Abstract
This study evaluated the UN-ECE Particle Measurement Programme (PMP) protocol for the measurement of solid particle number emissions under laboratory and on-road conditions for two passive diesel particle filters (DPF)–equipped medium and heavy-heavy duty diesel vehicles. The PMP number emissions were lower than the European light-duty certification value (9.6 × 1011 #/mi) for all standardized cycles, but exceeded this value during some higher load on-road driving conditions. Particle number measurements were generally less variable than those of the PM mass for the on-road testing, but had comparable or greater variability than PM mass for the laboratory measurements due to outliers. These outliers appear to be real events that are not apparent with integrated filter methods. The particle number measurements for the low cut point CPCs (3–7 nm) below the PMP system were approximately an order of magnitude higher than those for the PMP-compliant CPC (23 nm), indicating the presence of a large fraction of solid sub-23 nm particles. Although such particles are defined as solid by the PMP method, their actual state is unknown. Nucleation particles with a large sulfate contribution formed under a variety of conditions when the exhaust temperature near the DPF exceeded a “critical” temperature, typically >300°C.
1. INTRODUCTION
With regulatory limits in California and the United States (US) for 2007 heavy-duty diesel engines being met with the use of diesel particle filters (DPFs), efforts continue to try to improve the methods for the measurement of particulate matter (PM) at the resulting very low levels. Improvements to the gravimetric method have been issued by the US Environmental Protection Agency (US EPA) (CitationCFR, 2008) and in Europe (CitationAndersson et al. 2007a) for emission certification. While the new gravimetric methods are adequate for measurements near the certification limit, quantifying PM emissions will continue to be a challenge at the much lower emission levels that current wall-flow DPFs typically achieve.
The European Community has launched an extensive multi-nation research initiative under the auspices of the United Nation's Economic Commissions for Europe-Group of Experts on Pollution and Energy (UN-ECE-GRPE) to investigate a new PM regulation method (CitationAndersson et al. 2007a,Citationb; CitationGiechaskiel et al. 2008; CitationBosteels et al. 2007). The methodology developed under this program is the measurement of solid particle number above a particle size of 23 nanometers (nm) at 50% counting efficiency (D50). The measurement of solid particles has been studied and developed through the UN-ECE-GRPE Particle Measurement Programme (PMP). They concluded the solid particle methodology provided improvements over the mass method in terms of limits of detection, accuracy, discrimination power, variability, and reduced volatile artifacts (CitationAndersson et al. 2007a). They also concluded the solid particle methodology could discriminate between emission levels 20 times lower than a filter-based gravimetric measurement under the testing conditions used. While counting only solid particles may not be indicative of all the diesel PM components of interest from a health effects perspective, this still represents an advancement in measuring particles at the low emission levels of current wall-flow DPF-equipped engines.
To date, our efforts in California are the only studies of the PMP protocol in the US (CitationHerner et al. 2007a; CitationZhang et al. 2008). The objective of this study was to evaluate the proposed PMP method for determining particle emissions from medium-heavy and heavy-heavy duty diesel vehicles and its potential in California for PM measurements in the laboratory and in-use. For this study, comparisons between gravimetric PM mass and solid particle number (using the PMP protocols) were made under laboratory conditions on a chassis dynamometer and on-road conditions using a mobile reference laboratory. The on-road testing element was specifically added to evaluate the effectiveness of the European PMP methodology under real-world driving conditions. The highlights of this project are summarized in this paper and discussed in greater detail in CitationDurbin et al. (2008).
2. EXPERIMENTAL
2.1. PMP Measurement System
The PMP measurement system is a particle sampling system specifically designed to measure the number concentration of solid, non-volatile particles. The initial regulatory work for the European PMP focused on light-duty diesel and gasoline direct injection applications (CitationAndersson et al. 2007a). The PMP is also now being examined for heavy-duty applications in Europe (CitationAndersson et al. 2007b). Our study is a direct contribution to that international effort.
The term “solid” particles is an operational definition, based on the PMP design, with “solid” particles defined as ones having a diameter between 23 nm and 2.5 μm and of sufficiently low volatility to survive a residence time of 0.2 s at 300°C. The PMP system consists of a pre-classifier/cyclone sampling directly from the constant volume sampler (CVS) with a 2.5 μm size cut, a volatile particle remover (VPR) for volatile species control, and a CPC. The VPR includes an initial hot dilution (150°C) stage, where the volatile concentration is reduced, followed by a tube heated to 300°C, where volatiles are evaporated, followed by a second diluter to prevent subsequent nucleation. Although the temperature of the evaporation tube is set at 300°C, the actual sample temperature can be lower than this value. Measurements of the PMP system used for this program indicated that the actual sample temperature was ∼200°C (CitationAyala et al. 2006). After the VPR, particles were then counted using a CPC. For this program, a TSI Model 3790, with a D50 size cut of 23 ± 3 nm, was used. A schematic of the PMP system is provided in the Supplemental Information.
