Abstract
Microbial aerosols could cause various human and animal health problems and their control is becoming a significant scientific and technological topic for consideration. Filtration is considered to be one of the main processes of the removal of biological aerosols from the air carrier minimizing bioaerosol concentration in industrial and domestic dwellings. However, with regards to biologically active particles, their removal from the air carrier does not solely solve the problem of microbial contamination of the ambient air. Considering that in some situations bioaerosol particles collected on the filter could re-enter the air carrier, some disinfection is required to ensure that no biologically active particles could possibly be removed from the filter surface and reach human occupied areas. This article describes the results of laboratory investigations involving Melaleuca alternifolia (tea tree oil) as disinfecting media for inactivation of common environmental fungal spores on the filter surface. It was found that 50% and 40% of Aspergillus niger and Rhizopus stolonifer spores, respectively, were inactivated over a period of 60 minutes. A single factor ANOVA test confirmed that the reported rates of inactivation were statistically significant (> 95%). For Rhizopus statistically significant inactivation by 31% was also observed after 30 minutes with no inactivation occurring over shorter time periods. No statistically significant inactivation occurred for Aspergillus niger for any time shorter than 60 min.
1. INTRODUCTION
Due to recent outbreak of Avian and Swine influenza viruses, an elevated risk for public health caused by airborne microbial contaminates is given a substantial attention. Fungal spores, which are usually considered as lower pathogenic microorganisms compared to viral and bacterial strains, are also capable of causing serious human and animal diseases, including respiratory allergy, asthma and rhinitis (CitationMaroni et al. 1995). A commercial heating, ventilation, and air conditioning system (HVAC) would be one of the main entry points for controlling indoor air quality (CitationAgranovski et al. 2006). However, when filters are used for collection of biological aerosols, capturing of viable particles is not sufficient for effective control of indoor air quality. Captured viable particles are able to proliferate and grow on the filter surface and, for certain parameters of filtration process, re-entry back to the gas carrier. For example, some study of survival of captured bacterial and fungal spores on the air filter found that atmospheric dust, which was simultaneously deposited on the filter surface, may serve as nutrient for fungal particles if the carrier gas humidity was relatively high (CitationMaus et al. 2001).
In an actual filtration process, as cake of collected particles builds up on the filter surface, the air velocity in the filter pores rises, resulting in an increase of the drag force acting on the particles collected on the filter. Under certain conditions, the drag force could exceed the magnitude of adhesion forces, increasing the possibility of re-entrainment of the previously captured microbial aerosols from the filter back to the air stream. CitationWeis et al. (2002) investigated the possibility of re-entrainment of viable Bacillus anthracis spores after bioterrorists' attack on the United States mail handling areas in 2001. They found that Bacillus anthracis spores could be re-aerosolized from surfaces under common office activities. CitationJankowska et al. (2000) suggested that if fungal spores grow on the HVAC filters, the actual re-entrainment rate may become even higher compared to values observed in laboratory experiments. This finding was especially relevant for ventilation system start-up situations when the air velocity may suddenly increase, causing significant particle detachment from the filter.
All of the above findings clearly indicate the crucial role of air filters as a potential site of microbial growth and a likely source of airborne microbial contamination from re-entrainment. Therefore, there is a significant need for rapid and reliable inactivation of collected particles on the filter surface.
Our previous investigations involving inactivation of bacterial aerosols (CitationPyankov et al. 2008) demonstrated that Melaleuca alternifolia, known as tea tree oil (TTO), is an effective natural material for their disinfection. However, given the more robust nature of fungal spores, the efficiency of the process in application to inactivation of fungal species required further consideration. This article is focused on performance evaluation of TTO used for inactivation of fungal spores captured on the filter surface. Due to the substantial robustness of fungal spores to physical and biological stresses, which is usually much higher compared to bacterial particles (CitationAgranovski et al. 2002), the results obtained for bacteria could not be directly used to evaluate the performance of the method for inactivation of fungal particles collected on the filter fibers. The attractiveness of this approach is based on the premise that, if successful, this method of inactivation could be used for development of cost-effective, user-friendly, and low-resistant technology, which could be incorporated into HVAC systems with minimal modification of currently used apparatus.
