Abstract
This study was conducted to evaluate the effect of aerosol generation, relative humidity, and method of sampling on the culturability of the vegetative bacteria Pantoea agglomerans (P. agglomerans) formerly known as Erwinia herbicola. This research has relevance both for the use of this organism as a biowarfare simulant and for bioaerosol exposure assessment and public health. The culturability of P. agglomerans was tested using a test chamber against two generating systems (Collison and Bubble nebulizers), two sampling systems (the all-glass impinger (AGI-30), and the BioSampler), three collection media (water, TSB, and PBS) and across a range of humidities. Results indicated that the Bubble nebulizer was 15% more efficient in generating viable P. agglomerans counts (p ≤ 0.05). No difference was observed in overall efficiency between sampling methods (p > 0.05). However, as a collection media, PBS was observed to yield higher (p ≤ 0.01) viable counts compared to sterile deionized water. Relative humidity was found to strongly influence airborne P. agglomerans culturability. Culturable P. agglomerans was below the limit of detection for RH < 15% and then increased in a log-linear fashion to humidities of 75%. This research will help identify optimal means for evaluation of environmentally sensitive airborne bacteria for purposes of exposure assessment and public health as well as homeland security.
INTRODUCTION
Simulants are organisms ubiquitous in the environment that are used in laboratory testing to avoid the use of the more hazardous and expensive biological agents. These organisms must display some of the key airborne characteristics of biological toxicants such as aerodynamic size, viability, and tolerance to adverse environmental conditions, without being pathogenic (CitationForney et al. 1997).
Currently there are four biological simulants used by the US Army as models for evaluating sampling and analysis techniques: Bacillus globigii (Bg), a gram positive, spore-forming bacterium that simulates Bacillus anthracis and other hardy bacteria; MS2 coliphage, a small RNA virus used to simulate viral organisms such as the variola virus that causes smallpox; ovalbumin, an egg-white protein that simulates proteinaceous toxins of small molecular weight such as Ricin; and Pantoea agglomerans, a gram negative non-spore forming bacteria that simulates fragile vegetative bacteria such as Yersinia pestis, which is the source of the plague.
The simulant P. agglomerans (ATCC-33243, formerly Erwinia herbicola), was the focus of this research. Previous studies (CitationForney et al. 1997; CitationWinters et al. 1997) reported P. agglomerans to have poor survival characteristics when sampled using the traditional methods of impingement into liquid or collecting onto dry filters. Survivability has been assessed by culturing to obtain conventional colony forming units (cfu) per volume of air, and compared to results obtained by ELISA (CitationForney et al. 1997). Losses of 30 to 70% were reported after sampling with an impinger and 90 to 99% after collecting on a filter (CitationForney et al. 1997). Possible sources of the reported losses were attributed to the aerosolization process, sensitivity to low humidity once airborne, and to the sampling process (CitationForney et al. 1997).
Previous studies evaluating P. agglomerans survivability relied on the Collision® nebulizer (BGI Inc., Waltham, MA) to generate aerosolized P. agglomerans stocks (CitationForney et al. 1997; CitationWinters et al. 1997). This nebulizer is the instrument of choice and the gold standard for laboratory bioaerosol generation (CitationMainelis et al. 2002; CitationAgranovski et al. 2002; CitationUlevicius et al. 1997; CitationStone and Johnson 2002; CitationHeidelberg et al. 1997). One downside of the use of this nebulizer (CitationGussman 1984) is that whole classes of generators are called “Collison nebulizer,” each with varying dimensions that alter the mass median diameter (MMD) and geometric standard deviation (σd) of the aerosol output, making inter-experimental comparison difficult. Furthermore, CitationUlevicius et al. (1997) hypothesized that the sheer stress of the Collison nebulizer is the cause of bacterial viability losses in the nebulization process. To minimize these losses, their group designed an alternate generation mechanism called the “Bubble generator” reported to be gentler on bacteria, as it relies on a bubbling mechanism to aerosolize bacteria at low pressures.
Survival of aerosolized vegetative bacteria similar to P. agglomerans have been reported to decrease with decreasing relative humidity (RH) (CitationTheunissen et al. 1993; CitationStone and Johnson 2002), with losses of up to 90% when testing at RH lower that 50% (CitationStewart et al. 1997; CitationLin and Li 1999). One study using a genetically engineered P. agglomerans (an antibiotic resistant strain of Erwinia Herbicola) (CitationMarthi et al. 1990) found this bacteria survives better at low RH compared to three other similarly engineered vegetative gram-negative bacteria. No other studies were found on survival of P. agglomerans at low RH.