2.2. Laboratory Descriptions
The laboratory testing for this project was conducted over a series of test cycles on a chassis dynamometer at the California Air Resources Board's (CARB's) heavy-duty vehicle emissions laboratory in Los Angeles and in conjunction with a larger CARB PM toxicity study (CitationBiswas et al. 2008).
The on-road testing was conducted using the University of California at Riverside's (UCR's) Mobile Emissions Laboratory (MEL). The MEL is a Class 8 trailer equipped with a full-flow dilution tunnel and gaseous and PM emissions sampling systems meeting regulatory requirements (CitationCocker et al. 2004a,Citationb; CitationCFR 2008). This allows the MEL to make measurements fully compliant with reference methods for emission certification under in-use driving and environmental conditions.
2.3. Test Vehicles, Fuels, and Lubricants
For the laboratory testing, a 1999 International 4900 medium-heavy-duty diesel vehicle with a 7.6 liter, 6 cylinder, 210 horsepower International DT466E engine was used. For the on-road testing, the test vehicle was UCR's Heavy-Heavy-Duty-Vehicle class 8 truck equipped with a 14.6 liter, 6 cylinder, 475 horsepower, 2000 Caterpillar C-15 engine. Both engines utilized direct fuel injection, turbocharging, and were certified to a 0.1 g/hp-h PM and 4.0 g/hp-h NO x standard. Both engines were retrofitted with passive DPFs that regenerate or burn off accumulated PM when exhaust temperatures are adequate. The 1999 International vehicle was retrofitted with an Engelhard DPX™ trap and the 2000 Caterpillar vehicle was retrofitted with a Johnson Matthey Continuously Regenerating Trap (CRT™). These passive DPFs behave differently than active DPFs, which regenerate periodically in discrete events and are being used by original equipment manufacturers (OEMs) on 2007 and newer diesel engines in the US. The 1999 International had a mileage of 40,000 miles and was loaded to 21,000 lbs to simulate a partial load in the medium heavy-duty weight class. The 2000 Caterpillar had a mileage of 18,000 miles and was loaded by the 65,000 lbs MEL trailer itself. All tests were conducted with CARB Ultra Low Sulfur Diesel (ULSD) fuel (S < 15 ppmw) and lubricating oil with typical sulfur levels in a range from 0.3 to 0.6%.
2.4. Test Cycles
The cycles selected for the laboratory testing on the 1999 International 4900 truck included the Urban Dynamometer Driving Schedule (UDDS), a 50 miles per hour (mph) steady state cruise mode, and an idle test. The UDDS was composited with two back-to-back cycles to be 35 min and the cruise and idle tests were each 45 min long. These times were selected to meet the requirements of the other separate, but coordinated CARB study (CitationBiswas et al. 2008).
Testing was performed on-road with the 2000 Caterpillar equipped truck using the UDDS, the cruise and urban segments of the European Transient Cycle (ETC), and the CARB creep cycles. A description of each of these standard cycles is provided in the Supplemental Information. In addition, tests were performed for vehicle operation in real-world, normal flow-of-traffic. The on-road test cycles were shorter in time than the laboratory cycles, with the UDDS, ETC cruise, ETC urban, and CARB creep cycles being 18, 10, 10, and 13 min long, respectively. Two flow-of-traffic conditions were used, which are denoted as Routes 1 and 2 in the figures below. The flow-of-traffic tests denoted Route 1 were conducted under freeway driving conditions that included long climbs in elevation where the engine was subjected to much higher loads than seen in the standard cycles. The flow-of-traffic tests denoted Route 2 were collected on a long arterial road with stops only about every 2 miles. The on-road testing was conducted on public roads and freeways in the Palm Springs area of California.
2.5. Particle Number Sampling
The solid particle number sampling for the laboratory and the on-road testing was conducted post the PMP system. The PMP-compliant, TSI Model 3790 CPC (23 nm D50 cut point) was used for the PMP particle number measurement. Additional CPCs with smaller cut points were used post the PMP system to provide information on particles in smaller size ranges that are not being counted under the current PMP protocol. For the laboratory tests, two TSI 3025A CPCs (3 nm D50 cut point) were used post the PMP. For the on-road testing, a TSI 3025A CPC and a 3760A CPC (11 nm D50 cut point) were used post the PMP system and a TSI 3022A CPC (7 nm D50 cut point) was used to sample particles directly from the CVS tunnel. Thus, the 3022A measures not only the particles defined to be solid under the PMP protocol, but also volatile particles that should be eliminated by the PMP system. and show the details of the sampling for the laboratory testing and the on-road testing, respectively.
