren , J. A. , Gras , J. , Nessler , R. , Kleefeld , C. , Kalivitis , N. , Aaltonen , V. , Wilhelm , R.-T. and Havlicek , M. 2006 . Intercomparisons and Aerosol Calibrations of 12 Commercial Integrating Nephelometers of Three Manufacturers . J. Atmos. Ocean. Tech. , 23 : 902 – 914 .
- Moosmüller , H. and Arnott , W. P. 2003 . Angular Truncation Errors in Integrating Nephelometry . Rev. Sci. Instrum. , 74 : 3492 – 3501 .
- Quinn , P. K. , Bates , T. S. , Coffman , D. J. , Miller , T. L. , Johnson , J. E. , Covert , D. S. , Putaud , J.-P. , Neusuess , C. and Novakov , T. 2000 . A Comparison of Aerosol Chemical and Optical Properties From the 1st and 2nd Aerosol Characterization Experiments . Tellus , 52B : 239 – 257 .
- Sierau , B. , Covert , D. S. , Coffman , D. J. , Quinn , P. K. and Bates , T. S. 2006 . Aerosol Optical Properties during the 2004 New England Air Quality Study—Intercontinental Transport and Chemical Transformation: Gulf of Maine Surface Measurements, Regional and Case Studies . J. Geophys. Res. , 111 : D23S37 doi: 10.129/2006JD007568