Abstract
Aerosolized coliphage phiX174 and lactococcal phage P008 were sampled with two types of filter, polycarbonate (PC) and polytetrafluoroethylene (PTFE). The recovery was determined by plaque assays and quantitative polymerase chain reaction (qPCR). Recovery by qPCR was greater than by culture and PC filters outperformed PTFE filters both by culture and by qPCR relative recovery. The results of the plaque assays showed that the infectivity of the recovered phages was affected by the aerosolization/air sampling. The presence of viruses in air samples should be determined by culture-independent assays.
Viruses are now recognized as the most abundant biological entities on the planet. They can be found on aerosol particles of various sizes, ranging from the nanometer size of the actual virus to tens of micrometers in aerodynamic diameter. The viral aerosol size will depend on a variety of factors, including the aerosol source as well as its nature, its concentration, and its distribution in the environment (CitationWichmann et al. 2000). Several samplers, such as liquid impingers (CitationVerreault et al. 2008), slit samplers (CitationThomas 1970; CitationDahlgren et al. 1961), and filters (CitationHarstad 1965; CitationHapp et al. 1966; CitationTseng and Li 2005) are available to sample viral aerosols. The efficacy of these samplers for airborne viruses most often depends on the recovery of infectious viral particles and, therefore, mainly on the preservation of viral integrity. It is currently impossible to determine, in a cloud of airborne viruses, the proportion of the aerosol that is really infectious, given that aerosolization, desiccation, sampling procedures, and environmental factors can significantly affect the structural integrity of the viruses (CitationVerreault et al. 2008). Hence, using a sampler to determine the concentration of infectious airborne viruses is invariably biased by the sampling procedure and the environmental conditions (temperature, humidity, etc.). An analytical procedure based on methods that are independent of viral infectivity, such as quantitative polymerase chain reaction (qPCR), is likely better suited for the analysis of air samples. The choice of a sampler for the recovery of airborne viruses should thus be based on the capacity of the sampler to harvest the targeted particle size.
Appropriate filters can efficiently capture nanometer-sized airborne virus-laden particles (CitationBurton et al. 2007) as well as larger particles, and can therefore intercept a much more representative cohort of airborne particles. In this study, 0.4 μ m polycarbonate (PC) capillary pore filters (SKC Inc., Eighty Four, PA) and 0.3 μ m polytetrafluoroethylene (PTFE) membrane filters (SKC Inc.) were compared for their efficiency in recovering airborne viruses. The filters, all 37 mm in diameter, were mounted on cellulose pads (SKC Inc.) of the same diameter and placed inside a separate 37 mm clear styrene three-piece cassette (SureSeal, SKC Inc.). Filter pumps (GilAir-5, Gilian, Sensidyne, LP, Clearwater, FL) were individually calibrated for PC or PTFE filters at a rate of 2 L/min. The efficiency of the samplers was assessed by phage plaque assays as well as qPCR. Two bacterial viruses, phiX174 and P008, were used as models. PhiX174 is a non-enveloped icosahedral coliphage of 27 nm in size and has a single-stranded circular DNA (ssDNA) genome containing 5,386 nucleotides (CitationSanger et al. 1978). P008 is a virulent Lactococcus lactis phage with an isomeric capsid of 53 nm in diameter and a non-contractile tail of 159 nm in length and 11 nm in diameter (CitationJarvis et al. 1991). It has a double-stranded DNA (dsDNA) genome of 28,538 base pairs (bp). Both phages and their host strains (E. coli C and L. lactis F7/2A) were obtained from the Felix d'Hérelle Reference Center for Bacterial Viruses (www.phage.ulaval.ca). Plaque assays were performed as reported elsewhere (CitationJarvis 1978) using GM17 medium supplemented with CaCl2 (10 mM) for L. lactis (30°C) and TSB medium for E. coli (37°C). Phage phiX174 has been used repeatedly as a surrogate for human and animal viruses (CitationVerreault et al. 2008), while phage P008 is responsible for milk fermentation failures (CitationJarvis et al. 1991).
