Abstract
Filtration efficiency of multi-walled carbon nanotube (MWCNT) aerosol by fibrous filter was evaluated experimentally. Mono-mobility test aerosols with electrical mobility diameter of 100, 200, and 300 nm were generated by the atomization of MWCNT aqueous suspension followed by mobility classification with a differential mobility analyzer (DMA). By analyzing the shape of classified aerosol particles under a scanning electron microscope, it was found that the DMA-classified 300 nm particles were fibrous in shape and had uniform diameter of about 60 nm and length of 2.1 micrometer. On the other hand, 100 nm and 200 nm particles contained a fairly large amount of multiply charged fibrous particles with a larger diameter. These test aerosols were challenged to a medium performance fibrous filter at various filtration velocities. As a result, fibrous particles were captured by fibrous filter at a higher collection efficiency than the spherical particles with the same mobility. By analyzing the single fiber capturing efficiency, interception incorporating the rotation of fibrous particles is found to be the dominant capturing mechanism for the fibrous particles in the studied size range.
INTRODUCTION
Air filtration is one of the most effective means to protect industrial workers from exposure to airborne nanoparticles. Numerous studies were conducted to investigate the filtration of aerosol by fibrous filters (CitationHinds 1982). According to the conventional filtration theory, airborne particles are captured by mechanical collection mechanisms such as Brownian diffusion, inertia, gravity, and interception in the absence of electrical effects. However, conventional filtration theory has not been fully tested against nanometer-sized particles, especially for fibrous nanoparticles such as carbon nanotubes (CNTs) and nanowires. CNTs are one of the most promising nanomaterials which have great potential applications for developing new devices in various fields due to unique physical and chemical properties that cannot be achieved by bulk materials (CitationIijima 1991). On the other hand, recently, toxicology and risk assessment of CNT aerosol is a matter of serious concern (CitationLam et al. 2006; CitationWarheit 2009; CitationEllinger-Ziegelbauer and Pauluhn 2009). In order to conduct inhalation and filtration studies, it is key to develop generation methods and characterization techniques of airborne CNT. Recently, CitationBaron et al. (2008) generated aerosolized single-walled CNT (SWCNT) for inhalation study using mill and cyclone generator. CitationMyojo et al. (2009) also used a rotating brush aerosol generator and they controlled the number concentration of multi-walled CNT (MWCNT) aerosol up to 20,000 particles cm–3. They succeeded in aerosolizing commercial MWCNT powder for an exposure study of mice, but the size distribution of generated test particles was too broad and filtration efficiency of these aerosols was not investigated.
The aerodynamics of airborne CNTs are also of great interest since they have a diameter in free molecular flow regime and a length in continuum flow regime. Filtration of CNTs would be a good means to study the aerodynamics of CNTs because air filters collect particles by various collection mechanisms and the dominant collection mechanisms can be easily found by measuring the collection efficiency at various particle sizes and filtration velocities.
Theoretical prediction of filtration efficiency of fibrous aerosols was conducted by several research groups mainly in relation to asbestos in 1980s. CitationGradon et al. (1988) numerically analyzed the motion and the deposition of fibrous particles onto single fibers. They investigated the single fiber capturing efficiency by fibrous filter mainly due to interception. CitationSpurny (1986) provided experimental collection efficiency of asbestos for fibrous and nuclepore filters. He showed a higher possibility of fibrous aerosol passing through a filter especially at a lower filtration velocity. CitationAsgharian and Cheng (2002) analyzed the trajectories of fibrous aerosol particles which rotate by a shear force in a flow field. They derived the single fiber capturing efficiency by inertia and interception as a function of Stokes number and the aspect ratio of fibrous particles. Although these previous works provide some insight in aerodynamics of fibrous particles, uncertainty still remains in the aerodynamics of one-dimensional nanostructured objects such as carbon nanotubes.
More recently, regarding carbon nanotubes and nanowires, CitationKim et al. (2007) generated MWCNT aerosol particles of controlled diameter and length by laser ablation together with an aerosol classification technique. They investigated the Brownian rotation and electrostatic alignments of MWCNT aerosol in a differential mobility analyzer (DMA) and provided clear relationship between the mobility and the geometric size of MWCNT. However, no studies of the filtration efficiency of MWCNT aerosols have been reported.
