Abstract
In this study, an efficient parallel-plate single-stage wet electrostatic precipitator (wet ESP) with a width of 75 mm, effective precipitation length of 48 mm and gap of 9.0 mm was designed and tested to control fine and nanosized particles without the need of rapping. The collection plates are made of sand-blasted copper plates coated with TiO2 nanopowder instead of hydrophilic membranes. Three gold wires (diameter: 100 μ m) were used as the discharge electrodes and a pulse jet valve was used to regularly purge the wires. The design of the present wet ESP is aimed at solving the problems of traditional dry ESPs: reduction of the collection efficiency due to particle deposition on the discharge electrodes and collection electrodes, back corona, and particle re-entrainment. The collection efficiency at initially clean and heavy particle loading conditions was tested and compared to a similar dry ESP.
Experimental results showed that when the wet ESP was initially clean, the particle collection efficiency ranged from 96.9–99.7% for particles ranging from 16.8 to 615 nm in electrical mobility diameter at an aerosol flow rate of 5 L/min (residence time of 0.39 s) and an applied voltage of 4.3 kV. After heavy loading with TiO2 nanopowder about 1.2 ± 0.06 g/plate, the collection efficiency of the present wet ESP for corn oil particles was shown to reduce only slightly to 94.7–99.0% for particles from 16.8 to 615 nm in diameter.
INTRODUCTION
Electrostatic precipitators (ESPs), including dry and wet types, have been widely used to remove particles emitted from various industrial processes. When particles are introduced into a single-stage ESP, they are charged by diffusion and field charging mechanisms and are collected by electrostatic forces on the collection electrodes (CitationHinds 1999). In a well-designed dry ESP, the collection efficiency for micron and submicron particles can reach up to 95% as shown in several experimental (CitationHuang and Chen 2002; CitationMarek et al. 2005) and numerical studies (CitationOglesby and Nichols 1978; CitationYoo et al. 1997; CitationGoo and Lee 1997; CitationLu and Huang 1998). However, aerosol penetration was found to increase with increasing operation time due to the deposition of particles on both the discharge wires and collection electrodes. CitationHuang and Chen (2003) constructed a laboratory-scale, single-stage, wire-plate dry ESP to investigate the loading effect of particles on the collection efficiency. Their results showed that the aerosol penetration for fine cement particles of 0–600 nm in diameter increased after 120 min of particle loading due to the reduction of the ion current. The maximum penetration increase of 4% (from 3.5 to 7.5%) occurred at 300 nm. In addition to the decrease in collection efficiency from the accumulated dust cake, back corona also reduced the efficiency by decreasing the particle charging ratio and migration velocity (CitationChang and Bai 1999). Furthermore, dry ESPs can not be used to control sticky or oily particles, which are difficult to remove from the collection electrodes by rapping (CitationAltman et al. 2001).
Wet ESPs have been developed to control a wider variety of particulate pollutants and exhaust gas conditions compared to dry ESPs, especially for particles that are sticky, corrosive, or have high resistivity (CitationAltman et al. 2001a, Citation2001b). The periodic or continuous scrubbing water flow, used to wash the collection electrode surfaces, was found to prevent particle re-entrainment caused by rapping, which occurs in dry ESPs (CitationUSEPA 2003). CitationLungren et al. (1995) invented a wire-in-tube wet ESP in which the charging electrode consisted of a tapered section, a rod and four discs to enhance particles charging efficiency. Scrubbing water was first introduced onto a diamond-shaped plate, which was used to equalize the water flow, and then flowed into the inner surface of the collection electrode to remove the collected particles. They found that the opacity of the flue gas was reduced from 35 to 0% in the pilot unit when the applied voltage was increased from 30 to 75 kV. However, the collection efficiency for different particle diameters and particle loadings was not discussed. Similar types of wire-in-tube wet ESPs were used to control the emissions of incinerator flue gas (CitationKim et al. 2000) and diesel particulate matter (CitationSaiyasitpanich et al. 2006, Citation2007). The collection efficiency of particle mass of the wet ESP for 10–1000 nm diesel particles was measured to be from 92 to 69% when the gas velocity was from 1.38 to and 5.61 m/s (or the corresponding residence time from 0.4 to 0.1 s) (CitationSaiyasitpanich et al. 2007), respectively.
