Abstract
Dispersing hydrophobic compounds in aqueous media is a challenging problem. Many methods and technologies have been, and continued to be developed to address this issue and in this article we explore electrohydrodynamic spraying (electrospray for short) as an alternative approach. In our work a model hydrophobic compound, cholesterol, was successfully dispersed into aqueous media in the form of nanoparticles via electrospray. In total, six cholesterol formulations were prepared via electrospray or bulk mixing methods. The effects of preparation methods on the appearance of the formulations, the final cholesterol concentration, and the loss of cholesterol during filter sterilization were investigated. The bioavailability of the cholesterol in the formulations was examined by culturing the cholesterol auxotrophic wild type NS0 cell line in chemically defined medium supplemented with the different cholesterol formulations. We have demonstrated that electrospray is an efficient and low energy technique to disperse hydrophobic compounds in aqueous media, and we are able to culture NS0 cells using several electrosprayed cholesterol formulations.
1. INTRODUCTION
Hydrophobic compounds play a vital and fundamental role in living organisms. Important drugs, including the anticancer drugs piposulfan, etoposide, paclitaxel, and camptothecin, are hydrophobic, and many consumer products, including foods, paint, lubricants, detergents, and personal care products contain hydrophobic compounds. Conversely, water is the most common continuous phase, both in living organisms and many consumer products. This mismatch leads, therefore, to the basic and challenging problem of how to deliver, or disperse, hydrophobic compounds in an aqueous environment.
A significant number of methods and technologies have been, and continued to be developed to address this problem. Shaking, stirring, whipping, and spraying processes are commonly applied in the consumer products industry. High shear homogenization, ultrasonication, high-pressure homogenization and solvent emulsification/depletion are widespread in the pharmaceutical industry.
Electrohydrodynamic spraying (electrospray for short), in contrast, was developed as a way to disperse material into the gas phase. In this technique a liquid is slowly injected through a capillary tube by a syringe pump. An electrical potential is applied to the capillary tube, and as the fluid passes through it the electric field induces free charges at the surface of the liquid. When the electrical stress overcomes the surface tension the liquid meniscus at the tube opening develops into a conical shape followed by a liquid jet, which is commonly called the Taylor cone-jet mode. The jet is not stable and, due to varicose or kink instabilities (CitationCloupeau and Prunet-Foch 1994), breaks into an aerosol comprised of fine, monodisperse, droplets.
Electrospray is an elegant and versatile tool. It has been used to assist pyrolysis reactions and chemical vapor deposition processes to produce inorganic particles, such as fine metal powder (Sn, Ag, Au, etc.), metal oxide particles (ZrO2, TiO2, etc.), ceramic particles (Si, SiO2), and semiconductor quantum dots (CdSe, GaAs, etc.) (CitationJaworek 2007; CitationSalata 2005). Using a single needle or concentric needles, composite particles, including poly(methyl methacrylate)-pigment nanoparticles and cocoa butter microcapsules containing a sugar solution or an oil-in-water emulsion, have been produced (CitationWidiyandari et al. 2007; CitationLoscertales et al. 2002; CitationBocanegra et al. 2005). Electrospray has also been used to deposit particle suspensions or polymer materials on surfaces to form coatings or on-demand patterns, such as silica particle coatings on a quartz glass, and poly(lactide-co-glycolide) or poly(ethylene glycol) coatings on biomedical implants (CitationJaworek 2007; CitationSalata 2005; CitationKumbar et al. 2007). In biological applications, electrospray is well known for its use in electrospray ionization mass-spectrometry. It has also been used to produce biodegradable polymeric micro/nanoparticles for drug and protein delivery (CitationXie et al. 2006; CitationXie and Wang 2006). Finally, biological materials, such as cells, DNA, insulin, alkaline phosphatase, have also been electrosprayed without changing the biological activity of these materials (CitationJaworek 2007; CitationSalata 2005; CitationJayasinghe et al. 2005; CitationDavies et al. 2005).
The goal of our work is to explore electrospray as an alternative method for dispersing hydrophobic compounds into aqueous media for diverse biological applications, such as the production of cell culture medium supplements or drug delivery carriers. The hydrophobic compound of interest is dissolved in an appropriate solvent, for example ethanol, and sprayed to produce droplets. A collection dish containing the desired aqueous medium is placed below the ground electrode. The solvent evaporates as the droplets travel toward the collection medium and the residual particles, whose size can be much smaller than the droplets originally produced by jet breakup, are collected in the medium. One advantage of this approach is that the energy required to disperse the hydrophobic compounds is only injected into the solvent stream and not into the aqueous medium.
In this article, cholesterol was chosen as a model hydrophobic compound. This material is representative of the hydrophobic materials that must be provided in cell culture media and is an important component of many cell membranes. In particular, cholesterol imparts rigidity to the fluid membrane, increases the mechanical durability of the lipid bilayer, and plays a key role in lipid raft assembly and function (CitationSimons et al. 2000). From the drug delivery perspective, cholesterol is also a good model for lipophilic drugs. Like most lipophilic drugs the solubility of cholesterol in aqueous solutions is very low, on the order of 1.8 μ g/mL or 4.7 μ M (CitationHaberland and Reynolds 1973), and, like the well known anticancer drug Taxol®, cholesterol (molecular weight = 387.6) is a small molecule. In addition, the solubility of lipophilic drugs in lipids is much higher than in aqueous solutions, and, therefore, lipid nanoparticles, like the cholesterol nanoparticles in this work, may also be useful as carriers to deliver lipophilic drugs.