TTO is commonly used as a natural topical antiseptic and also as a complementary medicine for bacterial and fungal infections. Several investigations have confirmed the in-vitro activity of TTO against a wide range of common postharvest pathogens (CitationCox et al. 2001; CitationRay 2000; CitationGustafson et al. 1998; CitationHammer et al. 1999; CitationHammer et al. 2002). The proposed mechanism of antifungal action is based on the capability of TTO to change or damage functioning of fungal membranes (CitationCarson et al. 2006; CitationCox et al. 2000). CitationWilliams et al. (1993) reported that some types of TTO, which contain increased concentrations of terpinene-4-ol, display enhanced antimicrobial activity, since this compound has healing and antiseptic properties. The other investigation of time-related inactivation of microbes by tea tree oil fully supported this conclusion (CitationMay et al. 2000). In addition, the TTO vapor, produced by evaporation of oil from a TTO coated filter, has also demonstrated some ability to inhibit fungal growth and affect sporulation (CitationCarson et al. 2006; CitationInouye 2000).
Use of TTO as filter coating material is also attractive because of the fact that it could attenuate the kinetic energy when the particle collides with the fiber and thus, minimize/eliminate any possibility of particle rebounce and increase filter efficiency (CitationMullins et al. 2003; CitationBoskovic et al. 2007).
2. MATERIALS AND METHODS
2.1. Fungal cultures
Aspergillus niger was obtained from Southern Biological, Australia, and a Rhizopus sp. culture was obtained from the existing Griffith University stock. A. niger is commonly reported from indoor environments. Rhizopus stolonifer, a species of bread mold, produces sporangia that bear sporangiospores (asexual spores) (CitationTortora et al. 2002). A small amount of each fungus was transferred in a tube with 9 mL of sterile water. The tube was shaken to spread the spores over the entire amount of liquid and 150 μ L was then pipetted onto an agar in a plastic Petri dish (90 mm diameter) with following spread along the nutrient surface to achieve an even culture growth. Both Aspergillus niger and Rhizopus stolonifer were grown on a malt extract agar (MEA) medium (OXOID, CM 59) in an incubator set at 25°C for 7 days and 3–4 days, respectively. A bowl with water was placed in the incubator to keep the relative humidity at 55–65%. All preparations and experiments were conducted in a Class II biological safety cabinet (Model BH 2000, Biolab, Australia) to prevent spore release into the laboratory environment.
An average size of spores of A. niger used in the experiment was 3.5 (± 0.9) μ m × 4.5 (± 0.6) μ m (). The smallest diameter measured was 1.9, the largest 5.0 μ m. The sporangiospores of Rhizopus are slightly angular and ornamented (). The average size was 2.4 (± 0.3) μ m × 3.3 (± 0.5) μ m. The minimum and maximum diameters measured in our experiment were 1.5 μ m and 4.5 μ m, respectively.
2.2. Fiber Coating Oils
Two types of oil were used in experiments. Tea tree oil (Felton Grimwade & Bosisto's Pty Ltd, Oakleigh South, Australia) is extracted from Melaleuca alternifolia strains (native to New South Wales, Australia)—by steam distillation of the leaves. TTO may contain up to 100 separate components, mostly monoterpenes, sesquiterpenes and their alcohols, and while some components may induce allergic effects in rare cases, it is generally considered to possess a low toxicity (CitationCarson et al. 2006), especially in dilute form. The main antimicrobial agents present in the oil are terpinen-4-ol (35–45%) and 1,8-cineole (1–6%), however other components such as α-terpineol, terpinolene, and α - and γ-terpinene are also often present (CitationMay et al. 2000). TTO can be regarded as semi-volatile, however accurate volatility information is difficult to obtain, based on the multicomponent nature of TTO.