Phage-containing aerosols were generated with an atomizer (model 9302, TSI Inc., Shoreview, MN, USA) at a dispersion rate of 3.0 L/min. The aerosols were then passed through a desiccator (model 306200, TSI Inc.) before entering the 3.36 liter GenaMini chamber (SCL Medtech Inc., Montreal, QC, Canada) from which the aerosols were sampled (). Aerosols inside the chamber were mixed with the dilution air at a rate of 20 L/min and were maintained at a continuous flow throughout the sampling procedure, the residence time of the aerosol inside the chamber was thus estimated at 8.8 s. Of the nine sampling ports available on the chamber, six were used for aerosol sampling and one was used for monitoring aerosol size distribution and concentration with an aerodynamic particle sizer (APS, model 3321, TSI Inc.) (). HEPA filtered and dried, medical-grade compressed air was used for aerosol generation and dilution. Inside the chamber, relative humidity was < 20% and temperature was between 29°C and 31°C, as measured with a hygrometer/thermometer probe (model 4154, Control Company, Friendswood, TX, USA).
FIG. 1 Chamber set-up. Connections are made with 6.35 mm diameter Stainless Steel (SS) tubes with 4.5 mm interior diameter and flexible 8 mm diameter polyurethane tubes with 5 mm interior diameter. (A) atomizer (between A and B: 73.5 cm SS and 26.5 cm polyurethane tubes), (B) desiccator (between B and C: 59 cm SS and 20 cm polyurethane tubes), (C) aerosol chamber (image provided by SCL Medtech) (between C and D: 93 cm polyurethane tube), (D) aerodynamic particle sizer, (E) sampling ports, (F) dilution and generation air flow rate controls, (G) outlet for contaminated air to exit to HEPA filter, (H) inlet for medical-grade compressed air to enter set-up, (I) outlet for clean air to exit to atomizer, (J) inlet for dry aerosol to enter chamber. The set-up was placed in a biosafety level 2 cabinet.
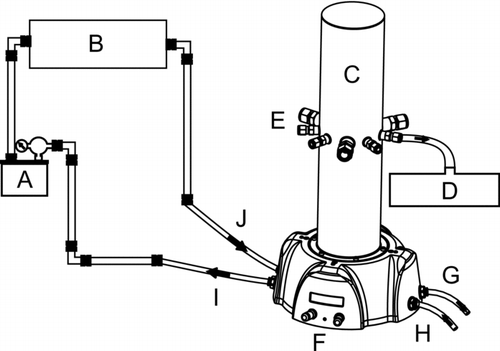
FIG. 2 Simplified upper view of the aerosol chamber showing the positions of the APS and the filters on the sampling ports (numbered 1 to 9). The PTFE and PC filters were switched from the grey to the white positions from one experiment to another; a single color represents a single type of filter. Ports 3 and 9 were not used in this study, so the valves are shown on the diagram as closed.
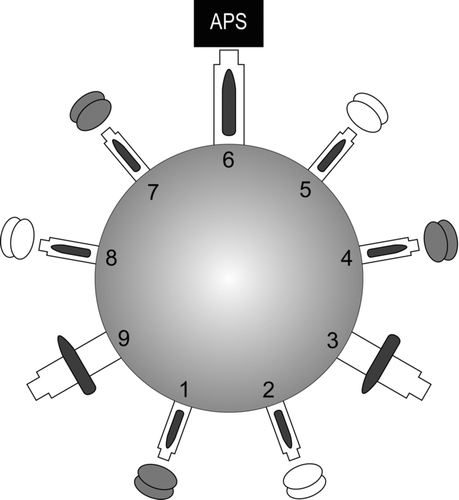
The preparation of the chamber's nebulization medium necessitated the preparation of two phage suspensions. Solution 1 was composed of 49 ml phage buffer (10 mM Tris-HCl, 100 mM NaCl, 10 mM MgSO4, adjusted to pH 7.4) and of 1 ml fresh lysate of phage phiX174. Solution 2 was composed of 49 ml phage buffer and of 1 ml fresh lysate of phage P008. The nebulization medium, called Solution 3, was composed of a 50/50 mixture of Solutions 1 and 2. Then, 70 ml of Solution 3 was used in the atomizer. The mass median diameter (MMD) of the aerosols ranged between 1.3 and 1.7 μ m, and the total concentration ranged between 3.6 × 103 and 6.3 x 103 particles/cm3. Aerosols were sampled for 20 min using filters positioned as illustrated in . Each sampling setup consisted of three PC filters and three PTFE filters alternatively placed either at positions 1, 4, and 7 or at positions 2, 5, and 8. Phages were eluted from the filters (still mounted in the cassettes with the support pad) with 5 ml sterile MiliQ water supplemented with 0.01% Tween 20. The latter was shaken on a vortex for 20 min to maximize recovery. We had previously determined that this shaking step had no effect on the infectivity of both phages (data not shown). The liquid was then transferred to sterile tubes and kept at 4°C until analysis by plaque assays and qPCR which were done within 24 h after sampling. The above sampling experiment was repeated six times. Culture and qPCR analysis were performed in duplicate on the nebulization mediums.