To date we have demonstrated that we can produce cholesterol nanoparticles via electrospray, capture them in liquid media, and characterize them using dynamic light scattering. We have tested the biological activity of these nanoparticles on a wild type cholesterol dependent cell line, NS0 cell line from murine myeloma (Sigma 85110503) and we are able to culture the cells using several of the electrosprayed cholesterol formulations.
2. MATERIALS AND METHODS
Preparation of Cholesterol Formulations
A schematic of the electrospray apparatus used in this work is available in the supplementary material. To optimize the preparation process of cholesterol formulations, we used a three factor (cholesterol concentration, liquid flow rate and needle size) 3 × 3 × 2 full factorial experimental design with two replicates. The optimized process was as follows: cholesterol powder (Sigma C3045) was dissolved in ethanol (Fisher Scientific A405P-4 or Pharmco-Aaper E200GP) at a concentration of 2 mg/mL. The mixture was delivered to a 27-gauge needle by a syringe pump at a flow rate of 2 mL/h. A voltage of approximate 2500 V was applied to the needle and the observation of a stable Taylor cone-jet mode confirms the proper conditions for generating fine droplets. The ethanol evaporated from the droplets, aided by heat input from the lamp, and the residual cholesterol particles were captured in an aqueous solution placed in a grounded aluminum dish 5.5 cm below the needle tip. The collection time for all electrosprayed formulations was 1.5 h.
Three cholesterol formulations were prepared by collecting the electrosprayed cholesterol particles in solutions with one of the following final compositions: 1% Pluronic F68 in 1X PBS (formulation referred to as ChoPlu), 1% Pluronic F68 in a solution of 25 mg/mL methyl-β -cyclodextrin in 1X PBS (formulation referred to as ChoCycPlu), or 25 mg/mL methyl-β -cyclodextrin in 1X PBS (formulation referred to as ChoCyc). Methyl-β -cyclodextrin is a widely used carrier molecule/solvent for cholesterol in NS0 cell culture (CitationGorfien et al. 2000; CitationKeen et al. 1995; CitationKeen et al. 1996; CitationWalowitz et al. 2003) and is, therefore, important to study in order to compare our results with previous work. All cholesterol formulations were sterilized by filtering through 0.22 μ m PVDF filters (Fisher Scientific 09-720-3) before they were used in cell culture.
In addition to the electrospray method, a cholesterol formulation was also produced by bulk mixing (ChoCyc Mix): methyl-β -cyclodextrin was first dissolved in 1X PBS at a concentration of 25 mg/mL. Cholesterol powder was then added to the methyl-β -cyclodextrin/PBS solution, mixed with a vortex mixer for 1 min, and stored at 25°C for several days until the cholesterol powder, based on visual observations, was completely dissolved. The formulation was sterilized by filtering through 0.22 μ m PVDF filters.
Two other commercial cholesterol formulations were tested. The first formulation (SyntheChol) was 500X SyntheChol™ NS0 supplement (Sigma S5442)—a proprietary cholesterol supplement in liquid form that is already sterilized and requires no further preparation. The second formulation (WatCho Mix) was water soluble cholesterol (Sigma C4951). This is a powdered product that contains 40 mg cholesterol per gram and the balance is methyl-β -cyclodextrin. Water soluble cholesterol powder was dissolved in 1X PBS solutions, at a cholesterol concentration of 1500 μ g/mL, and sterilized by filtering through 0.22 μ m PVDF filters.
Cholesterol Nanoparticle Size Measurements
Cholesterol particle size distributions were measured using dynamic light scattering (Brookhaven Instruments Corporation, BI 200SM). The concentrated cholesterol formulations were first diluted with particle-free distilled water, if necessary, to make the intensity of scattered light falls into an appropriate range. The wavelength of the laser was 632.8 nm and the detection angle was 90°. All measurements were carried out at 25°C with water as the solvent. The refractive index of the particles was set to 1.59 + 0i. Each measurement lasted 2 min. Each sample was measured 3 times and the average effective diameter was reported.
Cholesterol Concentration Measurements
Cholesterol concentrations were measured using an Amplex Red Cholesterol Assay Kit (Invitrogen, CA A12216). This assay is based on an enzyme-coupled reaction in which cholesterol is oxidized by cholesterol oxidase to yield H2O2 and the corresponding ketone product. H2O2 subsequently reacts with 10-acetyl-3,7-dihydroxyphenoxazin (Amplex Red reagent) to produce highly fluorescent resorufin.
Concentrated cholesterol formulations were pipeted from the center of the sample and diluted with 1X Reaction Buffer. 50 μ L aliquots of the diluted formulations or cholesterol standard samples were pipeted into a 96-well transparent bottom microplate. 50 μ L aliquots of the working solution containing the Amplex Red Reagent were then added to each microplate well. The microplate was incubated in the dark at 37°C for 30 min. The fluorescence was measured using an excitation at 530 nm and fluorescence detection at 590 nm. Each sample had 3 replicates and the averaged concentration was reported.