Biologically inactive light mineral oil (LMO) (Sigma Chemicals M3516) was used for determination of collected bioaerosol decay rate under influence of biologically inactive material. It has similar density, viscosity, boiling point, and surface tension to TTO and could be used to create similar physical conditions of filtration process on oil coated fibers, leaving biological activity solely for comparison against the results obtained for TTO.
2.3. Experimental Setup and Procedure
The experimental setup used in this project is shown in . Fungal aerosols were generated by our recently developed device based on aerosolization of fungal spores from cultures grown in standard 9 cm Petri dishes by vibration (CitationScheermeyer and Agranovski 2009). In brief, a 10 cm diameter, 2 Watts, 4 Ohm speaker was hermetically sealed by using soft rubber gasket to the bottom of cylindrical casing of the device (see ). A Petri dish, containing mature fungal spores (prepared according to the procedure described above), was placed directly on the speaker's surface and secured with thin flexible rubber strips. The speaker size was chosen to exactly accommodate a standard 9 cm diameter Petri dish that is placed on it, so that the Petri dish vibrated simultaneously with the speaker's membrane at different frequencies when running the experiment. A Hewlett-Packard 3312A Function Generator was used to set the amplitude and frequency of the vibrating speaker membrane to achieve the required particle concentration.
Before reaching the entry point of the aerosol chamber, the bioaerosol stream from the generator (10 L/min) was charge-equilibrated by an 85Kr sealed source (Model: 3012, TSI Inc., St. Paul, MN) and mixed with another portion of dry HEPA filtered air (11.0 L/min) to create a stream with sufficient flow rate required to sample simultaneously by 5 personal bioaerosol samplers (CitationAgranovski et al. 2002; CitationAgranovski et al. 2005) operating at flow rate of 4 L/min each. Another one liter per minute of air was allowed for an aerosol spectrometer (Model 1.108, Grimm Aerosol Technik, Ainring, Germany) used for monitoring bioaerosol concentration in the chamber to ensure uniform distribution throughout the apparatus. Such arrangement (four parallel filters) was convenient for direct comparison of a time related microbial decay results within each particular run, as all initial experimental conditions were identical. The fifth sampler was used to measure concentration of viable fungal particles in the chamber.
Relatively high efficient polypropylene fibrous filter with the fiber diameter of 12 μ m, thickness of 2 mm and packing density of 29% was used in this investigation (see ). High filtration efficiency was required to maximize the quantity of bioaerosols accumulated on the filter surface during the shortest possible time of bioaerosol.
Before each experiment, 12 mm diameter discs were cut from the filter sheet and hermetically sealed in filter holders. Four filter holders were connected to the inlets of modified personal bioaerosol samplers (CitationPyankov et al. 2007) and placed inside the aerosol chamber (see ). Samplers were located outside the chamber and operated at 4 L/min to create the bioaerosol flow through the filter holder corresponding to the face velocity of 0.6 m/s (commonly used for HVAC filters) and also for monitoring of possible fungal breakout through the filter. As discussed before, the fifth sampler was used to measure background concentration of viable fungal aerosols in the chamber. Each of the samplers was charged with 40 mL of sterile water and used for determination of a number of fungal spores passed through the filter according to the procedure described in CitationAgranovski et al. (2002).
Ten experimental runs were performed for each fungal culture used in this work. For each experimental run, firstly the filter was dry and fungal aerosols were collected directly on the filter surface. The second part of the run involved LMO supplied onto the filter surface with following utilization of coated filter for removal of bioaerosol particles. The third part of the experiment involved the biologically active TTO supplied onto the filter surface with following utilization of the filter for bioaerosol removal.
