Abstract
The use of respiratory tract dosimetry predictions to estimate the desired aerosol exposure concentration required for a specific target deposited dose in animal inhalation studies has been hindered by: (1) a lack of species/strain specific quantitative respiratory tract anatomy; and (2) verification by comparison of calculated and experimentally measured deposited doses. Using recent literature on tracheobronchial and pulmonary respiratory tract anatomy, dosimetry predictions for the Balb/c mouse were compared with deposited doses for 0.5, 1.0, and 2.0 micrometer diameter aerosols in a newly available nose-only exposure system. Spatial and temporal aerosol port to port uniformity of this nose-only exposure system was within ± 10% of the mean. Dosimetry predictions were in agreement with the measured mean deposited doses for the aerosols tested.
INTRODUCTION
Predictions of respiratory tract dosimetry have been used extensively for risk assessment and hazard analysis of airborne particulate matter. Another use of respiratory tract dosimetry predictions is to estimate the desired aerosol exposure concentration required for a specific target deposited dose in inhalation studies of particulate aerosols. One of the difficulties in performing these predictions is the lack of quantitative respiratory tract anatomy that is required for accurate dosimetry predictions. Even when the necessary anatomical information is available, the dosimetry predictions should be verified by comparison with experimentally measured deposited doses.
Most animal inhalation studies quantify the exposure concentration; however, there are relatively few that quantitatively measure respiratory tract deposition (CitationRaabe et al. 1988; CitationYeh et al. 1990; CitationNadithe et al. 2003; CitationHofstetter et al. 2004; CitationOldham et al. 2004; CitationWichers et al. 2006; CitationAlessandrini et al. 2008). A few of these studies have attempted to verify dosimetry model predictions. CitationNadithe et al. (2003) designed a nose-only exposure system and used a proprietary dosimetry code to compare experimental and predicted particle deposition for 3.9 (±0.5) micrometer diameter particles. CitationWichers et al. (2006) compared experimentally measured deposition with predictions from the MPPD dosimetry code (CitationMPPD 2002) for 1.95 micrometer diameter particles. Neither of these studies were able to utilize strain specific respiratory tract anatomy and respiratory physiology parameters. In a study by CitationNadithe et al. (2003) C57BL/6 mice were exposed but the dosimetry modeling used a combination of CD-1 and B6C3F1 mouse respiratory data. Their dosimetry predictions did not differ significantly from their in-vivo measurements. CitationWichers et al. (2006) exposed spontaneously hypertensive rats and their dosimetry predictions used Long Evans Hooded rat anatomy with their spontaneously hypertensive rat respiratory physiology. Their dosimetry predictions were <33% of their measured dose.
The purpose of this study was to determine if dosimetry predictions using a computer code (CitationNCRP 1997) based upon a tracheobronchial and pulmonary model for the Balb/c mouse (CitationOldham and Robinson 2007) were predictive of measured tracheobronchial and pulmonary deposition in newly available nose-only exposure system (TSE Systems, GmbH, Bad Homburg). Before performing the mouse aerosol exposure study, the aerosol spatial and temporal uniformity of the nose-only exposure system was determined as a function of system flowrate.