FIG. 1 Flow diagram of PM sampling system from the primary dilution tunnel for laboratory tests. Solid and dotted lines show measurement from the primary and secondary dilution tunnel, respectively. Numbers within parentheses are D50 cut-off diameter of each of the instruments. The term “solid” is utilized in parenthesis for those CPCs measuring particles that are solid in accordance with the PMP definition. (a) Lab test (1999 International). (b) On-road test (2000 Caterpillar).
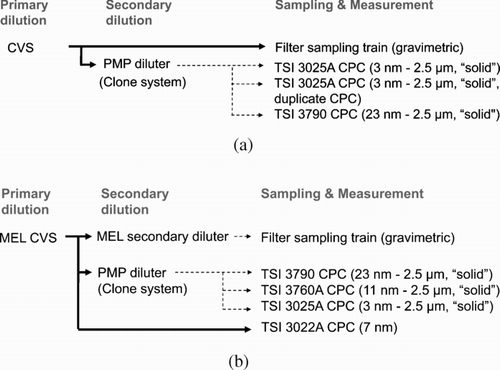
Tunnel blanks for particle number were performed on a daily basis post the PMP. CPC blanks averaged 0.31–0.33 #/cc for the 3790, and 0.65–0.71 #/cc for the 3025As, 0.37 #/cc for the 3760As. For the 3790 CPC, these tunnel blank values were slightly above the sensitivity level of 0.1 #/cc documented by TSI, and similar to values reported elsewhere (CitationAndersson et al. 2007a).
2.6. Particle Mass Sampling
The gravimetric PM mass measurements during laboratory and on-road testing were collected on 47 mm Teflo™ filters from a secondary dilution tunnel off the CVS tunnel. The gravimetric filter sampling conditions met CFR40 part 1065 procedures for sample residence time, dilution temperature, and filter face temperatures of 47 ± 5°C. However, the filter face velocities of 50 cc/s were lower than the recommended 100 cc/s.
Gravimetric tunnel blanks were collected throughout the course of testing, but were not subtracted when calculating emissions (CitationAndersson et al. 2007a). For the on-road testing, other filter blanks were collected to characterize possible contamination from transporting (trip blanks), loading (static blanks), and using filters in the sampling apparatus (dynamic blanks). Additional details about the PM mass blank levels are provided in the Results and Discussion Section.
2.7. Test Iterations
Laboratory and on-road standard test cycles were repeated at least seven times to fulfill the requirements as in the inter-laboratory correlation exercise (ILCE) for the PMP. For the laboratory tests, greater number of replicates were possible for the cruise (16 iterations) and UDDS (18 iterations) due to the sampling requirements for the associated CARB study (CitationBiswas et al. 2008). Fewer iterations were obtained for the on-road flow-of-traffic measurements and the laboratory idle tests (3 iterations).
3. RESULTS AND DISCUSSION
3.1. PM Mass Emissions
The average PM mass emissions on a per mile, per hour, and a brake specific basis are shown in for the laboratory and on-road tests, with error bars representing one standard deviation of the repeated tests. The brake specific PM mass emissions were obtained only for the on-road tests using engine speed, frictional torque, and actual torque from the engine control module (ECM) J1939 signal to determine horsepower (hp). The PM mass measurement results are all comparable in magnitude. For the laboratory and standard cycle on-road tests, the mass emission levels were all below 10 mg/mi, consistent with earlier studies for a properly functioning DPF (CitationHerner et al. 2007a; CitationKimura et al. 2004). PM emissions for the flow-of-traffic test (denoted Route 1) were higher at slightly over 26 mg/mi (6 mg/hp-h). The brake specific PM mass emissions were below the US 2007 certification standard of 10 mg/hp-h and are consistent with other engine studies (CitationKhalek 2005). The laboratory idle emissions are on a time basis and averaged 23 mg/h.
FIG. 2 PM mass result from laboratory and on-road testing. Note: The hatched bars represent brake specific mass emissions. Route 1 was composed of some hills and flat road conditions on California Interstate-10 Freeway. Only a single iteration was conducted over the on-road “Route 1,” so this value does not have an error bar.