However, the collection efficiency under heavy particle loading conditions for long time periods was not studied. Moreover, the previous study used a high flow rate of scrubbing water (4.44–6.22 L/min/m2 of collection area), which resulted in a thick water film, thus decreasing the electric field strength due to water's resistivity.
To improve the uniformity of the liquid film over the collection electrodes without having to supply the scrubbing water at high flow rate, CitationPasic et al. (2001) invented a membrane electrostatic precipitator which used hydrophilic membranes made of corrosion resistant woven fibers as the collection electrodes. In the bench scale test, CitationBayless et al. (2004) compared the membrane-based and steel plate wet ESPs for the collection efficiency of fly ash, iron oxide and calcium carbonate having particle sizes of 20–25, 1–5, and 2–3 μ m in diameter, respectively. The collection efficiency of the membrane-based wet ESP was found to be higher than the steel plate wet ESP. In the pilot scale test, the membrane wet ESP was used to remove iron-oxide particles with the peak gas velocity of 4 m/s or the corresponding residence time of 1.9 s. The collection efficiency of particle mass was approximately 96% for particles from 1 to 5 μ m in diameter. However, the collection efficiency of submicron particles under heavy particle loading conditions was not discussed. In addition, membranes may be expensive and not all have uniform wetting and corrosion-resistant properties, which must be carefully determined for optimal use in specific field applications (CitationBayless et al. 2004).
The objective of this study was to develop a wire-in-plate single-stage wet ESP to enhance the collection efficiency of fine and nanosized particles. Instead of hydrophilic membranes, sand blasted copper plates coated with TiO2 nanopowder were used as the collection plates. The design of the present wet ESP was aimed at solving the problems of the traditional dry ESPs: reduction of the collection efficiency due to particle deposition on the discharge electrodes and collection electrodes, back corona, and particle re-entrainment. The collection efficiency at initially clean and heavy particle loading conditions was tested and compared with that of the dry ESP.
EXPERIMENTAL MATERIALS AND METHODS
The Present Parallel-Plate Wet ESP
The collection electrodes of the present parallel plate wet ESP were designed based the parallel-plate wet denuder in CitationTsai et al. (2008). As shown in , the wet ESP consists of two plexiglass plates (M) on which a copper plate (G) (100 mm in length, 75 mm in width, and 3.0 mm in thickness) was attached to the inner surface. A copper plate was used as collection electrode because of its high conductivity and ease with sandblasting. In order to make scrubbing water film flow uniformly on the collection electrodes (G) at low flow rates, a frosted glass plate (FG, 70 mm in length, 75 mm in width, and 3.0 mm in thickness) coated with TiO2 nanopowder (Degussa AEROXITE TiO2 P25, Anatase, 20 nm) is attached to the inner surface (M) above the copper plate. Between the collection electrodes, a center piece (C) is sandwiched to form a 9 mm gap between the electrodes. On the center piece, three gold discharge wires (GW) (99% purity, 100 μ m in diameter, Surepure Chemetals Inc.) spaced at 16 mm in the flow direction are fixed. These gold wires were used as discharge wires due to their long lifetime of more than 6 months (CitationAsbach 2004). Two overflowing (OR) and collection reservoirs (CR) for continuous scrubbing water flow are installed at the top and bottom of the ESP, respectively. A pulse jet valve (Bag Filter Enterprise Co. Ltd., Taiwan) was used to generate pulse jet passing through 3 rows of small holes (diameter: 1.0 mm, 24 holes on each row) on the collection plates. The discharge wires were cleaned every 5 min with an instantaneous pressure of 2.95 atm (3.05 kg/cm2) and the instantaneous air flow rate passing through all 24 holes per wire was calculated to be 11 L/s. Pulse duration was about 0.5 s. During pulse jet cleaning, the scrubbing water flow was stopped for two minutes to prevent sparking over due to the water mist generated by the pulse jet.