The following procedure was used for all experimental runs. A fresh dish with the fungal culture was used for each experimental run. Filter holders with four fresh sterilized filters were secured in the chamber and connected to the bioaerosol samplers. For the first part of the experiment (dry filter), the bioaerosol was generated for a period of 5 min and then the generator was stopped and the filter holders were removed from the chamber and placed in a special container inside a HEPA filtered Biohazard cabinet. The first filter was removed from the holder immediately after switching off the generator, the second filter was removed 15 min later, and third and fourth 30 and 60 min, respectively, after stopping the generation process. Immediately upon removal from the holder, each filter was placed into the glass bottle containing 50 ml of sterile water and sonicated in a sonic bath (Biolab, Clayton, VIC, Australia) for the period of 1 min to ensure that the majority of particles were transmitted from the filter to the liquid. Then, an aliquot of 0.1 mL of an appropriate 10-fold dilution of the liquid was spread on the surface of the MEA medium and placed in an incubator set at 25°C for 3–7 days. Plates were inspected daily until colonies reached a visible size and Colony Forming Units (CFU) were counted with a colony counter (Biolab, Clayton, VIC, Australia).
For the second part of the experiment involving LMO, a set of fresh filter holders containing 4 fresh sterilized filters was installed in the aerosol chamber. Before commencement of the bioaerosol supply, the oil was delivered to the filters surface through a 3-jet Collison Nebulizer (BGI Inc., Waltham, MA, USA) for the period of 300 s in the presence of the HEPA filtered air stream passing through the filter. Previous measurements have found that for both types of oil, at flow rate of 6 L/min of compressed air, the nebulizer generates droplets with an aerodynamic mean diameter of approximately 300 nm, at a concentration of approximately 1 × 1011 pcm− 3(CitationPyankov et al. 2008). The time interval of 300 s was chosen as sufficient to provide uniform fiber coating by both types of oil. This assumption was confirmed by corresponding observations of the fibers through the microscope (see ). The amount of oil per one square centimeter of the filter used in these experiments was approximately 0.9 ± 0.2 g, which was obtained by weighing 10 filter samples before and after oil coating. The remainder of the procedure was as follows; the aerosol generator was operated for 5 min to ensure a representative concentration of collected particles on the filters. Then the generator was switched off and the filters were removed at 0, 15, 30, and 60 min intervals. Immediately upon removal, each filter was placed into a glass bottle containing 50 ml of sterile water and sonicated in a sonic bath for the period of 1 min to ensure that the majority of particles were transmitted from the filter to the liquid. Then, the liquid was centrifugated at 7,000 × g for 2 min to fully separate particles from the remaining oil and eliminate the influence of oil during the remainder of the procedure. The pellet was then mixed with 50 ml of sterile water and placed in the sonic bath to ensure uniform distribution of microorganisms throughout the liquid. Then, similarly to the first part of the experiment, an aliquot of 0.1 mL of an appropriate 10-fold dilution of the liquid was spread on the surface of the MEA medium, placed in an incubator set at 25°C for 3–7 days, and Colony Forming Units were counted after incubation. The procedure for the last part of the experimental run involving TTO was exactly the same as for the LMO. Temperature and relative humidity were monitored and controlled in the chamber at levels of 25°C and 45–55%, respectively.
A time related fungal inactivation (FI t ) was calculated by the following equation:
3. RESULTS AND DISCUSSION
The results for microbial inactivation of Aspergillus niger on the filter surface are shown in . All results were normalized according to Equation (Equation1). The error bars show standard deviation of at least 10 experimental runs. Both dry and LMO coated filtration regimes did not achieve any measurable microbial inactivation. Some decrease in the number of viable particles recovered from the filters after 30 and 60 min was not statistically significant compared to the number obtained at time 0, as was confirmed by a single factor ANOVA test (p < 0.05).
FIG. 4 Microbial decay of Aspergillus niger collected on the filter. Error bars represent standard deviation of ten experimental runs.