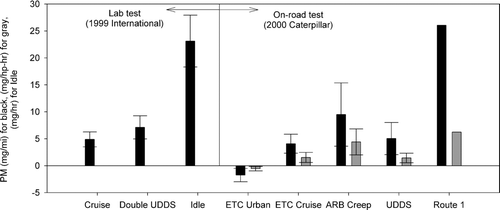
The collected filter masses depend on a number of factors, including system flow rates, operating parameters, and test cycle times. The filter weights for the on-road and laboratory testing are shown in for each standard cycle and the flow-of-traffic test. The on-road tests generally showed much lower filter weights than the laboratory tests due to shorter sampling times. In comparison with the FTP certification cycle (20 min), the on-road cycles were generally shorter (10–18 min) and the laboratory tests were longer (35–45 min). Typical filter weights for the on-road standard cycle testing ranged from slightly negative (i.e., just less than 0 μg) for the ETC Urban cycle to 7 μg on the ETC Cruise cycle. The highest on-road filter weight was for the flow-of-traffic measurement, where longer sampling times, higher sustained loads, and sustained exhaust temperatures >300°C were found. The laboratory filter weights ranged from 120 μg for the steady state cruise cycle to 50 μg for the double UDDS cycle. Only the filter weights for the laboratory idle (∼10 μg) were similar to those for the on-road standard cycle testing. It should be noted that some improvements in the PM mass detection limits are expected with the new CFR40 part 1065 requirements for 2007 (CitationCFR 2008). Increasing the face velocities from 50 cm/s, as used in this study, to 100 cm/s would have doubled the PM loading on filters.
Typical tunnel blanks for the on-road testing were ∼5 μg over 30 min or equivalent to 2 μg and 4 μg, respectively, over the cycle times for the ETC cycle (10 min) and the UDDS cycle (18 min). CE-CERT has also performed 4 h tunnel blanks with filter weights of 5 μg, suggesting the tunnel blank weight is associated more with filter handling and weighing accuracy than background PM. The masses of other types of blanks, including trip blanks, static blanks, and dynamic blanks, were typically within the repeatability limits for the microbalance reference filter, or ∼2–3 μg. The overall uncertainty in MEL's gravimetric PM filter measurements during this program was approximately 2.5 μg at one standard deviation or 7.5 μg at three standard deviations, as shown in . All of the on-road gravimetric filter mass measurements were either at or below 7.5 μg, with the exception of the one flow-of-traffic test and a single ETC test. The difficulty in making mass measurements at filter weights around and below 10 μg is reflected in the large error bars for the on-road standard cycle tests shown in . The detection limits for the filter mass for the laboratory testing were comparable to those for the on-road testing, although the filter masses for the laboratory tests were all well above these levels except for the idle tests. Tunnel blanks for the laboratory testing were collected periodically for the laboratory testing and ranged from 0 to 37 μg, with an average of 13 μg.
3.2. Particle Number Emissions by PMP Method
The average particle number emissions are shown in for both the laboratory and on-road tests, with the error bars representing one standard deviation of repeated test results. The data are shown on a logarithmic scale since the data for different testing conditions and CPCs varies over several orders of magnitude. The lower limit for the y-axis was selected to provide the best visualization of the data over the range of tests and instruments. Note that the error bars extend below the lower limit for the y-axis for the laboratory Cruise and UDDS tests, with the exception of the 3790 on the cruise, and for the on-road 3022A measurements for the UDDS, so they can not be adequately shown with the scale presented. However, the errors associated with the various testing conditions are discussed in greater detail below. For the laboratory idle test, only the 3022A was available for particle counts. For the higher cut point CPCs (3790 and 3760A), the emissions over the standard cycles are generally below the light duty vehicle European standard for particle number of 9.6 × 1011 #/mi for the on-road measurements, with the laboratory measurements about 7 to 19 times lower than this standard. Again, the reader is reminded that our tests are for heavy-duty engines and over different test cycles/conditions. The laboratory measurements agree better with levels measured for DPF-equipped light-duty diesel vehicles in the PMP light-duty (LD-ILCE), which were generally below the 2 × 1011 #/km (3.2 × 1011 #/mi) level (CitationAndersson et al. 2007a; CitationZhang et al. 2008). The laboratory 3790 CPC number emissions averaged 1.4 × 1011 #/mi for the UDDS and 0.5 × 1011 #/mi for the cruise. The on-road average particle number emissions for the standard cycles varied from 2.6 × 1011 to 6.7 × 1011 #/mi for the ETC cruise and ARB creep cycles, respectively.