Real-time PCR experiments were performed on a DNA Engine Opticon 2 apparatus (Bio-Rad Laboratories, Hercules, CA). Primers and probes were designed using the Beacon Designer 4.0 program (PREMIER Biosoft International, Palo Alto, CA). For phage phiX174, a dual-labeled probe was first designed (5′ -/56-FAM/CTCTCGTGCTCGTCGCTGCGTTGA/3BHQ-1/-3′; corresponding to nucleotide positions 533 to 556 in the phiX174 genome (CitationSanger et al. 1978)) followed by a reverse primer (5′ -CGGCAGCAATAAACTCAACAGG-3′; positions 609 to 630) and a forward primer (5′ -ACAAAGTTTGGATTGCTACTGACC-3′; positions 508 to 531). The targeted region was also cloned into TOPO (Invitrogen, Carlsbad, CA), purified (Qiagen Inc., Mississauga, ON, Canada), and used as a standard in the qPCR protocol. A master mix of the qPCR chemicals was prepared and then distributed in aliquots of 20 μ l per well in a 96 well plate. Each 20 μ l aliquot of the master mix contained a final concentration of 1X buffer (IQ Supermix, Bio-Rad Laboratories), 0.5 μ M forward primer, 0.5 μ M reverse primer, and 0.2 μ M probe. To each well, 5 μ l phage-containing samples were added for a qPCR reaction volume of 25 μ l. A standard curve was done using 5 × 102 to 5 × 106 plasmid copies per reaction tube. Each reaction was done in duplicate. The qPCR protocol was optimized (data not shown), and the best conditions were 94°C for 3 min (hot start) followed by 40 cycles of 94°C for 15 s and 60°C for 1 min. The cycle threshold or C(t) was used to calculate the concentration of the original sample. The C(t) corresponded to the PCR cycle at which the intensity of the fluorescence emitted by the sample exceeded the threshold of intensity set to identify positive samples.
For the detection and quantification of phage P008 (CitationMahony et al. 2006), a SYBR Green protocol was designed and optimized (data not shown) using a reverse primer (5′ -CCAGCAGTAGGGCGAACAAAG-3′; positions 5167 to 5187) and a forward primer (5′ -TGAGGGAGACGGAACAAACGG-3′; positions 5035 to 5056). A control plasmid was prepared as for phiX174. Quantitative PCR analysis was performed on 96 well plates as described for phiX174. The qPCR mixture was prepared using IQ SYBR Green Supermix (Bio-Rad Laboratories), the run conditions were 94°C for 3 min (hot start) followed by 35 cycles of 94°C for 20 s, 60°C for 30 s, and 72°C for 25 s. The melting curve was from 50°C to 95°C, with reading every 0.2°C and holding for 1 s.
Preliminary assays were performed to identify potential inhibitors of the plaque assays and qPCR reactions. The liquid used to recover phages from the filters was first spiked with phages, and we found no inhibitors of the plaque assays or qPCR. Then, the filters (PC and PTFE) were eluted using phage-containing liquid to verify the effect of the elution process on phages. No difference was observed as a result of either qPCR or the plaque assays for either type of filter. Selective elution of free phage DNA was also ruled out by treating the eluates with DNAse I (Roche Diagnostics, Germany).