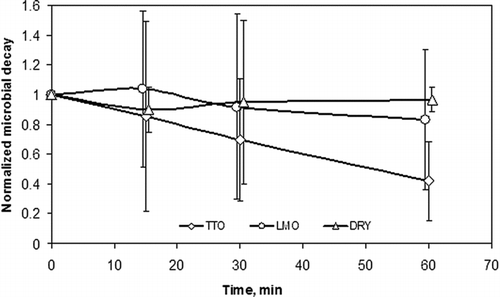
When the TTO was used for the filter coating, no inactivation of fungal particles was demonstrated for the first 30 min of experiment. Even 31% inactivation at 30 min was not statistically significant according to ANOVA test results. On the other hand, the results obtained for TTO coated filters demonstrated statistically significant fungal inactivation after 60 min of treatment. Fifty-eight percent of inactivation of fungal aerosol on the filter surface was achieved for the above time interval. It should be noted that the relatively large error bars indicate large variation and fluctuation in the inactivation over time among experiments. It could be explained by hydrophobic nature of this microorganism and possible non-uniformity of suspensions at the stage of analytical study.
The results obtained for Rhizopus are presented in . Similarly to A. niger, no inactivation was demonstrated for the dry and LMO coated filters. As is seen in the figure, graphs for both regimes coincide at the level of zero inactivation throughout the entire experimental time interval of 60 min. A statistical insignificance of the difference between the results was also confirmed by ANOVA test.
FIG. 5 Microbial decay of Rhizopus stolonifer collected on the filter. Error bars represent standard deviation of ten experimental runs.
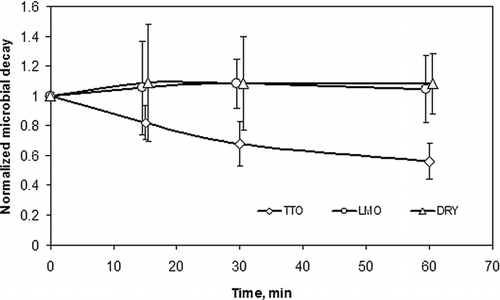
Using of TTO for treatment of Rhizopus did not lead to any success for the first 15 min of experimental run, with no difference between the results demonstrated by ANOVA test for this time period. However, compared to A. niger, the first statistically significant inactivation of Rhizopus was achieved after 30 min of treatment, making thirty two percent of fungal particles inactive. After 60 min of TTO action, the total percentage of inactivated particles of Rhizopus reached 56%.
The above results indicate that even robust fungal aerosols could be inactivated by TTO. However, as was shown, a time related efficiency of inactivation is much lower compared to our previously reported results for inactivation of bacterial aerosols (CitationPyankov et al. 2008) when less than 10% survival was demonstrated for robust B. subtilis bacterial strain after 30 min of treatment. Moreover, the efficiency of inactivation of more sensitive bacterial aerosols of E.coli and P. fluorescens was reported at the level above 99% for the same time interval of 30 min.
Obviously, extended periods of time necessary to inactivate fungal particles on the filter surface suggest that advanced technology would be required to remove fungal aerosols from the air using filtration process. On the other hand, as discussed before, thin coating film of TTO enhances adhesion between collected particle and filter media, thereby decreasing the likelihood that deposited particles would be re-entrained.
A small experiment was performed to evaluate possible re-entrainment of viable fungal particles from the TTO coated filter. The same laboratory setup (see ) was used to generate fungal particles and TTO mist. A fresh filter was installed in the holder, and the holder was connected to a critical orifice controlled vacuum pump operated at the standard flow rate of 4 L/min. Firstly fungal aerosols and then TTO mist were generated and collected on the filter similarly to our previously described procedure. On completion of collection of fungal particles and TTO mist, the vacuum pump was disconnected and either two, three or four samplers were connected to the filter holder by using specially made manifold capable to accommodate the required number of samplers operated in parallel. This procedure allowed evaluating possible fungal re-entrainment at the range of velocities covering the entire operational range used for HVAC systems. Use of multiple samplers was required as the maximum flow rate through each device, due to geometrical limitations, cannot exceed 5 L/m. The analytical procedure described before was used for analysis of samplers' collecting liquid to identify any presence of viable particles re-entrained from the filter to the air carrier. The results of this experiment look very encouraging as no viable fungal particles were detected in the samplers for all three repeats of the experiment.