In the final stage of this study we found that the denatured ethanol (Fisher Scientific A405P-4; 95.27% ethyl alcohol, 1% methyl isobutyl ketone, 1.0% ethyl acetate, 1% hydrocarbon, 1.73% other compounds) used to dissolve the cholesterol for formulations produced by electrospray generated a strong fluorescence signal when analyzed with the Amplex Red Cholesterol Assay Kit (Invitrogen, CA A12216). When 50 μ L of denatured alcohol was mixed with the Amplex Red reagent, the solution turned pink immediately, while no color change was observed for 50 μ L 200 proof alcohol (100% ethyl alcohol). Thus, for samples made with denatured alcohol the assay kit severely overestimated the concentration of the cholesterol present. In contrast, there was no signal when 200 proof ethanol (Pharmco-Aaper E200GP) was tested alone. Due to the difficulty of directly measuring the cholesterol concentrations of the original electrosprayed formulations by other, more complex, analytical methods, we used the following indirect method to estimate the true cholesterol concentrations. Solutions containing 2 mg/mL cholesterol were produced using either denatured or 200 proof ethanol. The mixtures were electrosprayed for 1.5 h using our standard operating conditions and collected in the 1% Pluronic F68/1X PBS solution. Two electrosprayed samples were produced from each solution, and the Amplex Red Cholesterol Assay Kit was then used to measure (in triplicate) the cholesterol concentrations. When denatured ethanol was used, the measured cholesterol concentration was 1730 ± 56 μ g/mL, a value that is 10 times higher than the 165 ± 21 μ g/mL measured when 200 proof ethanol was used. The latter corresponds to a cholesterol capture efficiency in the solution of about 40%. We therefore divided the cholesterol concentration measured for the original electrosprayed cholesterol formulations by a factor of 10 and these are the values reported in this paper. Formulations made by other means did not involve ethanol, so they were not affected by this problem.
Routine NS0 Culture Procedure
15 mL chemically defined hybridoma medium (Sigma H4409) supplemented with 8 mM L-glutamine (Sigma G7513) and 1X SyntheChol™ NS0 supplement (Sigma S5442) was placed in 75 cm2 T-flasks. NS0 cells were then seeded into the flasks at a concentration of 3 × 105 viable cells/mL and incubated at 37°C in a humidified atmosphere of 5% CO2 in air. This procedure was repeated every 3 days.
Bioavailability of Cholesterol Nanoparticles to NS0 Cells
15 mL chemically defined hybridoma medium supplemented with 8 mM L-glutamine was placed in 75 cm2 T-flasks. Concentrated cholesterol formulations were diluted and added to these flasks to produce the desired final concentration levels of cholesterol in the culture medium. The cholesterol concentration in the medium was measured immediately after addition, as well as 5 h and 24 h after the addition. NS0 cells were washed twice with cholesterol free hybridoma medium, and then seeded into the flasks at a concentration of 3 × 105 viable cells/mL, and incubated at 37°C in a humidified atmosphere of 5% CO2 in air. 200 μ L medium was taken out daily by pipeting below the surface of the medium. 10 μ L medium was used to count cells using the Trypan blue staining method to obtain growth curves. The remaining 190 μ L medium was centrifuged at 200 g for 5 min, and the concentration of cholesterol in the supernatant was measured to track the change in the cholesterol concentration during cell culture. Each condition had 3 replicates and results were reported as mean ± standard deviation.
The long-term cell maintenance experiment monitored cell growth in medium supplemented with ChoCycPlu and SyntheChol in 10 sequential passages. The cells were subcultured every 3 or 4 days. During the 10th passage, cells were cultured for 6 days and counted daily using the trypan blue staining method to obtain the growth and viability curves. Each condition had 3 replicates and results were reported as mean ± standard deviation.
3. RESULTS AND DISCUSSION
When producing the original cholesterol formulations via electrospray, we dissolved the cholesterol in denatured ethanol. As noted in the materials and methods section, unknown compounds in this ethanol reacted and fluoresced and the assay kit reported cholesterol concentrations that we estimate are ∼ 10 times too high. When we discovered this problem, we made new electrosprayed cholesterol formulations using 200 proof ethanol, and repeated all of the physical characterization experiments. The biological assays, however, were only conducted using the original formulations because the NS0 cell line had been lost in a cryo-storage failure and could not be easily replaced.
Creation of Concentrated Cholesterol Formulations
As summarized in , concentrated cholesterol formulations were created using the electrospray method and collected in three different solutions. In addition, two cholesterol formulations were created by bulk mixing: one by dissolving powdered cholesterol, at concentrations of 500, 1000, and 1500 μ g/mL into 25 mg/mL methyl-β -cyclodextrin-1X PBS solution, and the second by dissolving water soluble cholesterol, at a concentration of 1500 μ g/mL in 1X PBS. In order to make a direct comparison with the commercial product, we measured the cholesterol concentration of 500X SyntheChol™ NS0 supplement and found it to be 1500 μ g/mL. Thus, this value was used as the highest concentration for the formulations made by bulk mixing.