MATERIALS AND METHODS
Nose-Only Exposure System
The TSE Systems, GmbH exposure system used in this study is a stainless steel double plenum (inner plenum aerosol supply, outer plenum exhaled air) modular designed multilevel nose-only exposure system ( and ). Each of the three levels had eight animal exposure ports and two sampling ports. This exposure system is software controlled, compliant with the U.S. Food and Drug Administration Good Laboratory Practice regulations (21 CFR 58) and only requires sufficient external electrical power, pressure, and vacuum to function. Since this is a push-pull system, the animal exposure tubes are sealed making them suitable for hazardous aerosol exposures. Computer controlled mass flow meters control nebulizer and dilution air, and the chamber airflow. For user protection a pressure transducer continuously monitors the pressure differential between the outer plenum and ambient conditions and alerts the user and/or shuts the system down if the pressure differential exceeds preset limits. Temperature, humidity, O2, CO2, as well as, particle counts (via an optical particle counter) were continuously monitored within the inner plenum. The exposure tube adapter used a nose-directed aerosol flow approach (CitationPauluhn 2003; CitationMoss et al. 2006) similar to other nose-only exposure systems except that the tube directing flow from the inner plenum extends well into the exposure tube (, view A). The internal diameter of this tube is 0.5 cm which results in exposure tube inlet velocities of 17.7 and 106 cm/s for system flow rates of 5 and 30 l/min, respectively. The exposure tube adapters supplied were made of nonconductive polyoxymethylene and utilized a double “O-ring” seal for connection to the polymethlymethacrylate exposure tubes.
FIG. 2 Schematic (not to scale) of the TSE Exposure System: A—exposure tube adapter detail; B—mass flow controllers for nebulizer and dilution air; C—nebulizer; D—nebulizer drain; E—“O”-ring sealed exposure tube; F—filter protected O2 & CO2 probe with pump; G—mass flow metered filter sample; H—Pressure tap on outer plenum; I—Optical particle counter; J—temperature and humidity probe; K—optional connection for second optical particle counter or other monitor device; L—outer plenum connection to vacuum pump; M—carbon and HEPA filter mass flow controlled system exhaust.
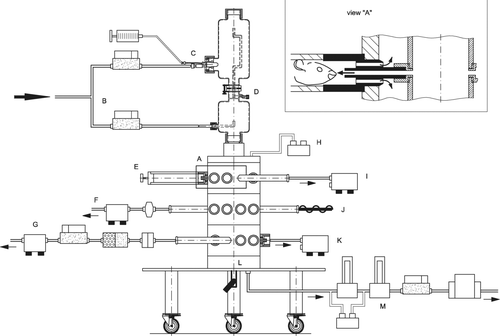
Port Uniformity Measurements
The animal exposure ports were numbered and the sampling ports were also identified to be able to track port specific results (). The exposure system was set up according to the manufacturer's instructions, so that half of the air flow occurred through the nebulizer and half as dilution air within the nebulizer module. Unfiltered laboratory air from the room where the exposure system was located was used to provide ambient aerosols for these measurements. Since our evaluation was focused on mice, only three total system flowrates were evaluated: 10, 20, and 30 l/min. To simulate nebulizer produced humidity, tap water was used and pumped into the nebulizer at 10 ml/h by the syringe pump. Temperature, relative humidity, O2, and CO2 were monitored during these experiments to provide guidance for the future animal exposures. Particles were simultaneously counted in six different size ranges (0.1–0.2; 0.2–0.3; 0.3–0.5, 0.5–1.0; 1.0–5.0, and >5.0 μm) using a recently calibrated Climet 7600 optical particle counter (Climet Instruments, Redlands, CA) that sampled at 85 cc/min. This particle counter was selected because its sampling flowrate was between the predicted (21 cc/min for a resting mouse; CitationGuyton 1947) and measured minute volumes using single (unrestrained) and double chamber (restrained) plethysmography (66–125 cc/min; CitationDeLorme and Moss 2002) for female Balb/c mice. A 3/8” stainless tube was sealed in the center of an exposure tube and connected to the Climet 7600 using flexible conductive tubing (Bev-A-XXX, Cole Palmer). Sampling time was set at 45 s with a 15 s delay between samples. When the exposure tube was randomly moved to a new exposure port there was a 2 min delay before sampling started. For each experiment, particle number measurements were repeated 3 or 4 times resulting in the standard error of the mean usually less than 10% of the mean value for each experiment. Experiments were conducted over a two-hour time period so the temporal stability of the system could be assessed by repeated measurements at selected ports.