FIG. 4 Particle Number Counts per mile for lab and on-road tests for HD trucks. Note: ' Without outliers. Route 2 is a transient driving which is composed of flat road driving with 5 stops in a 10 min stretch). For Route 1, the 3025A and 3022A concentrations were both saturated and hence the values represent absolute lower bounds. Also, the 3760 concentrations were above the maximum stated range for the instruments. *3022A sampled at CVS while other CPCs sampled under PMP dilution system. **This 3025A is a duplicate 3025A CPC for the laboratory testing. The laboratory idle test was integrated over the entire test period, and as such does not have an error bar.
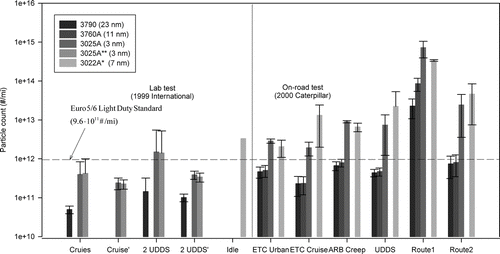
For heavy-duty engines, emissions values are typically reported on a brake specific basis because of the variability of the load carried by the vehicle. The brake specific 3790 CPC number emissions (not shown) ranged from approximately 0.76 × 1011 #/hp-h for the ETC cruise to 2.9 × 1011 #/hp-h for the creep cycle. These are comparable to or slightly below values measured in studies in Europe, which showed levels around 3–4 × 1011 #/hp-h for different test cycles (CitationBosteels et al. 2007).
Particle counts for the higher load flow-of-traffic driving conditions, denoted Route 1, were considerably higher than those for the standard cycles, as seen in . In fact, for these tests the CPCs either saturated at the maximum count rate or reached concentrations above their maximum stated range, thus these values are absolute lower bounds. The flow-of-traffic tests were associated with higher engine loads, and hence, sustained exhaust temperatures of 300°C or more. Size distributions collected during these tests showed that the particles were primarily in the nucleation mode (see CitationDurbin et al. 2008). Sulfate measurements using ion chromatography for one test showed that approximately half of the mass was sulfate related, indicating that the nucleation mode particles may be attributed to the conversion of SO2 to SO3, which is likely aided by the Pt-catalytic coating on the DPF. This is consistent with results from CitationKittelson et al. (2006) who found that the formation of nucleation particles downstream of a CRT™ increased dramatically at temperatures >300°C. Sulfate also comprised a significant PM mass fraction for the laboratory tests when exhaust temperatures were >300°C and nucleation was observed (CitationHerner et al. 2007b) and under similar conditions to other studies (CitationGrose et al. 2006).
The particle number measurements for the 3790 and the 3760A CPCs were about an order of magnitude lower than those for the 3022A or the 3025A for the on-road tests, suggesting the presence of sub-23 nm “solid” particles out of the PMP sampling system and solid and volatile particles in the CVS. CitationKasper (2004) reported that at the temperatures and residence time used, the PMP VPR system removed 90–98% of the volatile nucleation mode particles downstream of a catalyzed DPF. Whenever there is a large nucleation mode indicated by a high 3022A count, the 3790 count is 10% or less of the 3025A count. Thus, much of the apparent PMP solid particle count could simply be breakthrough of the VPR system and not true solid particles. This breakthrough could result from either incomplete evaporation, evaporation followed by renucleation, or particles composed of sulfuric acid and hydrocarbons could pyrolyze into solid-like particles (CitationArnold et al. 2008) as they pass through the PMP. CitationAyala et al. (2006) reported that the actual sample temperature in the evaporation section of the VPR for the PMP used in this study was closer to 200°C, which would allow some hydrocarbon and volatile species to survive through the PMP. “Solid” nanoparticles (<23 nm) getting past the PMP VPR were also observed by CitationHerner et al. (2007a). CitationDeFilippo and Maricq (2008) also found nuclei particles that remained nonvolatile to >400°C at idle and vehicle speeds below ∼30 mph, although these particles were removed with an efficiency comparable to soot particles by a DPF. The laboratory tests showed some trends of lower mean particle number emissions for the 3790 compared to the 3025A, but these comparisons were complicated by the variability of the measurements.
For some on-road driving cycles, the 3022A CPC, which was counting particles directly from the CVS, measured particles that are not seen post the PMP system. During the ETC cruise, the 3022A CPC (7 nm) measured higher particle counts than the PMP diluted 3025A CPC (3 nm). For this cycle, size distribution measurements showed that a majority of the particles in the primary dilution tunnel were nucleation particles (see CitationDurbin et al. 2008). These nucleation particles are typically volatile and get eliminated by the PMP system before being measured by the 3025A, as expected. Nucleation events were also identified in the on-road UDDS test cycles, but the comparisons between the 3022A and the 3025A CPCs did not show significant differences outside the variability of the testing. For the lighter load creep and ETC urban cycles, in contrast, size distributions showed that nucleation mode particles were not formed (see CitationDurbin et al. 2008). For these cycles, the 3022A and the 3025A CPCs measured similar values, consistent with most particles being solid in nature and measured by the PMP system. These results are still puzzling, however, in that the 3790 and 3760A CPC counts are about an order of magnitude lower than those of the 3025A and 3022A CPCs. This suggests that most of these “solid” particles are smaller than 11 nm. This is inconsistent with filtration theory which would suggest that such particles are very efficiently removed by diffusion. More work will be necessary to understand these inconsistencies.