4. CONCLUSIONS
Indoor HVAC systems could by colonized by a number of various species of collected bacterial and fungal particles. Many of those microorganisms could potentially cause infectious diseases such as allergic rhinitis, asthma and hypersensitivity (CitationMaroni et al. 1995). To minimize or even eliminate the possibility of re-entrainment of viable species from the filter surface back to the air carrier, a new concept utilizing coating of filter fibers by TTO has been suggested. It was shown that using of TTO as coating media could provide two major benefits; firstly, it has some microbial disinfecting properties which inactivate collected microorganisms on the filter preventing them from reaching dwelling's ambient air in viable form. Secondly, additional adhesion provided by the oil film minimizes the possibility of particle re-entrainment, thus keeping them on the filter even at the air velocities exceeding common HVAC operational parameters by a factor of four.
REFERENCES
- Agranovski , I. , Agranovski , V. , Grinshpun , S. , Reponen , T. and Willeke , K. 2002 . Development and Evaluation of a New Personal Sampler for Viable Airborne Microorganisms . Atmos. Environ. , 36 : 889 – 898 .
- Agranovski , I. , Safatov , A. , Borodulin , A. , Pyankov , O. , Petrishchenko , V. , Sergeev , A. , Sergeev , A. A. , Grinshpun , S. and Agranovski , V. 2005 . New Personal Sampler for Viable Airborne Viruses: Feasibility Study . J. Aerosol Sci. , 36 ( 5–6 ) : 609 – 617 .
- Agranovski , I. E. , Huang , R. , Pyankov , O. V. , Altman , I. S. and Grinshpun , S. A. 2006 . Enhancement of the Performance of Low-Efficiency HVAC Filters Due to Continuous Unipolar Ion Emission . Aerosol Sci. Tech. , 40 ( 11 ) : 963 – 968 .
- Boskovic , L. , Agranovski , I. E. and Braddock , R. D. 2007 . Filtration of Nanosized Particles with Different Shape on Oil Coated Fibres . J. Aerosol Sci. , 38 ( 12 ) : 1220 – 1229 .
- Carson , C. F. , Hammer , K. A. and Riley , T. V. 2006 . Melaleuca alternifolia (Tea Tree) Oil: A Review of Antimicrobial and Other Medicinal Properties . Clin. Microbiol. Rev. , 19 ( 1 ) : 50 – 62 .
- Cox , S. D. , Mann , C. M. , Markham , J. L. , Bell , H. C. , Gustafson , J. E. , Warmington , J. R. and Wyllie , S. G. 2000 . The Mode of Antimicrobial Action of the Essential Oil of Melaleuca alternifolia (Tea Tree Oil) . J. Appl. Microbiol , 88 ( 1 ) : 170 – 175 .
- Cox , S. , Mann , C. , Markham , J. , Gustafson , J. , Warmington , J. and Wyllie , S. 2001 . Determining the Antimicrobial Actions of Tea Tree Oil . Molecules , 6 ( 2 ) : 87 – 91 .
- Gustafson , J. E. , Liew , Y. C. , Chew , S. , Markham , J. , Bell , H. C. , Wyllie , S. G. and Warmington , J. R. 1998 . Effects of Tea Tree Oil on Escherichia coli . Lett. Appl. Microbiol. , 26 ( 3 ) : 194 – 198 .