Deposition Measurements
Tracheobronchial and pulmonary deposition was measured in 24 male Balb/c mice (mean body mass = 21.5 g) using three sizes (0.5, 1.0, 2.0 μm nominal diameters) of fluorescent monodisperse polystyrene latex (PSL) particles (Duke Scientific, Palo Alto, CA). The mass median aerodynamic diameters of the PSL particles were calculated using the size and density data provided by the supplier; geometric diameter was verified using light microscopy in our laboratory. Particle suspensions were diluted so that no more than 5% of the liquid droplets would contain PSL doublets (CitationRaabe 1968). The suspension was placed in an ultrasonic water bath and sonicated for 45 minutes immediately prior to being placed into a 60 cc syringe for infusion into the nebulizer by a syringe pump at 10 ml/h. The exposure system was set up for a total flowrate of 30 l/min with half the air flow in the nebulizer and half as dilution air within the nebulizer module. The system was equilibrated for 20 min (without turning on the syringe pump) prior to loading the animals into the exposure system. The aerosol was sampled using a (0.22 μm diameter pore) 47 mm diameter polycarbonate filter (Whatman International, Ltd., Maidstone England) in a stainless steel filter holder connected at sampling port E (). Flow (85 cc/min) was controlled by the Climet 7600 optical particle counter, which also monitored for leaks and filter breakthrough. To quantitate the number of fluorescent particles on the filter a cell-counter hemacytometer (CitationBhalla 1997) was used with an ultraviolet equipped microscope (Fluorphot, Nikon, Japan). The filter sample was sonicated for 1 h prior to counting the particles. Complete removal of fluorescent PSL from the filter was verified using fluorescent microscopy.
Particle losses on the exposure tube adapters () were determined by sonicating each adapter for 20 min in approximately 500 ml of soapy water followed by vacuum filtering the resulting suspension through (0.22 μm pore diameter) 47 mm diameter polycarbonate filters. This procedure was repeated twice in an attempt to ensure complete particle removal. Particles were counted using fluorescent microscopy.
Animals
All procedures involving Balb/c mice were reviewed and approved by the Institutional Animal Care and Use Committee at the University of California, Irvine. Animals were housed in isolator cages on a 12 h light/dark cycle and provided with food and water ad libitum prior to the 30 min exposure period. Immediately after the exposure the animals were euthanized i.p. with a lethal dose of sodium pentobarbital (120 mg/kg). Body mass was measured and the upper trachea (just below the larynx) was tied shut before opening the chest and removing the lung. Upon removal each lung was placed into a 50 ml centrifuge tube with 20 ml of approximately 6M NaOH to dissolve the tissue. After the lung tissue dissolved (12 h), the suspension was vacuum filtered through 0.22 μm pore 47 mm diameter polycarbonate filters. To ensure particle removal each tube was washed three times with 25 ml of distilled water. For each wash the tube was placed in an ultrasonic water bath and sonicated for 15 min prior to filtration of the wash. The fluorescent monodisperse particles on the polycarbonate filters were counted using fluorescent microscopy. Deposition efficiency was calculated by dividing the number of particles from each lung by the estimated number of particles inhaled by the mouse, which was determined using predicted (CitationGuyton 1947) and measured minute volumes (CitationDeLorme and Moss 2002) and the sampling filter data. The time period between the end of the exposure and tying the trachea shut was recorded for each mouse to see if these potential differences in particle clearance times affected the calculated deposition efficiency.