TABLE 1 Summary of cholesterol formulations investigated
For the cholesterol powder, the bulk mixing method did not work for all of the desired cholesterol concentrations. The cholesterol only dissolved completely in the 500 μ g/mL solution and this took approximately 2–3 days. The cholesterol in the 1000 and 1500 μ g/mL solutions never completely dissolved. In contrast, the water soluble cholesterol dissolved in 1X PBS immediately but after storage at 4°C white floccules appeared and these did not redissolve even after the temperature of the solution was brought back to 25°C. The concentration of cholesterol in the solution simultaneously decreased from an initial value of 1500 μ g/mL to about 1000 μ g/mL, consistent with cholesterol flocculation.
Appearance of the Cholesterol Formulations
Each formulation was first characterized based on visual appearance. summarizes the results. The appearance of the cholesterol formulations produced by electrospray differed depending on the collection solution used, but did not depend on whether denatured or 200 proof ethanol was used to dissolve the cholesterol. When methyl-β -cyclodextin was not added to the collection solutions, the formulation looked milky before sterilization and slightly milky after sterilization. As discussed in more detail below, the change in appearance was accompanied by a decrease in particle size that was most likely due to the remove of large particles and the breakup of loose aggregates/floccules during filtration. All other formulations were clear both before and after sterilization.
TABLE 2 Appearance of the cholesterol formulations
Size Distribution of Cholesterol Nanoparticles
Dynamic light scattering was used to detect the presence of particles, and, where possible, determine the size distribution parameters. summarizes the results of these experiments, and the values reported are based on data from 3 to 10 independently produced samples. Two parameters are reported: the effective diameter and the “A CR (avg.)” values. The latter has units of kilo counts per second (kcps), and is an indirect measure of the particle number density since, at constant pinhole setting and particle size, the count rate increases with particle number density.
TABLE 3 Cholesterol nanoparticle sizes of all formulations before and after filtration
Since Pluronic F68 is a polymer surfactant, it self-assembles into micelles when the concentration reaches critical micelle concentration (CMC = 0.04 mM). We first measured the particle size from the 1% Pluronic F68/1X PBS and the 25 mg/mL methyl-β -cyclodextrin/1X PBS solutions. Prior to filtration we found the particle (micelle) size was about 1 μ m, while after filtration, scattering from either solution was insignificant. Thus, we do not expect micelle formation or scattering from Pluronic F68 and methyl-β -cyclodextrin to interfere with scattering from the cholesterol particles in the filtered formulations.
We then measured scattering from all of the concentrated cholesterol formulations before and after filtration. No significant scattering was observed from the water soluble cholesterol either before or after filtration. All of the remaining formulations had some scattering from the original formulations but only those containing Pluronic F68 had scattering signals strong enough to analyze after filtration. illustrates a typical size distribution measured for the cholesterol nanoparticles (made with denatured ethanol) collected in 1% Pluronic F68/ 1X PBS solutions (ChoPlu). The effective diameter of the cholesterol particles in the ChoPlu sample was 137 nm and the polydispersity was 0.066. When 200 proof ethanol was used to dissolve the cholesterol powder in the electrosprayed formulations, the effective diameters of the ChoPlu and the ChoCycPlu formulations after sterile filtration were both ∼ 150 nm. The stability of the electrospray formulations is quite good. When stored for two weeks at 4°C (20°C), the average particle size of the ChoPlu sample () increased from 149 nm to 159 nm (182 nm).
FIG. 1 A typical size distribution of cholesterol nanoparticles collected in 1% Pluronic F68/ 1X PBS (Dp, effective = 137 nm after sterilization with a 0.22 μ m PVDF filter, polydispersity = 0.066).
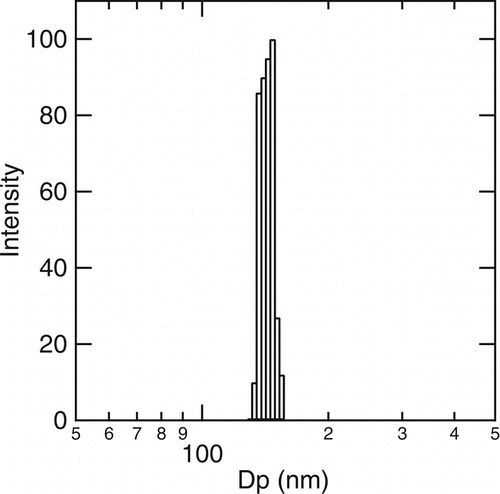
For the ChoPlu and ChoCycPlu samples, we compared the observed particle size in the aqueous phase to that expected based on electrospray scaling laws assuming that the low concentrations of cholesterol do not change the physical properties of ethanol. As detailed in the supplementary material, the calculations suggest that Coulombic fissioning is significant under the conditions of these experiments.
Cholesterol Concentration
summarizes the concentration of cholesterol, in units of μ g/mL for each of the five cholesterol formulations both before and after filtration. The reported values are based on measurements of 3 to 10 independently produced samples. The concentration of cholesterol in all of the formulations was several orders of magnitude higher than the solubility of cholesterol (1.8 μ g/mL) in water. For the electrosprayed formulations the cholesterol concentrations reported in correspond to our standard collection time of 1.5 h. When methyl-β -cyclodextrin is present, the concentrations can be increased by increasing the collection time. For example, we made ChoCyc and ChoCycPlu formulations containing ∼ 500 μ g/mL cholesterol by spraying for 5 h. In the absence of methyl-β -cyclodextrin (ChoPlu formulations), collecting for more than 1.5 h produces formulations that are difficult to filter.