- Hammer , K. A. , Carson , C. F. and Riley , T. V. 1999 . Antimicrobial Activity of Essential Oils and Other Plant Extracts . J. Appl. Microbiol. , 86 ( 6 ) : 985 – 990 .
- Hammer , K. A. , Carson , C. F. and Riley , T. V. 2002 . In Vitro Activity of Melaleuca alternifolia (Tea Tree) Oil Against Dermatophytes and Other Filamentous Fungi . Journal of Antimicrobial Chemotherapy , 50 : 195 – 199 .
- Inouye , S. , Tsuruoka , T. , Watanabe , M. , Takeo , K. , Akao , M. , Nishiyama , Y. and Yamaguchi , H. 2000 . Inhibitory Effect of Essential Oils on Apical Growth of Aspergillus Fumigatus by Vapour Contact . Mycoses , 43 ( 1–2 ) : 17 – 23 .
- Jankowska , E. , Reponen , T. , Willeke , K. , Grinshpun , S. A. and Choi , K.-J. 2000 . Collection of Fungal Spores on Air Filters and Spore Re-Entrainment from Filters Into Air . J. Aerosol Sci. , 31 ( 8 ) : 969 – 978 .
- Maroni , M. , Seifert , B. and Lindvall , T. 1995 . Indoor Air Quality; A Comprehensive Reference Book (Vol. 3) , Amsterdam : Elsevier .
- Maus , R. , Goppelsroder , A. and Umhauer , H. 2001 . Survival of Bacterial and Mold Spores in Air Filter Media . Atm. Env. , 35 : 105 – 113 .
- May , J. , Chan , C. H. , King , A. , Williams , L. and French , G. L. 2000 . Time-Kill Studies of Tea Tree Oils on Clinical Isolates . J. Antimicrob. Chemother. , 45 ( 5 ) : 639 – 643 .
- Mullins , B. , Agranovski , I. and Braddock , R. 2003 . Particle Bounce During Filtration of Particles on Wet and Dry Filters . Aerosol Sci. Tech. , 37 : 587 – 600 .
- Pyankov , O. , Agranovski , I. , Pyankova , O. , Mokhonova , E. , Mokhonov , V. , Safatov , A. and Khromykh , A. 2007 . Using Bioaerosol Personal Sampler in Combination with Real-Time PCR Analysis for Rapid Detection of Airborne Viruses; Feasibility Study . Envir. Microbiology , 9 : 992 – 1000 .
- Pyankov , O. , Agranovski , I. , Huang , R. and Mullins , B. 2008 . Removal of Biological Aerosols by Oil Coated Filters . CLEAN—Soil, Air, Water , 36 ( 7 ) : 609 – 614 .
- Ray , M. B. 2000 . Photodegradation of the Volatile Organic Compounds in the Gas Phase: A Review . Dev. Chem. Eng Min Process. , 8 ( 5–6 ) : 405 – 439 .
- Scheermeyer , E. and Agranovski , I. E. 2009 . Design and Evaluation of a New Device for Fungal Spore Aerosolization for Laboratory Applications . J. Aerosol Sci. , 40 : 879 – 889 .
- Tortora , G. J. , Funke , B. R. and Case , C. L. 2002 . Microbiology: An Introduction (Media Update) , Benjammin Cummings .
- Weis , C. P. , Intrepido , A. J. , Miller , A. K. , Cowin , P. G. , Durno , M. A. , Gebhardt , J. S. and Bull , R. 2002 . Secondary Aerosolization of Viable Bacillus Anthracis Spores in a Contaminated US Senate Office . Jama , 288 ( 22 ) : 2853 – 2858 .
- Williams , L. R. , Home , V. N. and Lusunzi , I. 1993 . An Evaluation of the Contribution of Cineole and Terpinen-4-ol to the Overall Antimicrobial Activity of Tea Tree Oil . Cosmetic, Aerosols and Toiletries in Australia , 7 : 25 – 28 .