FIG. 1 Schematic diagram of the parallel-plate wet ESP. Plexiglass plates (M), enter piece (C), frosted glass plate (FG), sand-blasted copper plate (G), overflowing reservoir (OR), collecting reservoir (CR), golden wire (GW), liquid inlet (LI), liquid outlet (LO), aerosol inlet (AI), aerosol outlet (AO), pulse jet valve (PJ), air hole (AH).
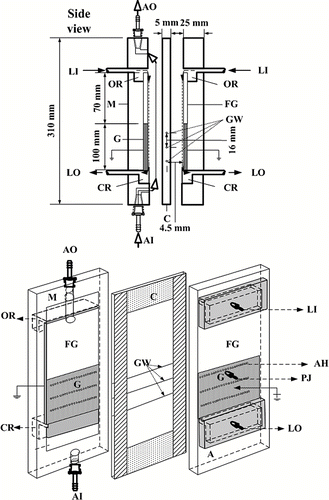
In order to increase the hydrophilicity of the collection plates, which will enhance the uniformity of the water film and reduce the scrubbing water flow rate, the copper plate surfaces were first sand blasted to an average depth of 61.0 μ m and then coated with TiO2 nanopowder (Degussa P25) based on the method described in CitationTsai et al. (2008). The surfaces were first sonicated with DI water (18.2 MΩ cm) and then dried by purging with compressed air. 0.5 g of TiO2 nanopowder was well dispersed in 50 ml DI water by ultrasonication and then applied onto the sand blasted surfaces. 30 min later, the excess solution was removed, and the plates were calcinated at 300°C for 90 min to facilitate thermal bonding of the TiO2 nanopowder onto the copper plate surfaces.
Tap water was used as the scrubbing liquid for the collection electrodes. Two peristaltic pumps (Model MP-1000, Eyela Co., Japan) were used to continuously pump water into and out of the overflow and collection reservoirs. The appropriate scrubbing water flow rate, important to water film thickness and uniformity (CitationTsai et al. 2008), was determined in this study. The goal was to minimize the flow rate in order to decrease the water waste and film thickness (which decreases the electric field due to water's resistivity), while also ensuring the film is uniform.
To determine the hydrophilicity of the coated surfaces, the water contact angle was measured by using a Contact Angle System (FTA125, First Ten Angstroms, VA). The contact angle was found to be only 6.0 ± 4.2°, which is very small compared to 104.03 ± 1.73° and 117.17 ± 2.83° for the smooth copper plates and uncoated sand-blasted copper plates, respectively.
Particle collection efficiency experiments were conducted at different aerosol flow rates and applied voltages under initially clean conditions to determine the optimum operation conditions. TiO2 nanopowder was then used to create heavy loading conditions for comparing the collection efficiency of the dry and present wet ESP.