TABLE 4 Cholesterol concentration before and after filtration
The concentration of cholesterol in the formulations before and after filtration only changed by 2.4% to 16.7%, values that are much lower than the almost 100% cholesterol loss observed by Gorfein et al. (2000) during filtration of their LTI 1st generation supplements at 1X concentration. Their large cholesterol losses may be due to the low initial cholesterol concentrations (the absolute concentrations are not reported) used by Gorfein et al. (2000) or the choice of the filter membranes. We observed cholesterol losses as high as 90% if we used HT Tuffryn membrane (VWR 28142-340) rather than the PVDF membranes used here. The 2.4% to 16.7% cholesterol losses are not enough to account for the large change in particle size distribution parameters (), which suggests that only nanoparticles are able to pass through the filter, and the difference in particle size measured before and after filtration is mainly caused by the breakup of loose aggregates/floccules or the removal of large foreign particles, such as dust, that entered the solutions during collection. Large particles scatter light strongly, giving a strong signal and affecting the reported particle size significantly.
The data in also show that ChoPlu had a very high “A CR (avg.)” value, indicating a high concentration of cholesterol nanoparticles in the formulation. Following the same logic, the low “A CR (avg.)” values indicate that not nearly as many cholesterol particles existed in the ChoCyc, ChoCycPlu, ChoCyc Mix, and WatCho Mix formulations. Since the cholesterol concentrations of these formulations are high, the cholesterol most likely exists as a molecular complex with the methyl-β -cyclodextrin (CitationGorfien et al. 2000).
In summary, the characterization studies suggest that in the ChoCyc, ChoCyc Mix, and WatCho Mix formulations the cholesterol is largely solubilized by the methyl-β -cyclodextrin. In the absence of methyl-β -cyclodextrin, the cholesterol is present as nanoparticles (ChoPlu). When both Pluronic F68 and methyl-β -cyclodextrin are present (ChoCycPlu) part of the cholesterol is solubilized by methyl-β -cyclodextrin and part of the choleseterol is present as nanoparticles.
Compared with bulk mixing, electrospray is a more efficient technique of dispersing hydrophobic compounds into aqueous solutions and can produce more concentrated cholesterol formulations than bulk mixing. Even without methyl-β -cyclodextrin, the amount of cholesterol dispersed by electrospray in aqueous solutions was about 100 times higher than the solubility of cholesterol in water. With methyl-β -cyclodextrin, higher concentrations were achieved in ChoCyc than in ChoCyc Mix. The fact that we did not have to wait several days for the solution to form suggests that the high surface area created by electrospray is an advantage because it significantly decreases the preparation time. In addition, when we simply mixed cholesterol/ethanol solutions with methyl-β -cyclodextrin solutions, rather than producing a clear solution, cholesterol floccules formed. Thus, although the components of the ChoCyc and the ChoCyc Mix formulations are essentially the same, the preparation method makes a significant difference in the appearance of the final product.
Bioavailability of Cholesterol for NS0 Cells
While NS0 cells normally require serum as a cholesterol source, several research groups have demonstrated the growth of NS0 cells in protein free and chemically defined medium, using chemically defined cholesterol supplements (CitationKeen et al. 1995; CitationKeen et al. 1996; CitationGorfien et al. 2000; CitationOhmori 1988; CitationOjito et al. 2001; CitationWalowitz et al. 2003; CitationZhang et al. 2005; CitationSpens and Haggstrom 2005; CitationWalowitz et al. 2005; CitationTalley et al. 2005). The auxotrophic nature of NS0 cells with respect to cholesterol makes this cell line a natural choice for characterizing the bioavailability of cholesterol in the different formulations produced in this work, especially the cholesterol nanoparticles in ChoPlu produced by electrospray.
ChoPlu, ChoCyc, ChoCycPlu, ChoCyc Mix, WatCho Mix, and SyntheChol were diluted and added to cholesterol free hybridoma medium at concentrations of 0.6 μ g/mL, 3 μ g/mL, 6 μ g/mL, or 9 μ g/mL. illustrates the growth of NS0 cells as a function of the cholesterol formulations. The SyntheChol results are shown in each figure because this commercially available product was used to subculture the cells and is, therefore, the positive control. All the formulations supported cell growth. The ChoPlu formulation could only support cell growth at higher concentration levels relative to SyntheChol, while the performance of formulations made by bulk mixing were similar to SyntheChol. Our results with water soluble cholesterol contrast with those of CitationGorfien et al. (2000) who found that water soluble cholesterol did not promote growth at all.
FIG. 2 (a) Growth of NS0 cells supplemented with SyntheChol, ChoPlu, ChoCycPlu, and ChoCyc (3 replicates). (b) Growth of NS0 cells supplemented with SyntheChol, ChoCyc Mix, and WatCho Mix (3 replicates). The * indicates that the correction factor of 10 was applied.