3.3. Measurement Repeatability for Particle Mass and Particle Number
shows the coefficients of variation (COVs) for particle number and PM mass for all standardized cycles for both the on-road and laboratory tests and for the laboratory tests with the outliers removed. The on-road PM mass showed much higher COVs compared to 3790 number based COVs, except during the ETC Cruise cycle. Most of the on-road PM mass variability is due to the low filter weights. As discussed above, the PM mass filter loading and associated COVs depends on a number of factors including flow rates and system operating parameters, and cycle duration and load. The COV for the 3022A that sampled from the primary CVS was higher than those for the CPCs post the PMP system for nearly all on-road standard cycles. This can be attributed to variability in volatile particles eliminated by the PMP, especially for the UDDS and cruise cycles.
FIG. 5 Coefficients of Variation. Note: * 3022A sampled from CVS while other CPCs sampled under PMP diluter. *** Negative value.’ Without outliers.
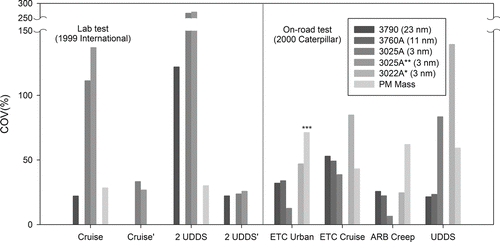
Particle number measurements for the laboratory testing showed larger variability for the different instrument/test cycles. This variability can be attributed to outlier test results for both the cruise and the UDDS cycles with much higher counts than the others in the sets, as presented in the Supplemental Information. One key observation is that the outlier events appear to be real differences in particle number emissions. The real-time emission results provide insight into the outlier tests and are included in the Supplemental Information. The real-time results showed that the concentrations for the 3025A CPCs exhibited several spikes up to the 4,000 to 100,000 particles/cc level below the PMP, as opposed to typical levels that were below 100 particles/cc. These concentration spikes for the outlier tests were also typically observed by both 3025As post the PMP, and the 3790 for some points. These particle count levels are much higher than any reported by others or for other studies in the same laboratory (CitationHerner et al. 2007a), and at this point we cannot fully explain these occurrences. Phenomena such as deposition, storage and release, and regeneration are known to impact PM emissions from DPFs. Swanson et al. (2009) observed large spikes in particle number concentration downstream of new and aged CRT™s when the temperatures were below 380°C. In both cases, the spiking did not occur in a discrete forced regeneration but rather during continuous regeneration. They hypothesized the spikes were associated with local hotspots and the soot deposits breaking up. The spikes observed in the present study also were not associated with a discrete regeneration event since the DPF is continuously regenerating at the exhaust temperature levels when the spikes occurred.
The highest variability for the laboratory tests was seen for the 3025A CPCs with the lower size cut point (3 nm), although outlier tests were also found for the PMP-compliant 3790 (23 nm) for the UDDS. The COV for the 3790 measurements was comparable to that of PM mass for the cruise, but much greater than that of PM mass for the UDDS at ∼120%. The 3790 COV for the UDDS improved to 22% with its one outlier point removed. The 3790 COV data with the outlier removed is in the same range of 24–59% reported for the intra-lab variance for the ILCE_LD round robin (CitationGiechaskiel et al. 2008). The COVs of the lower cut point 3025As were all greater than that of the PM mass for the laboratory testing and ranged from 110 to 270%. The COVs for the laboratory low cut point CPCs improved considerably when the two of the highest outlier points removed, however, to levels of approximately 24–33%. The improvement of the measurement repeatability with the removal of outliers suggests that consideration of statistical methods for dealing with outliers may be useful to the extent that other laboratories encounter the issue or until further studies help us determine the nature of these events.