Multiple bioaerosol sampling techniques are currently available, (CitationMuilenberg 2003) each with specific advantages. Of these, the AGI-30 all-glass impinger (Ace Glass®, Little Rock, AR) has been suggested as one of the samplers of choice for the collection of viable microorganisms by the International Aerobiology Symposium (CitationHenningson and Ahlberg 1994; CitationJensen et al. 1992) and the ACGIH (1986). However, this commonly used method for sampling biological aerosols has been reported to impart significant stress on sensitive microorganisms, resulting in viability losses (CitationStewart et al. 1995; CitationTerzieva et al. 1996) as well as losses to re-aerosolization (CitationMainelis et al. 2002; CitationLin et al. 2000). In order to minimize these losses, an alternative sampler was developed by Willeke et al, known as the BioSampler, (SKC Inc, Eighty Four, Pa) which combines impingement and centrifugal motions (CitationWilleke et al. 1998; CitationLin et al. 2000). This sampler has been reported to increase survival rate of sampled bioaerosols by minimizing shear forces and evaporation of the collection fluid.
Accordingly, in order to better understand how measurements of the sensitive test bioaerosol P. agglomerans relate to actual airborne concentrations, we examined methodological influences of generation, sampling, and humidity on P. agglomerans culturability. This work has relevance to exposure assessment, public health, and biodefense research.
MATERIALS AND METHODS
For this project, the Collison nebulizer was used as a reference method of aerosol generation. The bubble nebulizer described in the literature (CitationUlevicius et al. 1997) was replicated in our lab as a means to increase viability when generating bioaerosols, and was used as the test method. Culturability losses due to low RH were tested in our study by modifying the relative humidity inside the chamber during sampling. Finally, the BioSampler was used in parallel to an AGI-30 impinger (our reference method) so that overall efficiency (including biological and physical efficiency) of the samplers, could be evaluated. Both samplers were also tested with different collection fluids to investigate optimum culturability. Viability was assessed with the plate-count method.
General Setup
All tests were conducted inside a chemical fume-hood, where a 125 L chamber was connected to the generation, sampling and auxiliary ports as shown in . The generators described below were connected (one at a time) to the house air through a drying cylinder filled with dessicant (23001, Drierite Co. LTD, Xenia, OH), and a HEPA filter in order to condition the house air for later adjustment of the relative humidity, and to achieve low background particle concentrations in the chamber.
FIG. 1 Laboratory setup of the generation-to-sampling train; APS = aerodynamic particle sizer; T = temperature; RH = relative humidity.
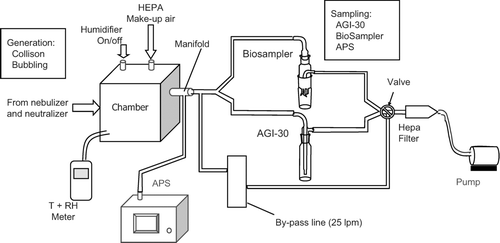
A Collison nebulizer (CN-8, BGI Inc., Waltham, MA) was used as the reference generator. Filtered and dried air was supplied at a generating pressure (Pg) of 68.9 kilopascal (KPa) to the inlet port, where air pressure for aerosol generation was monitored with a pressure gauge. The filtered and dried air was diverted in order to supply 10 lpm of additional air for drying purposes (Qdry).
A bubble Nebulizer described by CitationUlevicius et al (1997), was replicated in our lab, except with a smaller volume container in order to minimize the amount of bacterial solution required in each experiment. The lid of a 250 ml Nalgene wide-mouth jar (Cat #2118-0008) was drilled to fit the air dispersion unit (Corning 39525-30C), two glass tubes for dry-air supply to enhance evaporation of the liquid from the forming droplets (made from modified Pasteur pipettes (VWR 1462-200)) and an exit port (rounded brass tube fitting). The air dispersion unit consists of a glass tube with a fritted-glass disk at 90°, which is submerged in the liquid (). Each port was made airtight either with silicone or a bored rubber stopper. As described in the design paper (CitationUlevicius et al. 1997), Qdry was set to 15 liter per minute (lpm) and Qbubl to 1.5 lpm. The minimum amount of liquid necessary to cover the air dispersion unit is 35 ml, and Hdry was set 2 mm above the liquid surface. Both Qdry and Qbubl were supplied from filtered and dried house air, and the flow-rate monitored and controlled with respective flow meters.
A two-milli-Curie Kr-85 charge neutralizer (Model 3012, TSI., St. Paul, MN) was connected between the nebulizer and the chamber, in order to minimize losses to the tubing and chamber due to electrostatic forces. The chamber consisted of a 125 liter acrylic cube, with a port for easy access to the inside of the chamber for periodic cleaning, and several access ports for connection of the test equipment, sampling devices, and conditioning of chamber air.
The sampling stage consists of a sampling manifold, the two samplers to be tested (an AGI-30® and a BioSampler®), an equilibrating by-pass line, and an Aerodynamic Particle Sizer (APS) (Model 3310, TSI Inc., St Paul, MN). A manifold was designed so that the total sampling flow (30 Lpm) was properly balanced between the APS (5 Lpm) and the two samplers (12.5 Lpm each). This way, equal velocity samples of the aerosol inside the chamber enter the inlets of the test samplers and the APS (Hinds 1982). The sampling manifold consists of three concentric stainless steel cylinders with connecting ports for the respective sampler flow rates of 5 lpm, 12.5 lpm, and 25 lpm.