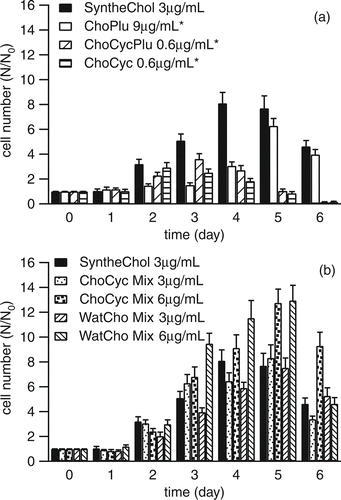
To better understand the reasons for these differences in growth behavior we tracked the change of cholesterol concentration in the T-flasks before and after NS0 cells were seeded. presents the same sequence of T-flask cultures as in , but in these figures, the y-axis represents the concentration of cholesterol and the x-axis is extended to include times before the cells were seeded into the T-flasks. For all of the formulations there was an immediate and often dramatic decrease in the cholesterol concentration, from the calculated value based on dilution, immediately after the addition of the formulation to the medium. For example, when ChoPlu was added at a concentration level corresponding to 9 μ g/mL, the cholesterol concentration in the medium immediately decreased to 0.4 μ g/mL (t = –24 h), and further decreased to 0.2 μ g/mL after 24 h (t = 0– h). Similar losses were observed when these experiments were repeated with nanoparticles produced using 200 proof ethanol. Even for SyntheChol, where the initial concentration was close to the expected value of 3 μ g/mL, the concentration after 24 h was reduced to 1.35 μ g/mL.
FIG. 3 Change of cholesterol concentration in medium before (– 24 h to 0– h) and after (0+ h till 144 h) NS0 cells were seeded. “–24”: right after adding supplements into T-flasks without seeding any NS0 cells. “–19”: 5 hours after adding supplements into T-flasks without seeding any NS0 cells. “0–”: 24 hours after adding supplements into T-flasks without seeding any NS0 cells. “0+”: right after NS0 cells were seeded. “24”: 24 hours after NS0 cells were seeded (Day 1). “48”: 48 hours after NS0 cells were seeded (Day 2). “72”: 72 hours after NS0 cells were seeded (Day 3). “96”: 96 hours after NS0 cells were seeded (Day 4). “120”: 120 hours after NS0 cells were seeded (Day 5). “144”: 144 hours after NS0 cells were seeded (Day 6). (a) Supplements were SyntheChol, ChoPlu, ChoCycPlu, and ChoCyc. (b) Supplements were SyntheChol, ChoCyc Mix and WatCho Mix. Each had 3 replicates. *Correction factor of 10 was applied and values have been multiplied by a factor of 5 for clarity.
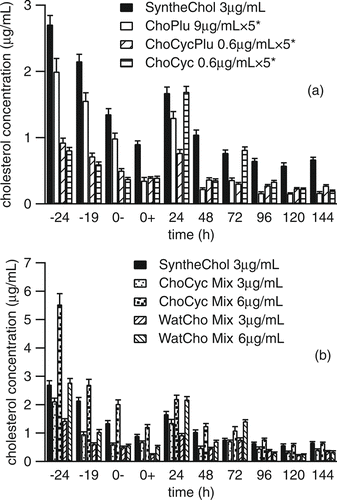
In the absence of cells, these losses can only be explained by physical or chemical processes such as adsorption of the cholesterol, or cholesterol nanoparticles, onto the T-flask surfaces or at the air-liquid interface. Other studies have shown that the material used to make the culture vessel can have a dramatic impact on the viability of cultured cells. CitationKadarusman et al. (2005), for example, found that cholesterol-dependent NS0 cells cultured using 1000X cholesterol lipid concentrate from Invitrogen did not grow in wave bioreactors made from low-density polypropylene (LDPE) bags because of the significant loss of cholesterol in the first 5 h that they attributed to adsorption of cholesterol onto the walls. They also pointed out that adsorption is minimized when fluorinated ethylene propylene (FEP) is used as the reactor material. In our studies the T-flasks were made from polystyrene and furthermore, the surface to volume ratio (10:1) is fairly high. Thus, cholesterol loss by adsorption at an interface is a reasonable explanation for the initial steep decrease in cholesterol concentration. The differences observed between the six formulations are probably due to the form the cholesterol takes. In formulations that contain particles, rather than cholesterol–methyl-β -cyclodextrin complexes, adsorption of a particle at a surface should remove far more cholesterol than adsorbing a free or complexed cholesterol molecule.
It is also interesting to note that immediately after adding the cells (t = 0+ h), there was an additional drop in cholesterol concentration for all of the formulations. This observation confirms that cholesterol was not carried over into the culture by the seeded cells, and suggests that the hydrophobic cell surface can also attract cholesterol.
Given that the surface area of the nanoparticles is large, one would expect the concentration of cholesterol to stabilize at the cholesterol solubility limit of 1.8 μ g/mL, rather than the much lower values observed here even before cell culture started. Unfortunately, from the cholesterol delivery standpoint, the nanoparticles appeared to be rather stable. CitationMüller et al. (2004) explained that the increased stability of some lipid nanoparticles may be due to the formation of a degraded outer monolayer. CitationMüller et al. (2004) also pointed out that only the surface of the nanoparticles is accessible to water and oxygen, thus, if a degraded outer layer of molecules forms, it will protect the inner part, much like the oxidized layer that forms on top of aluminum does. This is consistent with the fact that pure cholesterol powder must be stored at –20°C to prevent degradation. In formulations where the cholesterol was complexed with methyl-β -cyclodextrin, the lipid concentration was more stable in the aqueous phase although some losses were still observed.