Deposition Predictions
The UCI typical path tracheobronchial and pulmonary airway model (CitationOldham and Robinson 2007) together with predicted and measured minute ventilation values for Balb/c mice were used in the NCRP (CitationNCRP 1997) dosimetry code to calculate predicted deposition efficiencies. Two ventilation values were used: (1) predictions of CitationGuyton (1947) based upon body mass; and (2) those measured in single and double plethysmographs (CitationDeLorme and Moss 2002). Since predictive extrathoracic airway (nasal, oral, pharyngeal, and larynx) deposition models for mice have not been developed, predicted deposition in these regions using the approach of CitationOldham et al. (1994), were performed. This approach used the experimental data for the CF1 mouse of CitationRaabe et al. (1988). The experimental data was corrected to account for some of the deposition found in the G.I. region due to particle clearance (CitationOldham et al. 1994) and also, the particle diameters were converted from the reported aerodynamic resistance diameter (CitationRaabe et al. 1988) to aerodynamic equivalent diameter using the method of CitationRaabe (1976). Particle inhalability, the fraction of particles in the exposure system which are actually inhaled, was calculated based upon the equation of CitationMenache et al. (1995). Predicted deposition efficiency was calculated as a percentage of the mass of unit density spheres entering the nose.
RESULTS
Nose-Only Exposure System
The nose-only exposure system functioned well. Airflows, temperature, O2, CO2, and the pressure differential between the outer plenum and the ambient room were stable throughout all experiments. Relative humidity became stable within 10 min of the start of nebulization. Because animal exposures were going to be conducted, test of the effectiveness of the software to prevent over pressurization and vacuum conditions that may affect respiratory parameters were performed. The default software alarm settings prevented any malfunction and unrealistic exposure conditions that could cause over pressurization or vacuum conditions (>0.2 mbar). Even intentional disconnection of the vacuum, air supply, particulate sampling, O2 and CO2 lines caused an immediate safe system shutdown and alarms to notify the user.
Port Uniformity Measurements
Four experiments were conducted at a total system flowrate of 10 l/min. Two of these runs sampled every port while the other two runs sampled 15 of the 24 ports. In addition, two experiments that sampled every port were conducted for the 20 and 30 l/min flowrates. Because of low particle counts in some size ranges (>1 micrometer diameter), reliable data for all of the ranges could not be obtained. When all of the particle size ranges counted were combined, each individual port was within 10% of the mean value for all 24 ports regardless of the flowrate tested (). It is important to note that the observed variability in particle counts is influenced by combined variability in: (1) the laboratory air used in these experiments; (2) exposure system; and (3) the particle counter over the two hour time period of the experiment. Repeated measurements of selected ports (all particle size ranges were combined) throughout each experiment resulted in <5% variation in total particle counts.
FIG. 3 Port uniformity data expressed as mean and standard error of all particles counted (0.1 ⩾ 5.0 micrometers) for each port as a percentage of the mean value for all 24 ports: (a) Four experiments conducted at 10 l/min.; (b) Two experiments conducted at 20 l/min.; (c) Two experiments conducted at 30 l/min. The error bars represent the standard error.
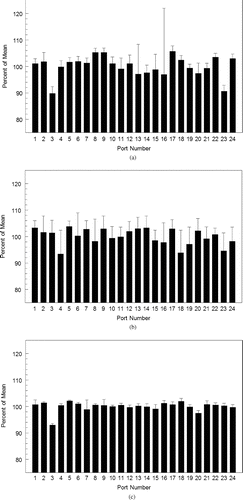
Deposition Measurements
The fluorescent particles were counted on the sampling filter to calculate an exposure concentration generated by the nebulizer module and from the mouse lungs (). Deposition efficiency for each level (top, middle, and bottom) of the exposure system was determined after 30 min of nose-only exposure () using calculated resting minute ventilation (CitationGuyton 1947) and measured Balb/c mouse minute volumes (CitationDeLorme and Moss 2002), the sampling filter and calculated inhalability values () from CitationMenache et al. (1995). Three mice were removed from the analysis because they exhibited respiratory distress requiring technician intervention as a result of repeated attempts of the mice to turn around in the exposure tubes. Deposition results of three additional mice were not included because the polycarbonate filter tore during filtration of their digested lung tissue. There was no relationship between deposition efficiency and the time required to tie shut the trachea (data not shown). Particle losses on 21 of the 24 exposure tube adapters were measured (). Particle losses on the exposure tube adapter from the three animals removed from the deposition analysis were not performed.