The two bioaerosol samplers were plumbed in parallel into the test apparatus through the 25 lpm port of the sampling manifold at 12.5 lpm each. The 12.5 lpm port was not used during these experiments and was closed, and the 5 lpm port was connected to the APS. The AGI-30®, which has been described elsewhere (CitationLin et al. 1997), is an all glass instrument with a curved inlet that has a 50% cut-size of 0.3 μ m (Nevalainen et al. 1992). The BioSampler® is also an all glass instrument, similar in concept and shape to the impinger, but with three angled nozzles, causing impingement and centrifugal motions (CitationWilleke et al. 1998; CitationLin et al. 2000). It has been shown to collect particles of aerodynamic diameter > 0.3 μ m, similar to the AGI-30 (CitationHogan et al. 2005)
In order to reach a steady state inside the chamber before sampling, a by-pass valve and line were plumbed using a modified impinger () that operates at 25 lpm, equal to the flow of the dual sampling units. This by-pass line was operated during the first minutes of nebulization, until the APS output reached a steady state. When steady state was observed, the valve was switched to direct the chamber aerosol to the AGI-30 and BioSampler.
Aerosol concentration inside the chamber was monitored with a 3310 APS. Chamber conditioning was achieved through two ports in addition to the sampling and generation ports. One of the ports was connected to a low resistance HEPA filter (12144 HEPA Capsule, Pall Life Sciences, East Hills, NY) used to minimize pressure differences and supply the chamber with make-up air—needed to prevent the chamber from collapsing in the event of a demand of air greater than the supply by the nebulizer port. Another port was used for humidification of the chamber.
Relative humidity inside the chamber was modified with an ultrasonic nebulizer (model 100HD, DeVilbiss® Co. HCW, Somerset, PA). The nebulizer's output was connected directly to the chamber and manually controlled with a two-way valve in order to increase relative humidity inside the chamber up to 75% (). Temperature and relative humidity were monitored using a Digital Thermo-hygrometer (Control Company, China).
Test Procedures and Analysis
The influences of generation, sampling, and humidity on P. agglomerans were evaluated using the two test nebulizers and two samplers described in the previous section. For each generation method, the aerosol was sampled simultaneously using both AGI-30 and BioSampler. The optimal generation method was first evaluated based on the fraction of culturable to total cells in the sampled aerosol, when analyzed by nebulizer. Because this test was not conclusive, the fractional change in culturable cells within the respective generation vessels was compared from before to after a 10 min run. The nebulizer that resulted in highest fraction of culturable P. agglomerans was selected. This generation method was used to test for the most efficient sampling method and collection fluid. Finally, the influence of humidity was examined using the optimal generation and sampling methods.
Prior to the start of experiments with P. agglomerans, tests were performed by aerosolizing polystyrene latex (PSL) beads of different and known sizes (0.8 μ m, 1.07 μ m, 2.1 μ m, 2.9 μ m, and 4.5 μ m). These tests were conducted to validate the use of the APS as a direct measure of what enters the samplers, as well as to verify APS calibration. The concentration inside the chamber was monitored with the APS, while sampling from the chamber with the AGI-30 (our reference sampler). Samples obtained with the impinger were analyzed under a microscope using a hemocytometer (Houser Scientific Co., Horsham, PA) and compared to the counts obtained using the APS.
P. agglomerans was used for all other tests in this study as described by CitationForney et al. (1997). The original stock, suspended in Tripticase Soy Broth (TSB) (Becton Dickinson, Cockeysville, MD) had an initial titer of 109 cells/mlliq. This initial stock was stored at 4°C and new working stocks were generated whenever additional suspension was needed for the experiments. The original stock and growth curve were obtained from Edgewood Chemical Biological Center (Aberdeen, MD). When material was needed for the aerosol trials, 0.6 ml of the original stock suspension were inoculated into 50 ml TSB and grown at 28°C shaking at 200 rpm for 16 h (CitationForney et al. 1997). Cultures were harvested by centrifugation at 5000 × g for 30 min at 4°C. The pellet was washed three times by resuspension/centrifugation using sterile/de-ionized water (sdH2O), its optical density was measured with a spectrophotometer (DR 4000U Hach Co., Loveland, CO) and stored at 2–8°C for up to 14 days (CitationForney et al. 1997). The harvested and washed P. agglomerans solution was taken out of the refrigerator 30 min prior to start of nebulization in order to equilibrate to room temperature. The effect of storage on viability of P. agglomerans and the shape of bioaerosol size distribution were examined.
In order to identify any possible contamination with particles that might affect the APS or culturability results, and to account for background, nebulizers were operated 5 min with air and 5 min with sterile DI water before each experiment, to obtain a “dry background” and a “H2O background” of the particle concentration in the chamber. The APS was used to measure background levels, which were later subtracted from results of P. agglomerans nebulization. When air or water nebulization backgrounds were above 2 particles/cm3 as measured by the APS, the system was inspected for leaks and the tubing and chamber were cleaned.