Previous work by CitationGorfien et al. (2000) and CitationWalowitz et al. (2003) found that “carryover” nutrient effects might permit NS0 cells to survive for a limited duration in the absence of exogenously supplied sterol. Although our cholesterol measurements presented in suggested that carryover was not an issue we still conducted 10 sequential passages of NS0 cells in cholesterol free medium supplemented with 0.6 μ g/mL ChoCycPlu to ensure that the growth of NS0 cells was sustainable. The results, shown in , demonstrate that although the growth of NS0 cells supplemented with ChoCycPlu may not be quite as good as that supplemented with SyntheChol, ChoCycPlu did maintain long term cell growth, and furthermore, the NS0 cells did not need levels of cholesterol as high as those provided by 1X SyntheChol. The growth curve and viability of the 10th passage are shown in . Here, we found that the growth of NS0 cells supplemented with ChoCycPlu caught up with those supplemented with SyntheChol by Day 4 and that the two groups reached peak cell density at different times.
4. CONCLUSIONS
Electrospray was used to produce monodisperse cholesterol nanoparticles that were readily collected in aqueous media. Different cholesterol formulations were prepared using electrospray or bulk mixing. Compared with bulk mixing, electrospray was found to be a more efficient technique for dispersing hydrophobic compounds into aqueous solutions. The bioavailability of cholesterol in these formulations was investigated using the cholesterol auxotrophic NS0 cell line and 1X SyntheChol as the positive control. Cholesterol nanoparticles stabilized by 1% Pluronic F68 only supported the growth of NS0 cells at relatively high initial loadings, most likely because a large fraction of the cholesterol nanoparticles went to the interface of gas-liquid or were absorbed by the T-flask's walls. Formulations that contained methyl-β -cyclodextrin maintained the cholesterol concentration in the medium at levels high enough to support the growth of NS0 cells. In the future, surfactants or solvents other than Pluronic F68 and methyl-β -cyclodextrin, especially small-molecule surfactants, such as nonyl-maltopyranoside (CitationHu et al. 2008), should be investigated to determine their ability to maintain cholesterol concentration in the medium because these small-molecule surfactants are not harmful to cells and they are easily being removed in downstream purification.
Supplementary_Material.zip
Download Zip (146.3 KB)Acknowledgments
This work was supported by the National Science Foundation under grant No. EEC-0425626. We gratefully acknowledge Dr. Weiwei Hu from Biogen Idec and Dr. Xiaodong Tong from University of Minnesota for their valuable suggestions.
[Supplementary materials are available for this article. Go to the publisher's online edition of Aerosol Science and Technology to view the free supplementary file.]
Notes
*After a few days storage at 4°C cholesterol precipitated.
a Signal was too weak to get effective diameter.
b Cholesterol particle size of SyntheChol was not measured.
+SyntheChol was already sterilized when purchased from Sigma. Therefore, no filter sterilization was needed.
*The electrosprayed formulations were prepared with a collection time of 1.5 h.
REFERENCES
- Bocanegra , R. , Gaonkar , A. G. , Barrero , A. , Loscertales , I. G. , Pechack , D. and Marquez , M. 2005 . Production of Cocoa Butter Microcapsules Using an Electrospray Process . J. Food Sci. , 70 : E492 – E497 .
- Cloupeau , M. and Prunet-Foch , B. 1994 . Electrohydrodynamic Spraying Functioning Modes: A Critical Review . J. Aerosol Sci. , 25 : 1021 – 1036 .
- Davies , L. A. , Hannavy , K. , Davies , N. , Pirrie , A. , Coffee , R. A. , Hyde , S. C. and Gill , D. R. 2005 . Electrohydrodynamic Comminution: A Novel Technique for the Aerosolisation of Plasmid DNA . Pharm. Res. , 22 : 1294 – 1304 .
- Gorfien , S. , Paul , B. , Walowitz , J. , Keem , R. , Biddle , W. and Jayme , D. 2000 . Growth of NS0 Cells in Protein-Free, Chemically Defined Medium . Biotechnol. Progr. , 16 : 682 – 687 .
- Haberland , M. E. and Reynolds , J. A. 1973 . Self-Association of Cholesterol in Aqueous Solution . Proc. of the Natl. Acad. of Sci., USA , 70 : 2313 – 2316 .
- Hu , W. , Rathman , J. J. and Chalmers , J. J. 2008 . An Investigation of Small Molecule Surfactants to Potentially Replace Pluronic F-68 for Reducing Bubble-Associated Cell Damage . Biotechnol. Bioeng. , 101 : 119 – 127 .
- Jaworek , A. 2007 . Micro- and Nanoparticle Production by Electrospraying . Powder Technol. , 176 : 18 – 35 .
- Jayasinghe , S. N. , Qureshi , A. N. and Eagles , P. A. M. 2005 . Electrohydrodynamic Jet Processing: An Advanced Electric-Field-Driven Jetting Phenomenon for Processing Living Cells . Small. , 2 : 216 – 219 .