TABLE 1 Exposure concentration, number of fluorescent particles deposited in the lungs of Balb/c and calculated inhalability as a function of particle diameter
TABLE 2 Deposition efficiency in Balb/c mice as a function of particle diameter and level of the exposure system using calculated CitationGuyton (1947) and measured minute volumes (CitationDeLorme and Moss 2002)
TABLE 3 Mean particle deposition efficiency (percent) on the exposure tube adapters
Deposition Predictions
Predicted inspiratory and expiratory tracheobronchial and pulmonary deposition efficiency () using calculated (25 ml) and measured (125 ml) minute volumes were performed using the UCI typical path Balb/c lung model (CitationOldham and Robinson 2007). The mean deposition efficiency (± standard error) using the calculated minute volume (CitationGuyton 1947) and the experimentally measured deposition efficiency for CD-1 mice by CitationRaabe et al. (1988) are also plotted for comparison.
FIG. 4 Comparison of predicted inspiratory and expiratory deposition efficiency using the Balb/c mouse lung model for tracheobronchial and pulmonary regions for minute ventilations of 25 (•) and 125 (█) ml, the CF1 in-vivo data (▴) of CitationRaabe et al. 1988, the C56BL/6 data (○) of Nadithe et al. 2003 and the mean deposition efficiency from this study (⧫) using the equation of CitationGuyton (1947) for minute volume (∼25 ml). Error bars are standard deviations and in some cases were smaller than the symbol used to plot the point.
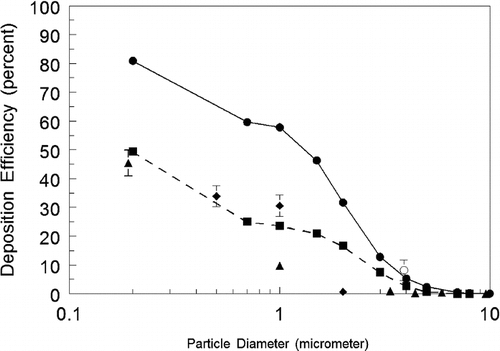
DISCUSSION
Unlike CitationCheng et al. (1989) and CitationO'Shaughnessy et al. (2003) we assumed that spatial and temporal aerosol uniformity within the nose-only exposure system were dependent on each other. Each spatial uniformity experiment required 2 h to complete and repeated measurements of selected ports throughout this time period indicated <5% variation, which suggests good temporal aerosol uniformity in the nose-only exposure system. However, since there were low numbers of particles above 1 μm in these studies, the upper particle size limit of this uniformity is not known. The spatial uniformity of the TSE exposure system was good and is likely better than the ±10% reported because the variability of the laboratory air and optical particle counter were not factored out of the calculation. In reviews of aerosol exposure chambers (CitationMacFarland 1983; CitationPhalen 1997; CitationTillery et al. 1976; CitationCheng and Moss 1995; CitationPhalen 2009), whole body chamber uniformity (ranging from ±2.1 to ±12.8) has varied depending on the tracer gas used (CitationMacFarland 1983) and as would be expected, vary as function of particle size (CitationYeh et al. 1986). For nose-only exposure systems, uniformity is typically better than ±10%. The particle losses on the exposure tube adapters, although not excessive, could probably be reduced by substituting a conductive material for the nonconductive polyoxymethylene.