Aerosolization of P. agglomerans from sdH2O was achieved with either the Collison or the Bubble nebulizer into the chamber. Before nebulization, an aliquot was separated for culturability analysis. In order to achieve a steady state inside the chamber, the 25 lpm by-pass line was turned on before the nebulizer. Once the nebulizer was turned on, the aerosol was allowed to mix and equilibrate inside the chamber before beginning of the 5–10 min sampling, for a total generation time of 10–15 min. After nebulization, an aliquot of the fluid from the nebulizer was separated for culturability analysis. Concentration inside the chamber was monitored with the APS for dynamic equilibrium with a 20 sec. sample every minute. Relative humidity control was achieved manually, by turning the nebulizer on and off as RH drifted within 5% of the desired value.
The air samplers were evaluated side-by-side, connected via the isokinetic manifold to the chamber (). The sampling strategy was decided after a series of initial runs to determine when steady state in the chamber had been achieved. Both the AGI-30 and BioSampler were filled with 20 ml of collection fluid (either sdH2O, TSB, or PBS) and ran simultaneously. Sampling began after concentration inside the chamber had reached a steady state as indicated by APS particle counts. Samples were collected over 5 min intervals.
In order to asses changes in culturability due to the generation process, the aliquots separated before and after nebulization from the solution in the nebulizers were plated following air sampling, along with the fluid from the samplers and the reference and quality control blanks. Each solution was serially diluted 10-fold and three dilutions were plated on duplicate trypticase soy agar (TSA) plates and incubated at 28°C as recommended by CitationForney et al. (1997). Plates containing 30 to 300 colony-forming units (cfu) were counted after 18 to 24 h. Water, TSB, and PBS were evaluated as collection media for both the AGI-30 and BioSampler.
Results from the cultured impinger and BioSampler fluids in colony forming units per ml of liquid plated were adjusted for volume of air passed through the samplers and final volume of liquid in the vessel to obtain culturability per volume of air, N cfu (in cfu/Lair) following Equation (Equation1).
N cfu | = |
colony forming units per L of air |
cfu/mLliq | = |
colony forming units per mL of liquid |
Vf | = |
final liquid volume in the impinger (in mLliquid) |
Qsamp | = |
sample flow rate (in Lair/min) |
Time | = |
sampling interval (in minutes) |
The resulting units for CE are: [culturable/total]. This is technically a unitless parameter, since both cfu and counts are measures of numbers of organisms, and reflects overall efficiency of the method, which includes physical efficiencies (inlet, transmission, collection, retention) and biological efficiency (CitationKesavan and Stuebing 2009).
Statistical Analysis
A Spearman's rank correlation coefficient (rs) was used for initial validation of the APS counts due to small sample size. Initial exploratory tests revealed a log-normal distribution of CE. Thus, data were log-transformed for parametric statistical analysis. The Shapiro-Wilk test for normality was used to confirm successful transformation. The differences between generation and sampling methods, and between collection media were investigated using t-tests. The relationship between RH and CE was evaluated using non-linear regression analysis. Finally, CE data were analyzed in a multiple logistic regression model where collection media, relative humidity, nebulizer, and sampler were included in the model, as well as interaction effects between the different variables. Statistical analysis was performed using STATA, and a p-value of ≤0.05 was considered significant.
RESULTS
Because the following experiments rely on APS counts to normalize sampler results, an initial validation test was performed. The APS counts of aerosolized PSL beads of 0.8 μ m, 1.07 μ m, 2.1 μ m, 2.9 μ m, and 4.5 μ m showed a good correlation (R2 of 0.95) with tallies obtained from the impinger's fluid counted with a hemocytometer ().
FIG. 3 Correlation of APS counts with optical (hemacytometer) counts. PSL beads of 0.8 μ m, 1.07 μ m, 2.1 μ m, 2.9 μ m, and 4.5 μ m were counted.
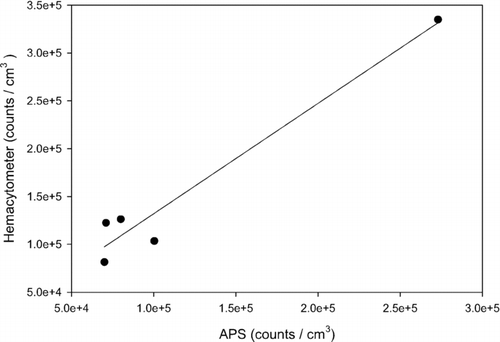
Water and air backgrounds monitored with the APS, were kept below 2 particles/cm3. Water backgrounds ranged between 0.04% and 1.5% of the peak produced when nebulizing P. agglomerans (). Air backgrounds ranged between 0.02% and 1.5% of the P. agglomerans peak
Effect of storage on viability and the shape of bioaerosol size distribution were also examined. No correlation was found between days in storage and culturability (cfu/mlliq) up to 14 days (R2 = 0.0095). Furthermore, no significant difference (p > 0.05) was found in the distributions of number median diameter and geometric standard deviations recorded with the APS between the 1st and the 14th days. The typical size distribution extended from 0.5 to 1.03 μ m. with a peak at 0.73 μ m ().