Particle Collection Efficiency Experiment and Loading Test
The particle collection efficiency experiments under initially clean conditions were conducted at aerosol flow rates of 5 and 10 L/min (corresponding to residence times of 0.39 and 0.19 s, respectively, over the total precipitation length of 48 mm, which is assumed to be three times the wire spacing) with applied voltages ranging from 3.8 to 4.3 kV. The experiment under each operation condition was tested for 6 to 8 h. The experimental setup is shown in . Polydisperse liquid corn oil particles (density of 0.9 g/cm3) were generated by the evaporation-condensation technique (CitationScheibel et al. 1982) at an oven temperature of 260°C. Two rotameters were used to control carrier gas and cooling air flow rates to obtain the desired particle diameter and concentration. Before being introduced into the present wet ESP, all charged particles were removed using a wire-in-tube ESP allowing only zero-charged particles to enter the wet ESP. To generate an electric field and corona ions, high positive voltage was supplied to the corona wires using a high voltage D.C. power supplier (Model SL150, Spellman High Voltage Electronics Corporation, NY, USA). The voltage and corresponding corona current were read directly from the power supplier. A scanning mobility particle sizer (SMPS, model 3081, TSI Inc.) coupled with a condensation particle counter (CPC, model 3022, TSI Inc.) was used to measure the size distribution of the polydisperse particles. The characteristics and number size distribution of the test aerosols are shown in , respectively. The particle collection efficiency of the wet ESP, ηtotal(dp), was calculated by the following equation:
TABLE 1 The characteristics of the test particles
To study particle loading effect on the collection efficiency and possible particle reentrainment after particle loading, Degussa P25 TiO2 nanopowder (resistivity of 0.75 × 106Ω · cm) (CitationSalah et al. 2004) was dispersed by a Jet-O-Mizer (Model 000, Fluid Energy Processing and Equipment Co., Hatfield, UK) at an aerosol flow rate of 5.67 ∼ 56.62 L/min corresponding to a mass loading rate of 0.1–100 g/hr as shown in . The particles that penetrated through the ESP (Wout, g) and the particles in the excess flow (Wex, g) were measured by weighing the filters at the outlet of the wet ESP and the excess flow. After loading for two hours, the quantity of particles in the ESP per plate, Wloaded (g), was then calculated to be 1.2 ± 0.06 g/plate by the following equation:
To examine possible redispersion of particles into gas stream after heavy TiO2 nanoparticle loading in the dry ESP, a particle reentrainment test was conducted. After two hours of loading, clean air at a flow rate of 5 L/min was introduced into the dry ESP when the applied voltage was 4.3 kV. The scanning mobility particle sizer (SMPS, model 3081, TSI Inc.) was used to measure the reentrained particle size distributions at 135 s sampling interval.
RESULTS AND DISCUSSION
Flow Rate, Uniformity, and Thickness of the Water Film
Surface texture and liquid flow rate are two important factors that affect the uniformity of the water film (CitationTsai et al. 2008). As mentioned previously, the contact angle was greater than 100° for the smooth collection electrodes or uncoated sand-blasted electrodes. It was found that water film on these electrodes was not uniform. In comparison, for the TiO2 coated, sand-blasted electrodes, scrubbing water was found to be uniform over the collection surfaces at the liquid flow rate per collection surface area (0.075 × 0.17 m2) of 2.31 L/min/m2. Similar findings were reported by CitationTsai et al. (2008), CitationYu et al. (2001), and CitationLiu et al. (2002). It was also found that channeling occurred when the liquid flow rate per collection surface area was lower than 2.31 L/min/m2. These findings demonstrate that both surface texture and liquid flow rate are important for creating a uniform water film. Comparing with the liquid flow rate of 4.44–6.22 L/min/m2 used by Saiyasitpanich et al. (Citation2006; Citation2007), the present design saved 48–63% of the scrubbing water. The thickness (w) of the water film was calculated to be 0.1 ± 0.18 mm by the method presented in CitationTsai et al. (2008). With this small thickness, the water film was still found to decrease the corona current compared to a dry ESP with the same design except without scrubbing water or purge air flows. shows the comparison of the corona current between the dry and wet ESPs, at various supplied voltages. It is seen that at the same voltage, the corona current was decreased after supplying the scrubbing water on the collection plate surfaces, due to the resistivity of the water film. Hence, the wet ESP collection efficiency is expected to be lower than the dry ESP operating at the same voltage. Therefore, for the initially clean condition the operation voltage has to be increased for the present wet ESP to have the same collection efficiency as the dry ESP. As will be shown later, the advantage of using scrubbing water flow and purge air flow becomes obvious when the collection surfaces are loaded heavily with TiO2 nanopowder.