- Kadarusman , J. , Bhatia , R. , McLaughlin , J. and Lin , W. R. 2005 . Growing Cholesterol-Dependent NS0 Myeloma Cell Line in the Wave Bioreactor System: Overcoming Cholesterol-Polymer Interaction by Using Pretreated Polymer or Inert Fluorinated Ethylene Propylene . Biotechnol. Progr. , 21 : 1341 – 1346 .
- Keen , M. J. and Hale , C. 1996 . The Use of Serum-Free Medium for the Production of Functionally Active Humanized Monoclonal Antibody from NS0 Mouse Myeloma Cells Engineered Using Glutamine Synthetase as a Selectable Marker . Cytotechnology. , 18 : 207 – 217 .
- Keen , M. J. and Steward , T. W. 1995 . Adaptation of Cholesterol-Requiring NS0 Mouse Myeloma Cells to High Density Growth in a Fully Defined Protein-Free and Cholesterol-Free Culture Medium . Cytotechnology. , 17 : 203 – 211 .
- Kumbar , S. G. , Bhattacharyya , S. , Sethuraman , S. and Laurencin , C. T. 2007 . A Preliminary Report on a Novel Electrospray Technique for Nanoparticle Based Biomedical Implants Coating: Precision Electrospraying . J. Biomed. Mater. Res. B, Appl. Biomater. , 81B : 91 – 103 .
- Loscertales , I. G. , Barrero , A. , Guerrero , I. , Cortijo , R. , Marquez , M. and Ganan-Calvo , A. M. 2002 . Micro/Nano Encapsulation Via Electrified Coaxial Liquid Jets . Science. , 295 : 1695 – 1698 .
- Müller , R. H. and Keck , C. M. 2004 . Challenges and Solutions for the Delivery of Biotech Drugs—A Review of Drug Nanocrystal Technology and Lipid Nanoparticles . J. Biotechnol. , 113 : 151 – 170 .
- Ohmori , H. 1988 . Development of a Serum-Free Medium for in vitro Immune Responses by Using β -cyclodextrin . J. Immunol. Methods. , 112 : 227 – 233 .
- Ojito , E. , Labrada , G. , Garcia , Z. , Garcia , N. and Chico , E. Study of NS0 Cell Line Metabolism in Lipid Supplemented Protein Free Media . Animal Cell Technology: From Target to Market: Proceedings of the 17th ESACT Meeting . June 10-14 2001 , Tylösand, Sweden. Edited by: indner-Olsson , E. , Chatzissavidou , N. and Lüllau , E. pp. 179 – 182 . Kluwer Academic Publishers .
- Salata , O. V. 2005 . Tools of Nanotechnology: Electrospray . Current Nanoscience. , 1 : 25 – 33 .
- Simons , K. and Ikonen , E. 2000 . How Cells Handle Cholesterol . Science. , 290 : 1721 – 1726 .
- Spens , E. and Haggstrom , L. 2005 . Defined Protein-Free NS0 Myeloma Cell Cultures: Stimulation of Proliferation by Conditioned Medium Factors . Biotechnol. Progr. , 21 : 87 – 95 .
- Talley , D. , Cutak , B. , Rathbone , E. , Al-Kolla , T. , Allison , D. , Blasberg , J. , Kao , K. and Caple , M. SyntheChol™ Synthetic Cholesterol for Cholesterol Dependent Cell Culture-development of Non-animal Derived Chemically Defined NS0 Medium . Animal Cell Technology Meets Genomics: Proceedings of the 18th ESACT Meeting . May 11–14 2003 , Granada, Spain. Edited by: Gòdia , F. and Fussenegger , M. pp. 577 – 580 . Netherlands : Springer .
- Walowitz , J. L. , Fike , R. M. and Jayme , D. W. 2003 . Efficient Lipid Delivery to Hybridoma Culture by Use of Cyclodextrin in a Novel Granulated Dry-Form Medium Technology . Biotechnol. Progr. , 19 : 64 – 68 .
- Walowitz , J. , Tescione , L. , Paul , W. , Jayme , D. and Gorfien , S. Optimized Feeding Strategy for NS0 Cells . Animal Cell Technology Meets Genomics: Proceedings of the 18th ESACT Meeting . May 11–14 2003 , Granada, Spain. Edited by: Gòdia , F. and Fussenegger , M. pp. 711 – 714 . Netherlands : Springer .
- Widiyandari , H. , Hogan , C. J. J. , Yun , K. M. , Iskandar , F. , Biswas , P. and Okuyama , K. 2007 . Production of Narrow-Size-Distribution Polymer-Pigment-Nanoparticle Composites via Electrohydrodynamic Atomization . Macromol. Mater. Eng. , 292 : 495 – 502 .
- Xie , J. , Lim , L. K. , Phua , Y. , Hua , J. and Wang , C. H. 2006 . Electrohydrodynamic Atomization for Biodegradable Polymeric Particle Production . J. Colloid and Interface Sci. , 302 : 103 – 112 .
- Xie , J. and Wang , C. H. 2006 . Encapsulation of Proteins in Biodegradable Polymeric Microparticles Using Electrospray in the Taylor Cone-Jet Mode . Biotechnol. Bioeng. , 97 : 1278 – 1290 .
- Zhang , J. and Robinson , D. 2005 . Development of Aninal-Free, Protein-Free and Chemically-Defined Media for NS0 Cell Culture . Cytotechnology. , 48 : 59 – 74 .