Relative recovery (RR) of the phages was calculated by dividing the concentration of phages per liter of air detected in the filter samples by the concentration of phages found in the nebulization medium (CitationHarstad 1965; CitationHapp et al. 1966; CitationTseng and Li 2005). This was done for the analysis of qPCR and culture results.
For data comparison, a mixed model analysis was performed. The random factor was linked to the samples, with a common correlation among the observations for all samples being introduced using a compound symmetric covariance structure. The univariate normality assumption was verified with the Shapiro-Wilk test. The Brown and Forsythe's variation of Levene's statistic test was used to verify the homogeneity of variances. The results were considered significant with P values ≤ 0.05. The data were analyzed using the SAS v9.1.3 statistical package program (SAS Institute Inc., Cary, NC).
COMPARISON BETWEEN BOTH FILTERS
Our qPCR results showed that PC filters were slightly better (1.2-fold) than PTFE filters for recovering aerosolized P008 phages (P < 0.05, ). Similarly, PC filters recovered 3.3-fold more phiX174 genomes than PTFE filters did (P < 0.05, ). The results of the plaque assays demonstrated that 3.1-fold more infectious P008 phages were also recovered with PC filters than with PTFE filters (P < 0.05, ). As for phiX174, PC filters were again more efficient (1.3-fold) in recovering infectious viral particles (P < 0.05, ).
FIG. 3 Relative recovery (RR) obtained with six separate aerosol samplings. Each dot represents the mean results of 3 filters analyzed twice. (a) RR of phiX174 by qPCR with PC filters is 3.3 fold higher than with PTFE filters; (b) RR of phiX174 by culture with PC filters is 1.3 fold higher than with PTFE filters; (c) RR of P008 by qPCR with PC filters is 1.2 fold higher than with PTFE filters; (d) RR of P008 by culture with PC filters is 3.1 fold higher than with PTFE filters. All results are statistically significant with p ≤ 0.05.
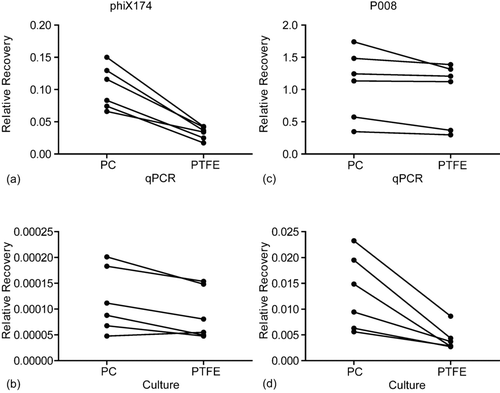
Thus, the qPCR and plaque assays indicated a better physical collection efficiency of phages P008 and phiX174 with PC filters as compared to PTFE filters. This is likely due to the structure of the filters. Scanning electron micrographs () show that PTFE filters have complex microscopic structures in which tailed phages could be trapped or broken during sampling. In contrast, PC filters have a seemingly smooth surface that is possibly less inclined to trap phages.
COMPARISON BETWEEN QPCR AND PLAQUE ASSAYS
The most striking air sampling result, obtained with both types of filter, was that most of the recovered aerosolized phages were no longer infectious, but their genome was intact enough to allow detection by qPCR. For coliphage phiX174, there were approximately 5 logs less infective viruses than total genomes recovered from the PC and PTFE filters. For the lactococcal phage P008, the difference was of approximately 2 logs. Infectivity in the nebulization medium was 1.2% for phiX174 and 2.4% for P008. Although the very low RH conditions could be responsible for the differences in infectivity of the viruses, no speculations can yet be made since only one RH condition was tested. Therefore, the reason for the low recovery of infectious phiX174 phages is still unclear and needs further investigation.
COMPARISON BETWEEN PHIX174 AND P008
The two model phages used in this study differ quite significantly from the standpoint of both genetics and morphology. Phage phiX174 has a small ssDNA genome packaged into a small icosahedral protein shell (27 nm), while phage P008 has a larger dsDNA genome encapsidated into a larger isometric capsid (53 nm) that is connected to a non-contractile tail (159 nm). Yet, both types of filter were able to recover a significant amount of aerosolized viral particles (based on detection by qPCR data).