Particle Collection Efficiency of the Wet ESP at Different Aerosol Flow Rates and Applied Voltages
shows the collection efficiency of the wet ESP as a function of corn oil diameter when the aerosol flow rate is 5 and 10 L/min and the applied voltage is 4.3 kV under the initially clean condition. The residence time of the aerosol in the present wet ESP was calculated to be 0.39 and 0.19 s for aerosol flow rates of 5 and 10 L/min, respectively. As seen in , decreasing the flow rate from 10 to 5 L/min (or increasing the residence time from 0.19 to 0.39 s) had a substantial effect on the collection efficiency as the Deutsch-Anderson equation dictates. At the flow rate of 5 L/min, the collection efficiency was 96.9–99.7% for particles from 16.8 to 615 nm in electrical mobility diameter. The minimum collection of 96.9% corresponds to the particle diameter of 126.3 nm. As the flow rate is increased to 10 L/min, the minimum collection efficiency is reduced to 84.1% at the corresponding diameter of 70 nm.
FIG. 4 Collection efficiency of the present wet ESP for corn oil particles at the aerosol flow rate 5 and 10 L/min and the applied voltage of 4.3 kV. Each test was repeated 6 times.
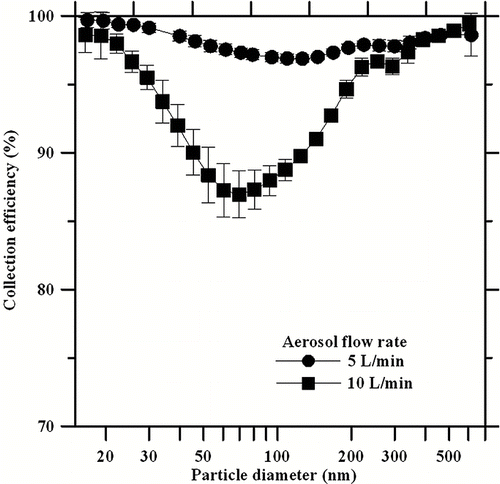
According to particle charging theory, particle charge decreases with decreasing particle diameter, while the mechanical mobility increases rapidly with decreasing particle diameter (CitationHinds 1999). Therefore, the collection efficiency of ESPs for particles in the size range of 0.1 to 1 μ m has a U-shape efficiency curve, as shown in . CitationSaiyasitpanich et al. (2006) also found a U-shaped collection efficiency curve for the wet ESP with a minimum of about 95% for particles between 80 and 250 nm in diameter when the supplied voltage was 70 kV, gas residence time was 0.4 s, and the particle concentration was 15.9 mg/Nm3.
shows the collection efficiency of the present wet ESP for corn oil particles in the size range of 16.8 to 615 nm under different applied voltages at the aerosol flow rate of 5 L/min. As shown in the figure, the corn oil particle collection efficiency also shows a U-shape efficiency curve for particles from 16.8 to 615 nm in electrical mobility diameter and the efficiency decreases with decreasing applied voltage because of the reduction of the electric field strength. When the applied voltage was decreased from 4.3 to 4.1 kV, the collection efficiency decreased slightly from 96.9–99.7% to 93.9–99.4%. The collection efficiency was further decreased to 74.2–82.4% and 11.4–35.5% for the same particle diameter range when the applied voltage was reduced to 3.9 and 3.8 kV, respectively. Based on these experimental results, it is certain that the present wet ESP can be operated to efficiently control fine and nanosized particles at an aerosol flow rate of 5 L/min, applied voltage of 4.3 kV, and scrubbing water flow rate per collection surface area of 2.31 L/min/m2.
FIG. 5 Collection efficiency of the present wet ESP for corn oil particles under different applied voltages. The aerosol flow rate is fixed at 5 L/min. Each test was repeated 6 times.