4. CONCLUSIONS
The objective of this study was to evaluate the European PMP protocol for the measurement of solid particle number under laboratory and on-road conditions. Under the PMP operational definition, “solid” particles are defined as ones having a diameter between 23 nm and 2.5 μm and of sufficiently low volatility to survive a residence time of 0.2 seconds at 300°C. Laboratory tests were performed on a chassis dynamometer over a UDDS cycle, a 50 mph cruise, and an idle. On-road testing was added to evaluate the effectiveness of the PMP under real-world conditions. The on-road tests were conducted over cycles such as the UDDS, the urban and motorway segments of the European ETC cycle, and the CARB creep cycle, as well as typical on-road highway conditions. The medium-duty vehicle and heavy-heavy-duty vehicle used for the laboratory and on-road testing, respectively, were each retrofitted with passive DPFs. Testing was conducted using a PMP-prescribed rotating disk diluter system with a 23 nm cut point 3790 CPC and additional CPCs with cut points of 3, 7, and 11 nms. Our principal observations are as follows:
-
The passive, retrofit, DPF-equipped medium-duty and heavy-heavy-duty-vehicle PMP number emissions based on 3790 CPC measurements were lower than the European light duty standard of 9.6 × 1011 #/mi. The emissions ranged from 0.5 × 1011 to 6.7 × 1011 #/mi depending on the cycle, vehicle/technology, and other test conditions used. The brake specific 3790 CPC number emissions ranged from approximately 0.76 × 1011 #/hp h to 2.9 × 1011 #/hp h for the on-road testing.
-
For the on-road tests, the COVs for the particle counts were generally lower than those for the PM mass measurements, with the exception of the ETC Cruise and some of the measurements with the lower cut point CPCs. For the laboratory tests, however, outliers were found on both the 50 mph cruise and the UDDS. The outlier events in particle number emissions appear to be real differences that can be seen with the PMP system but are not apparent in the mass measurement. In general, the COVs for the PM mass depended on filter loading and cycle duration, while the COVs for particle number depended on the presence of outliers. Further testing of additional engines/vehicles and engines/vehicles equipped with active DPFs could better define the range of variability in particle number measurements that might be seen over a larger fleet.
-
Significant nucleation was observed under conditions with high engine loads and sustained exhaust temperatures of 300°C or more for these vehicles equipped with passive DPFs. For the highest load on-road testing conditions, this included very high count levels post the PMP system. These nucleation particles had a large sulfate contribution that is probably due to the conversion of SO2 to SO3. The association of very high counts post the PMP system with the formation of high concentration volatile sulfate particles in the dilution tunnel raises questions about calling particles post the PMP solid, at least under high load conditions. This phenomena should also be studied for engines with active DPFs that regenerate in discrete events rather than as a function of exhaust temperature.
-
The particle number measurements for the low cut point CPCs post the PMP system were approximately an order of magnitude higher than those for the PMP-compliant CPC and the other high cut point CPCs post the PMP system. This indicates the presence of a significant fraction of sub-23nm particles that are not being counted by the PMP approach. The recommended PMP practice with a >23 nm cut point has the advantage of removing nucleation particles that can contribute to variability, but also removes the ability to characterize very small particles that survive heating in the VPR. The lower cut point CPCs showed greater variability than the higher cut point CPCs in the laboratory testing, but not the on-road testing.
The California Air Resources Board funded this work under contract No. 05-320. The authors acknowledge Dr. Marcus Kasper of Matter Engineering Inc., Mr. Jon Andersson of Ricardo, and Mr. Andreas Mayer for their assistance in planning, experimental preparation, and data analysis. We acknowledge Mr. Donald Pacocha of UCR for his assistance with the on-road testing. CARB investigators acknowledge cooperation with the Directorate General—Joint Research Center of the European Commission under a Memorandum of Understanding.
REFERENCES
- Andersson , J. , Giechaskiel , B. , Munoz-Bueno , R. and Dilara , P. 2007a . Particle Measurement Programme (PMP) Light-Duty Inter-Laboratory Correlation Exercise (ILCE_LD) Final Report No. GRPE-PMP-18-2. 18th PMP working meeting, January.
- Andersson , J. , Clarke , D. and Giechaskiel , B. 2007b . UN-GRPE Phase 3 Inter-Laboratory Correlation Exercise: Updated Framework and Laboratory Guide for Heavy-Duty Engine Testing. Working Paper No. GRPE-PMP-22-4. 22nd PMP working meeting, December.
- Arnold , F. Reichl , U. 2008 . “ Nanoparticle Formation in Diesel Vehicle Exhaust: First Measurements of Precursor Gases ” . Zürich, , Switzerland : 12th ETH Conference on Combustion Generated Nanoparticles .
- Ayala , A. , Herner , J. and Huai , T. 2006 . “ California's Efforts for Advancing Ultrafine Particle Number Measurements for Clean Diesel Exhaust ” . Proceedings of the 12th Diesel Engine Emissions Reduction Conference, Detroit, Michigan, August.