The overall measured particle deposition efficiency () for two of the three particle sizes was consistent with the CD-1 mouse deposition data of CitationRaabe et al. 1988 using Guyton's resting minute ventilation. However, the particle deposition efficiency for the 2.0 μm particles was not consistent with either the CD-1 mouse deposition data of CitationRaabe et al. (1988) or the C57BL/6 mouse data of CitationNadithe et al. (2003). The variability among the top, middle, and bottom level of the exposure system for 1 μm particles was also greater than expected. Some of this variability could be due to the short exposure duration, which would tend to highlight differences in minute volume among animals. For example, CitationDeLorme and Moss (2002) measured a wide range of minute volumes (59–135) for 20 gm female Balb/c mice using two exposure methods. The resting minute ventilation calculation (21 ml) based upon the measurements of CitationGuyton (1947) further highlights the potential variability in animal minute ventilation. Additionally, particle deposition variability may be due to the design of the exposure tube adapter (, view A). The aerosol entrance tube of this adapter extends into the exposure tube. There were chew marks on a majority of the adapters after the exposure. Any chewing that resulted in an increase in resistance or temporary obstruction by the tongue would redistribute airflow to the other exposure ports. Additionally, there was enough space between the side of the exposure tube and the delivery tube for mice to stick their nose to minimize exposure. One possible improvement would be an insert that fits over the aerosol delivery tube to prevent chewing and avoiding the exposure. Subsequent to this work, the manufacturer indicated that redesigned stainless steel exposure tube adapters that address these concerns were available; however, their effectiveness was not evaluated in this study.
This work was supported by TSE Systems, Inc., Chesterfield, MO 63005, USA.
REFERENCES
- Alessandrini , F. , Semmler-Behnke , M. , Jakob , T. , Schulz , H. , Behrendt , H. and Kreyling , W. 2008 . Total and Regional Deposition of Ultrafine Particles in a Mouse Model of Allergic Inflammation of the Lung . Inhal. Tox , 20 : 585 – 593 .
- Bhalla , D. K. 1997 . “ Specialized Sampling Techniques ” . In Methods in Inhalation Toxicology , Edited by: Phalen , R. F. 137 – 138 . Boca Raton, Fl : CRC Press .
- Cheng , Y. S. , Barr , E. B. , Carpenter , R. L. , Benson , J. M. and Hobbs , C. H. 1989 . Improvements of Aerosol Distribution in Whole-Body Inhalation Exposure Chambers . Inhal. Tox , 1 : 153 – 166 .
- Cheng , Y. S. and Moss , O. R. 1995 . “ Inhalation Exposure Systems ” . In Concepts in Inhalation Toxicology , Edited by: McClellan , R. O. and Henderson , R. F. 25 – 61 . Washington, D.C. : Taylor and Francis .
- DeLorme , M. P. and Moss , O. R. 2002 . Pulmonary Function Assessment by Whole-Body Plethysmography in Restrained Versus Unrestratined Mice . J. Pharm. Toxicol. Methods , 47 : 1 – 10 .
- Guyton , A. C. 1947 . Measurement of the Respiratory Volume of Laboratory Animals . Am. J. Physiol , 150 : 70 – 77 .
- Hofstetter , C. , Flondor , M. , Hoegl , S. , Thein , E. , Kemming , G. , Kisch-Wedel , H. , Kreyling , W. and Zwissler , B. 2004 . Aerosol Delivery During Mechanical Ventilation to the Rat . Exper. Lung Res. , 30 : 635 – 651 .
- MacFarland , H. 1983 . Designs and Operational Characteristics of Inhalation Exposure Equipment—A Review . Fundam. Appl. Toxicol , 3 : 603 – 613 .
- Menache , M. G. , Miller , F. J. and Raabe , O. G. 1995 . Particle Inhalability Curves for Humans and Small Laboratory Animals . Ann Occup. Hyg , 39 : 317 – 328 .
- Moss , O. R. , James , R. A. and Asgharian , B. 2006 . Influence of Exhaled Air on Inhalation Exposure Delivered through a Directed-Flow Nose-Only Exposure System . Inhal. Tox , 18 : 45 – 51 .
- MPPD, Multiple Path Particle Dosimetry model v. 1.0 . 2002 . Dutch National Institute of Public Health and the Environment and Centers for Health Research (CIIT) .