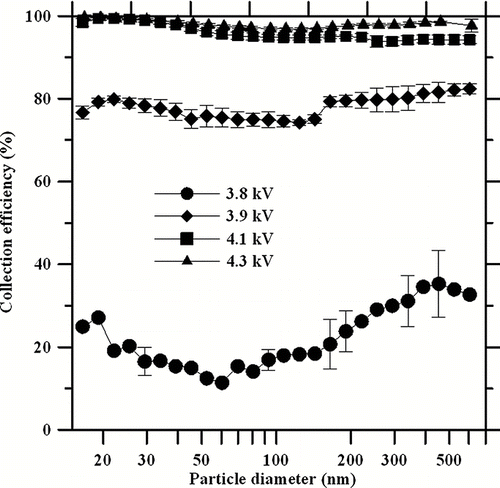
In the present wet ESP, high particle collection efficiency (99%) was found for small nanoparticles (16.8 to 29.4 nm) with an applied voltage of 4.3 kV. This is attributed to the high electrostatic precipitation efficiency ηelec(dp) and diffusive deposition ηdiff(dp) in this size range, which can be calculated by the following equations:
Particle Loading Test
The particle collection efficiency for corn oil particles was tested by the dry and wet ESP at an aerosol flow rate of 5 L/min and an applied voltage of 4.3 kV for a long operation time of 10 hours. The collection efficiency in the dry ESP was found to decrease only slightly from 99.2–100% to 98.7–100% for 10.4–149 nm particles after the 10-hour test (loaded corn oil: 5.04 × 10–5 mg/plate). Particle accumulation on the collected plates and wires was found to be negligible due to the small loaded particle mass. Similar tests for the wet ESP also showed that the collection efficiency was as high as the initial condition (99.1–98.4% for 10.4–149 nm particles) after 10 hours of loading corn oil particles. Therefore, heavy loading conditions on the collection plates had to be generated by dispersing powders, such as TiO2 nanopowder used in this study.
The collection efficiency was tested for the dry and wet ESP with an aerosol flow rate of 5 L/min, an applied voltage of 4.3 kV, and with a TiO2 loading quantity of 0.6 ± 0.06 g/hr/plate (4 ± 0.06 g/m3). As shown in , the collection efficiency decreased slightly from 96.9–99.7% to 92.1–99.3% for electrical mobility diameters of 16.8–615 nm in the dry ESP after 30 min of particle loading. However, after one hour of loading, the aerosol penetration increased sharply from 0.9–1.6% to 12.4–55.4% for the same range of electrical mobility diameters. The particle collection efficiency finally decreased below 35.0% for all particles (). This resulted from the reduction of the electric field strength due to the accumulation of TiO2 particles on the collected plates and the discharge wires. As shown in , it is evident that the corona current, and hence the electric field strength, decreases dramatically under the same applied voltage after two hours of heavy particle loading because of the additional resistivity of the dust layer.
FIG. 7 Collection efficiency for corn oil particles in the dry ESP at different TiO2 nanopowder loadings. The applied voltage and aerosol flow rate are 4.3 kV and 5 L/min, respectively.

Experimental results showed that after two hours of TiO2 nanoparticle loading, the total number concentration of reentrained particles was 612.2 #/cm3 (16.8 to 615 nm) at the first sampling interval of 135 s and then reduced to almost zero after that. Many particles were seen to be dislodged and redispersed back into the gas stream because the electrostatic voltage difference across the TiO2 dust layer was very low. These reentrained particles were too small to settle by gravity and would leave the dry ESPs (CitationRichards 1995).
Under the same loading conditions as the dry ESP, the collection efficiency of the present wet ESP for corn oil particles was measured to be as high as 97.6–99.5% and 94.9–99.9% for particles from 16.8 to 615 nm in electrical mobility diameter after 30 and 120 min particle loadings, respectively, as shown in . The particle loaded mass used in this study, 4 ± 0.06 g/m3, is much higher than 2.8–15.9 mg/Nm3 used in Saiyasitpanich et al. (Citation2006; Citation2007). By direct observation, the continuous scrubbing water was seen to wash the collection plates clean and maintain a uniform water film. The pulse air jet was also capable of cleaning the discharge wires regularly. These were key factors in maintaining a high collection efficiency of the present wet ESP under heavy particle loading conditions.