- Biswas , S. , Hu , S. , Verma , V. , Herner , J. D. , Robertson , W. H. , Ayala , A. and Sioutas , C. 2008 . Atmos. Environ. , 42 : 5622 – 5634 .
- Bosteels , D , May , J. , Nicol , A. J. , Such , C. H. , Andersson , J. D. and Sellers , R. D. 2007 . “ Investigation of the Feasibility of Achieving Euro VI Heavy-Duty Diesel Emissions Limits by Advanced Emissions Controls ” . Presentation of the Association of Emissions Control by Catalyst website. www.aecc.be. September.
- Cocker , D. R. III , Shah , S. D. , Johnson , K. , Miller , J. W. and Norbeck , J. M. 2004a . Development and application of a mobile laboratory for measuring emissions from diesel engines. 1. Regulated gaseous emissions. . Environ. Sci. Technol. , 38 : 2182 – 2189 .
- Cocker , D. R. III , Shah , S. D. , Johnson , K. , Zhu , X. , Miller , J. W. and Norbeck , J. M. 2004b . Development and application of a mobile laboratory for measuring emissions from diesel engines. 2. Sampling for toxics and particulate matter. . Environ. Sci. Technol. , 38 : 6809 – 6816 .
- Code of Federal Regulations . 2008 . “ Title 40, Part 1065, and Title 40 sections 86 and 89 for earlier testing ” .
- DeFilippo , A. and Maricq , M. M. 2008 . Diesel nucleation mode particles: Semivolatile or solid? . Environ. Sci. Technol. , 42 ( 21 ) : 7957 – 7962 .
- Durbin , T. , Heejung , J. , Cocker , D. R. III , Johnson , K. and Chaudhary , A. 2008 . “ Evaluation of the Proposed New European Methodology for Determination of Particle Number Emissions and its Potential in California for In-Use Screening ” . Final Report to CARB.
- Giechaskiel , B. , Ilara , P. and Andersson , J. 2008 . Particle Measurement Programme (PMP) light-duty inter-laboratory exercise: Repeatability and reproducibility of the particle number method. . Aero. Sci. Technol. , 42 : 528
- Grose , M. , Sakurai , H. , Savstrom , J. , Stolzenburg , M. R. , Watts , W. F. Jr. , Morgan , C. G. , Murray , I. P. , Twigg , M. V. , Kittelson , D. B. and McMurry , P. H. 2006 . Chemical and physical properties of ultrafine diesel exhaust particles sampled downstream of a catalytic trap. . Environ. Sci. Technol. , 40 : 5502 – 5507 .
- Herner , J. D. , Robertson , W. H. and Ayala , A. 2007a . SAE technical paper 2007-01-1114.
- Herner , J. D. , Ayala , A. , Robertson , W. H. , Chang , O. , Biswas , S. and Sioutas , C. 2007b . “ Nucleation Mode Particle Emissions from In-Use Heavy Duty Vehicles Equipped with DPF and SCR Retrofits ” . Reno, NV : American Association of Aerosol Research, platform presentation . September 24–28, 2007.
- Kasper , M. 2003 . SAE Technical Paper No. 2003-01-0286.
- Kasper , M. 2004 . SAE Technical Paper No. 2004-01-0960.
- Kasper , M. 2008 . SAE Technical Paper No. 2008-01-1177.
- Khalek , I. A. 2005 . “ 2007 Diesel Particle Measurement Research ” . SwRI Final Report No. 03.10415 to the Coordinating Research Council under project E-66-Phase 1, May.
- Kittelson , D. B. Watts , W. F. 2006 . On-road evaluation of two diesel exhaust aftertreatment devices. . J. Aerosol Sci. , 37 : 1140 – 1151 .
- Kimura , K. , Alleman , T. L. , Chatterjee , S. and Hallstrom , K. 2004 . SAE Technical Paper No. 2004-01-0079.
- Swanson , J. J. , Kittelson , D. B. , Watts , W. F. , Gladis , D. D. and Twigg , M. V. 2008 . Influence of Storage and Release on Particle Emissions from New and Used CRT™s submitted to . Atmos. Environ ,
- Zervas , E. Dorlhene , P. 2005 . Test of exhaust PM using ELPI. . Aerosol Sci. Technol. , 39 : 333 – 346 .
- Zhang , S. 2008 . “ Final Results from CARB's Evaluation Testing of the European Reference Vehicle from the Particulate Measurement Program ” . Presentation at the 18th CRC On-Road Emissions Workshop, March–April, San Diego, CA.