CONCLUSIONS
Taken together, our results showed that PC filters have a slight advantage over PTFE filters in recovering two model viruses in aerosols. We also undoubtedly demonstrated that the overall recovery of airborne viruses should be estimated based on the presence of genetic material rather than on viral infectivity to establish the presence or absence of the airborne virus sought. The use of molecular biological methods is more likely to avoid false negative results than infectivity assays for the detection of airborne viruses. Further investigations should be conducted to evaluate the significance of the airborne concentrations of viral genomes and to determine the true infectivity potential of these viruses.
We are grateful to S. Simard for statistical analysis and to D. Tremblay and M. Veillette for technical assistance. C.D. received funding from the Natural Sciences and Engineering Research Council of Canada (NSERC). C.D. is also the recipient of an FRSQ Junior 2 scholarship. This work was funded by a joint grant from FQRNT-NOVALAIT-MAPAQ and Agriculture and Agri-Food Canada.
REFERENCES
- Burton , N. C. , Grinshpun , S. A. and Reponen , T. 2007 . Physical Collection Efficiency of Filter Materials for Bacteria and Viruses . Ann. Occup. Hyg. , 51 : 143 – 151 .
- Dahlgren , C. M. , Decker , H. M. and Harstad , J. B. 1961 . A Slit Sampler for Collecting T3 Bacteriophage and Venezuelan Equine Encephalomvelitis Virus. I. Studies with T3 Bacteriophage . Appl. Microbiol. , 9 : 103 – 105 .
- Happ , J. W. , Harstad , J. B. and Buchanan , L. M. 1966 . Effect of Air Ions on Submicron T1 Bacteriophage Aerosols . Appl. Microbiol. , 14 : 888 – 891 .
- Harstad , J. B. 1965 . Sampling Submicron T1 Bacteriophage Aerosols . Appl. Microbiol. , 13 : 899 – 908 .
- Jarvis , A. W. 1978 . Serological Studies of a Host Range Mutant of a Lactic Streptococcal Bacteriophage . Appl. Environ. Microb. , 36 : 785 – 789 .
- Jarvis , A. W. , Fitzgerald , G. F. , Mata , M. , Mercenier , A. , Neve , H. , Powell , I. B. , Ronda , C. , Saxelin , M. and Teuber , M. 1991 . Species and Type Phages of Lactococcal Bacteriophages . Intervirology , 32 : 2 – 9 .
- Mahony , J. , Deveau , H. , Mc Grath , S. , Ventura , M. , Canchaya , C. , Moineau , S. , Fitzgerald , G. F. and van Sinderen , D. 2006 . Sequence and Comparative Genomic Analysis of Lactococcal Bacteriophages jj50, 712 and P008: Evolutionary Insights into the 936 Phage Species . FEMS Microbiol. Lett. , 261 : 253 – 261 .
- Sanger , F. , Coulson , A. R. , Friedmann , T. , Air , G. M. , Barrell , B. G. , Brown , N. L. , Fiddes , J. C. , Hutchison , C. A. III , Slocombe , P. M. and Smith , M. 1978 . The Nucleotide Sequence of Bacteriophage phiX174 . J. Mol. Biol. , 125 : 225 – 246 .
- Thomas , G. 1970 . An Adhesive Surface Sampling Technique for Airborne Viruses . J. Hyg. (Lond.) , 68 : 273 – 282 .
- Tseng , C. C. and Li , C. S. 2005 . Collection Efficiencies of Aerosol Samplers for Virus-Containing Aerosols . J. Aerosol Sci. , 36 : 593 – 607 .
- Verreault , D. , Moineau , S. and Duchaine , C. 2008 . Methods for Sampling of Airborne Viruses . Microbiol. Mol. Biol. R. , 72 : 413 – 444 .
- Wichmann , H. E. , Spix , C. , Tuch , T. , Wolke , G. , Peters , A. , Heinrich , J. , Kreyling , W. G. and Heyder , J. 2000 . Daily Mortality and Fine and Ultrafine Particles in Erfurt, Germany Part I: Role of Particle Number and Particle Mass . Res. Rep. Health Eff. Inst. , 5-86 discussion 87–94