- Nadithe , V. , Rahamatalla , M. , Finlay , W. H. , Mercer , J. R. and Samuel , J. 2003 . Evaluation of Nose-Only Aerosol Inhalaton Chamber and Comparison of Experimental Results with Mathematical Simulation of Aerosol Deposition in Mouse Lungs . J. Pharm. Sci , 92 : 1066 – 1076 .
- NCRP . 1997 . “ Report 125 ” . In Deposition, Retention and Dosimetry of Inhaled Radioactive Substances , Bethesda, MD : National Council on Radiological Protection and Measurements .
- Oldham , M. J. and Robinson , R. J. 2007 . Dosimetry Predictions in an Animal Model of Emphysema . Anat. Rec , 290 : 1309 – 1314 .
- Oldham , M. J. , Phalen , R. F. , Robinson , R. J. and Kleinman , M. T. 2004 . Performance of a Portable Whole-Body Mouse Exposure System . Inhal. Tox , 16 : 1 – 6 .
- Oldham , M. J. , Phalen , R. F. , Schum , G. M. and Daniels , D. S. 1994 . Predicted Nasal and Tracheobronchial Particle Deposition Efficiencies for the Mouse . Ann. Occup. Hyg , 38 ( Sup. 1 ) : 135 – 141 .
- O'Shaughnessy , P. T. , Achutan , C. , O'neill , M. E. and Thorme , P. S. 2003 . A Small Whole–Body Exposure Chamber for Laboratory Use . Inhal. Tox , 15 : 251 – 263 .
- Pauluhn , J. 2003 . Overview of Testing Methods Used in Inhalation Toxicity: From Facts to Artifacts . Tox. Letters , 104–141 : 183 – 193 .
- Phalen , R. F. 1997 . “ Inhalation Exposure Methods ” . In Methods in Inhalation Toxicology , Edited by: Phalen , R. F. 137 – 138 . Boca Raton, Fl : CRC Press .
- Phalen , R. F. 2009 . Inhalation Studies: Foundations and Techniques, , second edition , 109 – 134 . New York : Informa Healthcare .
- Raabe , O. G. , Al-Bayati , M. A. , Teague , S. V. and Rasolt , A. 1988 . Regional Deposition of Inhaled Monodisperse Coarse and Fine Aerosol Particles in Small Laboratory Animals . Ann. Occup. Hyg , 32 ( Sup. 1 ) : 53 – 63 .
- Raabe , O. G. 1976 . Aerosol Aerodynamic Size Conventions for Inertial Sampler Calibration . J. Air Pollut. Contr. Assoc , 26 : 856 – 860 .
- Raabe , O. G. 1968 . The Dilution of Monodisperse Suspensions for Aerosolization . Am. Ind. Hyg. Assoc. J. , 29 : 439 – 443 .
- Tillery , M. I. , Wood , G. O. and Ettinger , H. J. 1976 . Generation and Characterization of Aerosols and Vapors for Inhalation Experiments . Environ. Health Perspect , 16 : 25 – 40 .
- Wichers , L. B. , Rowan , W. H. III , Nolan , J. P. , Ledbetter , A. D. , McGee , J. K. , Costa , D. L. and Watkinson , W. P. 2006 . Particle Deposition in Spontaneously Hypertensive Rats Exposed Via Whole-Body Inhalation: Measured and Estimated Dose . Tox. Sci , 93 : 400 – 410 .
- Yeh , H. C. , Snipes , M. B. , Eidson , A. F. , Hobbs , C. H. and Henry , M. C. 1990 . Comparative Evaluation of Nose-Only Versus Whole-Body Inhalation Exposures for Rats—Aerosol Characterisics and Lung Deposition . Inhal. Tox , 2 : 205 – 221 .
- Yeh , H. C. , Newton , G. J. , Barr , E. B. , Carpenter , R. L. and Hobbs , C. H. 1986 . Studies of the Temporal and Spatial Distribution of Aerosols in Multi-Tiered Inhalation Exposure Chambers . Am. Ind. Hyg. Assoc. J , 47 : 540 – 545 .