CONCLUSIONS
This study designed and developed a parallel-plate single-stage wet ESP to control fine and nanosized particles without the need of rapping. Sand-blasted copper plates coated with TiO2 nanopowders were used as collection plates to enhance hydrophilicity for the scrubbing water film. A pulse jet valve was used to clean the corona wires regularly. Corn oil particles were used to conduct particle collection efficiency experiments with different applied voltages and aerosol flow rates under an initially clean condition. TiO2 nanopowder was used to create heavy loading conditions to compare the particle collection efficiency of the dry and present wet ESP.
The experimental results showed that the present wet ESP can be operated to efficiently control fine and nanosized particles at an aerosol flow rate of 5 L/min, applied voltage of 4.3 kV and scrubbing water flow rate per collection surface area of 2.31 L/min/m2 under the initially clean condition.
Under heavy loading conditions with a TiO2 loading quantity of 0.6 ± 0.06 g/hr/plate, the dry ESP particle collection efficiency decreased below 35% for corn oil particles in the electrical mobility diameter range of 16.8–615 nm after two hours of loading. Under the same loading conditions, the collection efficiency for corn oil particles in the same size range was measured to be as high as 94.9–99.9% for the wet ESP. This is because the uniform scrubbing water film and the pulse jet can effectively clean the collection plates and the corona wires, respectively, to keep the collection efficiency high.
For practical applications, the present lab-scale wet ESP could be scaled up to a size that allows treatment at high aerosol flow rates. When particles deposit on the collection electrode in the wet ESP, they will be removed by scrubbing water and will not accumulate on the collection electrode. Therefore, it is expected that the present wet ESP can be used to control fine and nanosized particulates emitted from nanomaterial manufacturing processes without the typical problems associated with dry ESPs, such as particle reentrainment due to rapping and back corona due to the formation of the dust cake with high specific resistivity. In addition, since the particle collection efficiency of the present wet ESP is very high, it can be used as airborne particle and biological aerosol samplers. The collected liquid samples can be analyzed further for chemical as well as biological compositions.
Acknowledgments
The support of the Taiwan National Science Council (Contract no. NSC96-2628-E-009-008-MY3 and NSC96-2120-M-006-005) is greatly acknowledged.
Notes
*Generated by a small-scale powder disperser (SSPD, model 3433, TSI Inc.).
REFERENCES
- Altman , R. , Offen , G. , Buckley , W. and Ray , I. 2001a . Wet Electrostatic Precipitation: Demonstrating Promise for Fine Particulate Control—Part I . Power Eng. , 105 : 37 – 39 .
- Altman , R. , Buckley , W. and Ray , I. 2001b . Wet Electrostatic Precipitation: Demonstrating Promise for Fine Particulate Control—Part II . Power Eng. , 105 : 42 – 44 .
- Asbach , C. 2004 . Development and evaluation of a highly efficient gas particle partitioner with minimal effect on the gas composition , Ph. D. thesis University Duisburg-Essen .
- Bayless , D. J. , Alam , M. K. , Radcliff , R. and Caine , J. 2004 . Membrane-Based Wet Electrostatic Precipitation . Fuel Process. Technol. , 85 : 781 – 798 .
- Chang , C. L. and Bai , H. 1999 . An Experimental Study on the Performance of a Single Discharge Wire-Plate Electrostatic Precipitator with Back Corona . J. Aerosol Sci. , 30 : 325 – 340 .
- Goo , J. G. and Lee , J. W. 1997 . Stochastic Simulation of Particle Charging and Collection Characteristics for a Wire-Plate Electrostatic Precipitator of Short Length . J. Aerosol Sci. , 28 : 875 – 893 .
- Hinds , W. C. 1999 . Aerosol Technology , 245 – 257 . New York : John Wiley & Sons .
- Huang , S. H. and Chen , C. C. 2002 . Ultrafine Aerosol Penetration through Electrostatic Precipitators . Environ. Sci. Technol. , 36 : 4625 – 4632 .
- Huang , S. H. and Chen , C. C. 2003 . Loading Characteristics of a Miniature Wire-Plate Electrostatic Precipitator . Aerosol Sci. Technol. , 37 : 109 – 121 .