In order to evaluate the influence of nebulizer operation on P. agglomerans culturability losses, the numbers of cfu in the nebulizer liquid from before to after the 10 min operation were compared (). Data was adjusted to liquid loss, to account for evaporation during operation. The log-transformed distributions were compared using a t-test. Whereas no before-to-after difference in culturable counts was observed for the Bubble nebulizer (p > 0.05), a loss of 15% was observed for the median Collison nebulizer data (p < 0.05).
FIG. 5 Culturability of the nebulizer fluid before and after nebulization, plotted by nebulizer. N = 28. The box extends from the 25th to the 75th percentile. The line across the box represents the median value. The whiskers extend to the most extreme observations that are within 1.5 times the percentiles. Points outside this range represent outliers. * denotes statistical significance.
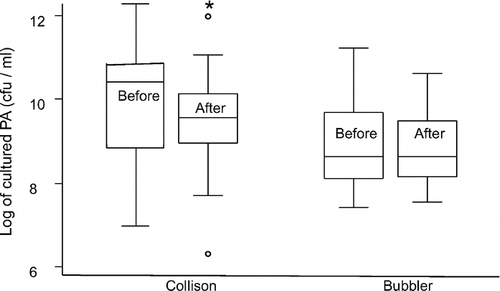
In order to assess sampling efficiency based on aerosolized bacteria, CE results from both BioSampler and AGI-30 (from the sampler fluids) were analyzed according to the nebulizer they were generated with (). When comparing median values, the bubble nebulizer yielded 22% higher CE than the Collison nebulizer (p = 0.09).
FIG. 6 Culturability efficiency (CE). Pooled data (n = 29) from aerosolized bacteria sampled with BioSampler and AGI-30, plotted by generator (Log scale).
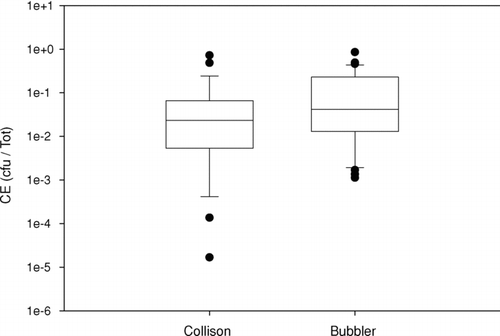
Analyzing exclusively data obtained with the bubble nebulizer, the BioSampler was on average 20% more efficient in capturing and retaining airborne P. agglomerans relative to the AGI-30; however, this difference was not statistically significant (p = 0.31) between the median CE of the two samplers ().
FIG. 7 Culturability efficiency (CE) for the BioSampler and AGI-30 impinger (n = 16). Data obtained using the bubble nebulizer and plotted by sampler (Log scale).
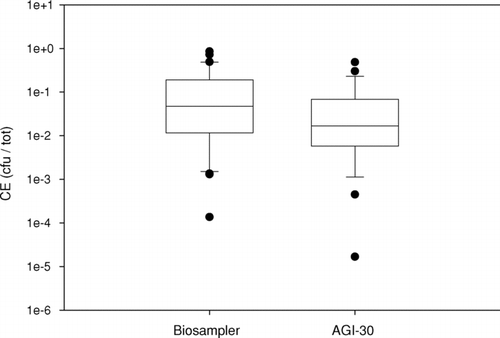
Based on data from the BioSampler, PBS collection media was observed to better preserve the ability of microorganisms to maintain their viability. We observed a 10% increase in median survivability of P. agglomerans relative to distilled water (p < 0.01) (). TSB was eliminated as a collection medium due to excessive foaming in the impinger caused by bubbling action during sample collection.
FIG. 8 Culturability efficiency (CE) data (n = 26 for water; n = 47 for PBS) plotted by collection media.
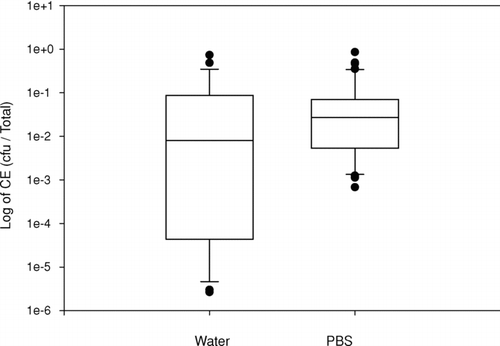
The CE of airborne P. agglomerans was observed to be strongly influenced by chamber humidity (). Below chamber humidity of 15%, no detectable culturable P. agglomerans was observed. At RH above 20%, the log of CE increased in a linear fashion (R2 = 0.37; p < 0.01) with RH, as described in Equation (Equation3) and .
FIG. 9 Culturability efficiency as a function of relative humidity; (a) values at RH < 20% and (b) values at RH > 20%.
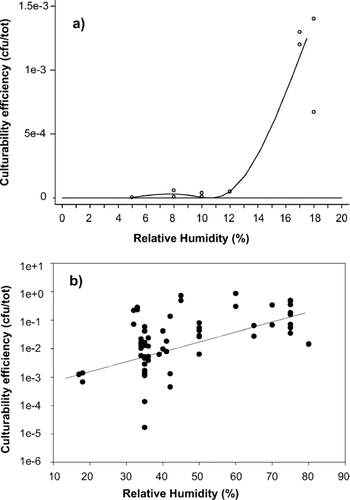
CE data were analyzed in a multiple logistic regression model where collection media and relative humidity were significant (p < 0.05) and neither nebulizer nor sampler were significant (p > 0.05). These results are consistent with the unadjusted results described above, and tests for interactions were all non-significant. The model is described by Equation (Equation4):
DISCUSSION AND CONCLUSIONS
The present study examined the effect of a combination of aerosol generation and sampling techniques as well as relative humidity, on the survivability of the bio-warfare simulant P. agglomerans. Two generation methods were examined: the bubbler and Collison® nebulizers. Based on culturability of the nebulizer fluid (cfu/mlliquid), we observed a 15% decrease (p = 0.01) in survivability associated with the Collison after 10 minutes. These results are consistent with those reported by CitationReponen et al (1997) who after 30 min observed greater than 40% metabolic injury with the Collison compared to < 1% with the bubbler. However, when nebulizers were compared analyzing culturability efficiency from the sampler fluids this difference was not observed (p > 0.05), although the geometric mean and interquartile range were slightly higher for the bubble nebulizer.
Previous studies (CitationForney et al. 1997) had reported storage stability of P. agglomerans up to 14 days. We confirmed this finding, and found no significant difference in the distributions of aerosolized P. agglomerans up to 14 days.
Overall sampling efficiency of aerosolized P. agglomerans was evaluated for two samplers: the traditional AGI-30 impinger and the more recently developed BioSampler®, designed to increase bioaerosol viability (CitationWilleke et al. 1998). Although efficiency of the BioSampler was slightly higher (5%) and its interquartile range reduced (10%) when compared to the AGI-30, the difference did not achieve statistical significance (p = 0.31). The sample size of 35 was adequate for detecting a 10% difference. This result is consistent with CitationLin et al. (2000) who reported non-statistical differences at sampling times up to 30 min with vegetative cells of P. fluorescens.
In order to test the influence of media used on survivability, three different collection media were tested: TSB, sdH2O, and PBS. TSB was eliminated as a collection media due to excessive foaming in the impinger caused by bubbling action during sample collection. Foaming was only minimal with the BioSampler, and this could present a clear advantage for this sampler since TSB would minimize osmotic stresses and provide ideal conditions for cell culturability (CitationLin et al. 2000). The efficiency of capturing culturable P. agglomerans was increased 10% for PBS compared with sdH2O, and the variability in efficiency was greatly reduced (56% reduction in interquartile range) with the use of PBS. This finding supports the popular use of PBS as collection media (CitationLundholm 1982; CitationJuozaitis 1994; CitationThorne et al. 1992; Duschaine 2000). Although Lin and Li (1998) used sdH2O with anti-foam and wetting agents, no studies were found with a systematic evaluation of water as collection media compared to PBS. PBS is the preferred medium due to the reduction in osmotic shock the cells experience when impacting the isotonic environment. Mineral oil has been suggested as collection media in order to decrease evaporation (CitationLin et al. 1999, Citation2000), but our team found it impossible to perform microbiological analysis with this media. Further studies are needed to evaluate alternative media and additives to decrease evaporation and increase culturability efficiency.
Finally, relative humidity experiments were conducted to examine the effect of environmental conditions on P. agglomerans survivability. A wide range of RH values were tested (from 5 to 75%) with two important observations. The first is that below 15% culturability is below the limit of detection. Few studies have evaluated bioaerosol recovery efficiency at such low humidities (CitationTheunissen et al. 1993; CitationCox and Wathes 1995) and our results are in agreement with their findings of a 3 order of magnitude reduction within the first 5 s of aerosolization at 5% RH. This finding has important implications for the possible use of P. agglomerans as a simulant under desertic conditions, limiting its use for outdoor dispersion. The second observation is that culturability efficiency above 15% increases with RH following a log-linear relationship. This last observation is supported by a study by CitationKo et al. (2000) which reported similar relationships, for spore-forming Serratia marcescens, and a wide body of literature that has identified a favorable effect of humidity on bacteria viability (CitationStone and Johnson 2002; CitationHeidelberg et al. 1997; CitationStewart et al. 1997; CitationLin and Li 1999).