- Kim , H. H. , Yamamoto , I. , Takashima , K. , Katsura , S. and Mizuno , A. 2000 . Incinerator Flue Gas Cleaning Using Wet-Type Electrostatic Precipitator . J. Chem. Eng. JPN. , 33 : 669 – 674 .
- Liu , Q. , Wu , X. , Wang , B. and Liu , Q. 2002 . Preparation and Super-Hydrophilic Properties of TiO2/SnO2 Composite Thin Films . Mater. Res. Bull. , 37 : 2255 – 2262 .
- Lu , C. and Huang , H. 1998 . A Sectional Model to Predict Performance of a Plate-Wire Electrostatic Precipitator for Collecting Polydisperse Particles . J. Aerosol Sci , 29 : 295 – 308 .
- Lundgren , D. A. , Marple , V. A. and Herrick , R. A. Electrostatic Precipitator Assembly . U.S. Patent 5, 395, 430 . 1995 .
- Marek , K. , Jaroslaw , D. and Jerzy , M. 2005 . Particle Precipitation Efficiency in an Electrostatic Precipitator . J. Electrost. , 63 : 761 – 766 .
- Oglesby , S. J. and Nichols , G. B. 1978 . Electrostatic Precipitation: Computer Model , 157 – 210 . New York : Marcel Dekker, Inc. .
- Pasic , H. , Alam , M. K. and Bayless , D. J. Membrane Electrostatic Precipitator . U.S. Patent, 6, 231, 643, B1 . 2001 .
- Richards , J. 1995 . Control of Particulate Emissions , APTI Course 413 6-20 – 6-23 . Washington, DC : EPA .
- Saiyasitpanich , P. , Keener , T. C. , Lu , M. , Khang , S. J. and Evans , D. E. 2006 . Collection of Ultrafine Diesel Particulate Matter (DPM) in Cylindrical Single-Stage Wet Electrostatic Precipitators . Environ. Sci. Technol. , 40 : 7890 – 7895 .
- Saiyasitpanich , P. , Keener , T. C. , Khang , S. J. and Lu , M. 2007 . Removal of Diesel Particulate Matter (DPM) in a Tubular Wet Electrostatic Precipitator . J. Electrostat. , 65 : 618 – 624 .
- Salah , N. H. , Bouhelassa , M. , Bekkoushe , S. and Boultif , A. 2004 . Study of Photocatalytic Degradation of Phenol . Desalination. , 166 : 347 – 354 .
- Scheibel , H. G. and Porstendörfer , J. 1982 . Generation of Monodisperse Ag- and NaCl Aerosols With Particle Diameters between 2 and 300 nm . J. Aerosol Sci. , 14 : 113 – 126 .
- Tsai , C. J. , Lin , G. Y. and Chen , S. C. 2008 . A Parallel Plate Wet Denuder for Acidic Gas Measurement . AIChE J , 54 : 2198 – 2205 .
- Tsai , C. J. , Wu , C. H. , Leu , M. L. , Chen , S. C. , Huang , C. Y. , Tsai , P. J. and Ko , F. H. 2009 . Dustiness Test of Nanopowders using a Standard Rotating Drum with a Modified Sampling Train . J. Nanopart. Res. , 11 : 121 – 131 .
- U.S. Environmental Protection Agency . 2003 . Air Pollution Control Technology Fact Sheet , Washington, DC : EPA . EPA-452/F-03-030
- Yoo , K. H. , Lee , J. S. and Oh , M. D. 1997 . Charging and Collection of Submicron Particles in Two-Stage Parallel-Plate Electrostatic Precipitators . Aerosol. Sci. Technol. , 27 : 308 – 323 .
- Yu , J. , Zhao , X. , Zhao , Q. and Wang , G. 2001 . Preparation and Characterization of Super-Hydrophilic Porous TiO2 Coating Films . Mater. Chem. Phys. , 68 : 253 – 259 .