We intend to build on the research reported here by considering alternate means for quantifying bioaerosols. Spreading an appropriate dilution of a liquid sample on solid medium for counting colony-forming units (CFU) is one of the most classical methods to quantify cell density. The advantages of cultivation are that it is technically easy to manage and inexpensive. However, colonies arise only from viable cells that are amenable to the growth on the media selected for the experiment.
With the development of fluorescent cell markers and advanced instrumentation technology such as flow cytometry, it has become possible to enumerate the total and viable number of microorganisms present in a sample over a wide range of input concentrations with a low degree of variability (CitationHewitt and Nebe-Von-Caron 2004). Since this method does not require that the target microorganism be directly examined or grown on culture medium, sampling time and volume constraints can be eliminated. Traditional microscopic methods can also be used to evaluate cell concentration and viability. Although this approach is relatively more accessible, it is also more time consuming and less accurate.
By directly comparing classical plate culturing with flow cytometry techniques for P. agglomerans we have been able to obtain a direct comparison between the total number of P. agglomerans in a sample (as determined by biomass counts) with the number of cultivable organisms enumerated by plating (Rule et al. 2007) but have yet to test this technique after aerosolization. The ratio of total and viable P. agglomerans in the stock solution prior to aerosolization and collection compared to the ratio obtained after collection will provide a mechanism to evaluate the loss of culturability of P. agglomerans during sample collection and processing.
Acknowledgments
This work was supported by the Aerosol Sciences Team at the US ARMY RDECOM Edgewood Chemical and Biological Center (Dr. Jerry Bottiger) under the auspices of the US Army Research Office Scientific Services Program, administered by Battelle. The authors acknowledge Agnes Akinyemi and Kristen Gibson for their expert assistance and support regarding microbiological laboratory techniques.
REFERENCES
- Agranovski , I. E. , Agranovski , V. , Reponen , T. , Willeke , K. and Grinshpun , S. A. 2002 . Development and Evaluation of a New Personal Sampler for Culturable Airborne Microorganisms . Atmos. Environ. , 36 : 889 – 898 .
- Cox , C. and Wathes , C. 1995 . Bioaerosols Handbook , Boca Raton, FL : Lewis Publishers, CRC Press .
- Forney , T. L. , Bell , E. C. and Bowdle , D. A. 1997 . Evaluation of Erwinia Herbicola as a surrogate biological warfare agent (BW) aerosol , Columbus, OH : Batelle memorial Institute .
- Gussman , R. A. 1984 . Note on the Particle size Output of Collison Nebulizers . American Industrial Hygiene Association Journal , 45 : B-8 – B-12 .
- Hewitt , C. J. and Nebe-Von-Caron , G. 2004 . The Application of Multiparameter Flow Cytometry to Monitor Individual Microbial Cell Physiological State . Adv Biochem Engin/Biotechnol. , 89 : 197 – 223 .
- Heidelberg , J. F. , Shahamat , M. , Levin , M. , Rahman , I. , Stelma , G. , Grim , C. and Colwell , R. R. 1997 . Effect of Aerosolization on Culturability and Viability of Gram-Negative Bacteria . Appl. Environ. Microbiol. , 63 : 3585 – 3588 .
- Henningson , E. W. and Ahlberg , M. S. 1994 . Evaluation of Microbiological Aerosol Samplers—A Review . J. Aerosol Sci. , 25 : 1459 – 1492 .
- Hogan , C. J. , Kettleson , E. M. , Lee , M.-H. , Ramaswami , B. , Angenent , L. T. and Biswas , P. 2005 . Sampling Methodologies and Dosage Assessment Techniques for Submicrometre and Ultrafine Virus Aerosol Particles . J. Appl. Microbiol. , 99 : 1422 – 1434 .
- Jensen , P. , Todd , W. , Davis , G. and Scarpino , P. 1992 . Evaluation of 8 Bioaerosol Samplers Challenged with Aerosols of Free Bacteria . Am. Ind. Hyg. Assoc. J. , 53 : 660 – 667 .
- Juozaitis , A. , Willeke , K. , Grinshpun , S. A. and Donnelly , J. 1994 . Impaction Onto a Glass Slide or Agar Versus Impingement into a Liquid for the Collection and Recovery of Airborne Microorganisms . Appl. Environ. Microbiol , 60 ( 3 ) : 861 – 870 . Mar
- Kesavan , J. and Stuebing , E. 2009 . “ Aerosol Sampling Efficiency Evaluation Methods at the US Army Edgewood Chemical Biological Center ” . In Environmental Monitoring , Edited by: Kim , Y. J. , Platt , U. , Gu , M. B. and Iwashi , H. New York : Springer .
- Ko , G. , First , M. W. and Burge , H. A. 2000 . Influence of Relative Humidity on Particle Size and UV Sensitivity of Serratia Marcescens and Mycobacterium Bovis BCG Aerosols . Tuber. Lung Dis , 80 ( 4–5 ) : 217 – 28 .
- Lin , W. H. and Li , C. S. 1999 . Evaluation of Impingement and Filtration Methods for Yeast Bioaerosol Sampling . Aerosol Sci. Technol. , 30 : 119 – 126 .
- Lin , X. J. , Willeke , K. , Ulevicius , V. and Grinshpun , S. A. 1997 . Effect of Sampling Time on the Collection Efficiency of All-Glass Impingers . Am. Ind. Hyg. Assoc. J. , 58 : 480 – 488 .
- Lin , X. J. , Reponen , T. , Willeke , K. , Wang , Z. , Grinshpun , S. A. and Trunov , M. 2000 . Survival of Airborne Microorganisms During Swirling Aerosol Collection . Aerosol Sci. Technol. , 32 : 184 – 196 .
- Lin , X. J. , Reponen , T. , Willeke , K. , Grinshpun , S. A. , Foarde , K. K. and Ensor , D. S. 1999 . Long-Term Sampling of Airborne Bacteria and Fungi into a Non-Evaporating Liquid . Atmos. Environ. , 33 : 4291 – 4298 .
- Lundholm , M. 1982 . Comparison of Methods for Quantitative Determinations of Airborne Bacteria end Evaluation of Total Viable Counts . Appl. Environ. Microbiol. , 44 ( 1 ) : 179 – 83 .
- Mainelis , G. , Gorny , R. L. , Reponen , T. , Trunov , M. , Grinshpun , S. A. , Baron , P. , Yadav , J. and Willeke , K. 2002 . Effect of Electrical Charges and Fields on Injury and Viability of Airborne Bacteria . Biotechnology and Bioengineering , 79 : 229 – 241 .
- Marthi , B. , Fieland , V. P. , Walter , M. and Seidler , R. J. 1990 . Survival of Bacteria During Aerosolization . Appl. Environ. Microbiol , 56 ( 11 ) : 3463 – 7 . Nov
- Muilenberg , M. L. 2003 . Sampling devices . Immunol Allergy Clin North Am Review , 23 ( 3 ) : 337 – 55 .
- Reponen , T. , Willeke , K. , Ulevicius , V. , Grinshpun , S. A. and Donnelly , J. 1997 . Techniques for Dispersion of Microorganisms into Air . Aerosol Sci. Technol. , 27 : 405 – 421 .
- Stewart , S. L. , Grinshpun , S. A. , Willeke , K. , Terzieva , S. , Ulevicius , V. and Donnelly , J. 1995 . Effect of Impact Stress on Microbial Recovery on an Agar Surface . Appl. Environ. Microbiol. , 61 : 1232 – 1239 .
- Stewart , I. W. , Leaver , G. and Futter , S. J. 1997 . The Enumeration of Aerosolised Saccharomyces Cerevisiae Using Bioluminescent Assay of Total Adenylates . J. Aerosol Sci. , 28 : 511 – 523 .
- Stone , R. C. and Johnson , D. L. 2002 . A Note on the Effect of Nebulization Time and Pressure on the Culturability of Bacillus subtilis and Pseudomonas Fluorescens . Aerosol Sci. Technol. , 36 : 536 – 539 .
- Terzieva , S. , Donnelly , J. , Ulevicius , V. , Grinshpun , S. A. , Willeke , K. , Stelma , G. N. and Brenner , K. P. 1996 . Comparison of Methods for Detection and Enumeration of Airborne Microorganisms Collected by Liquid Impingement . Appl. Environ. Microbiol. , 62 : 2264 – 2272 .
- Theunissen , H. J. , Lemmens-den Toom , N. A. , Burggraaf , A. , Stolz , E. and Michel , M. F. 1993 . Influence of Temperature and Relative Humidity on the Survival of Chlamydia Pneumoniae in Aerosols . Appl. Environ. Microbiol , 59 ( 8 ) : 2589 – 93 . Aug
- Thorne , P. S. , Kiekhaefer , M. S. , Whitten , P. and Donham , K. J. 1992 . Comparison of Bioaerosol Sampling Methods in Barns Housing Swine . Appl. Environ. Microbiol. , 58 : 2543 – 2551 .
- Ulevicius , V. , Willeke , K. , Grinshpun , S. A. , Donnelly , J. , Lin , X. J. and Mainelis , G. 1997 . Aerosolization of Particles from a Bubbling Liquid: Characteristics and Generator Development . Aerosol Sci. Technol. , 26 : 175 – 190 .
- Winters , D. R. , Martin , D. D. , Schwedlen , A. K. and Harper , B. G. 1997 . Erwinia Herbicola: A Vegetative Bacterial Simulant Division of Life Sciences, U.S. Army DPG, Internal Report 84022
- Willeke , K. , Lin , X. J. and Grinshpun , S. A. 1998 . Improved Aerosol Collection by Combined Impaction and Centrifugal Motion . Aerosol Sci. Technol. , 28 : 439 – 456 .