In this work, mono-mobility MWCNT aerosol was generated by the atomization of MWCNT aqueous suspension followed by the classification with DMA, and the filtration efficiency of fibrous aerosol was measured at various filtration velocities. The single fiber collection efficiency was compared with those predicted by given capturing mechanisms such as diffusion, inertia, gravity, and interception in order to find the governing collection mechanisms.
Preparation of Test Fibrous Aerosol
The test aerosol was generated by atomizing aqueous suspension of MWCNT. shows a schematic of the experimental setup used in this study. The MWCNT suspension was prepared by a method developed by AIST, Japan. In this method, the solid mixture of fructose and commercial MWCNT powder (Nikkiso Co. Ltd.) was mechanically milled. After removing impurities and fructose by liquid filtration and rinsing, a centrifugal separation was employed to obtain uniformly dispersed MWCNT suspension. The suspension was diluted with the ultra pure water to a given concentration (approximately 0.0125 to 0.05 wt %) for stable atomization.
A laboratory made Collison-type atomizer was used for aerosolization of the MWCNT suspension. The atomized water droplets containing MWCNT were dried by passing through a diffusion dryer with the flow rate of 3 L/min. The electrical mobility distribution of generated MWCNT aerosol was measured by a scanning mobility particle sizer (SMPS, TSI Model 3936). The sheath gas flow rate of the differential mobility analyzer (DMA; TSI long column) was varied for each experiment. For scanning on whole size distribution range of generated aerosol, 15 <Dp < 700 nm, the sheath gas flow rate was 3.0 L/min and aerosol flow rate was 0.3 L/min. For the generation of test aerosol with a given electrical mobility, such as in the case of generating filtration test aerosol and sampling MWCNT aerosol by membrane filter, the flow rates of the sheath gas and aerosol were, respectively, 10 and 1.5 L/min. The polydispersed and DMA-classified aerosols were collected onto a polycarbonate membrane filter with pore size of 100 nm (K010A025A, Advantec Co. Ltd.), and the geometric diameter and length of the MWCNT were measured by a scanning electron microscope (SEM, Hitachi S-4500).
shows the size distribution of generated aerosols measured by SMPS. When ultrapure water is atomized, a small peak appears at around 20 nm and almost no particles exist at a mobility diameter larger than 40 nm. The peak would be the residue particles from the pure water. When MWCNT suspension is atomized, the size distribution is bimodal with an additional peak between 200 and 400 nm. The second peak shifts to a larger size as the concentration of the MWCNT suspension increases, while the first peak becomes higher without a shift in particle size. The peak in the size range smaller than 100 nm is considered to be contaminants in the suspension, such as surfactants, amorphous carbon, and catalysts which were used to grow the MWCNT. shows a scanning electron micrograph of generated MWCNT, which were sampled by a membrane filter with the pore size of 100 nm. There are many micron-sized fibrous particles with small spherical particles. is a plot of length, Lf , against diameter, Df , of individual fibrous particles. It is seen from this figure that MWCNT particles have fairly uniform diameter of around 60 nm, but that the length is widely distributed from 200 nm to over 5 micrometer. In comparing the mobility equivalent diameter of MWCNT which is about 300 nm as shown in , the fibrous particle with Df = 60 nm and Lf = 1178 nm gives the projected area equivalent diameters of 300 nm according to the definition of Heywood diameter, D prj , (= ((4/π)Lf Df )0.5), Consequently, we may say that that the Heywood diameter D prj is roughly equal to the mobility equivalent diameter, as was reported by CitationKim et al. (2007). In the following filtration experiments, we used DMA-classified MWCNT particles with mobility equivalent diameters of 100, 200, and 300 nm as nominal size. However we found that the DMA-classified MWCNT particles in this size range, especially 100 and 200 nm particles, contain significant amount of multiply charged particles.
FIG. 2 Electrical mobility size distribution of generated aerosol with changing concentration of MWCNT-water suspension.
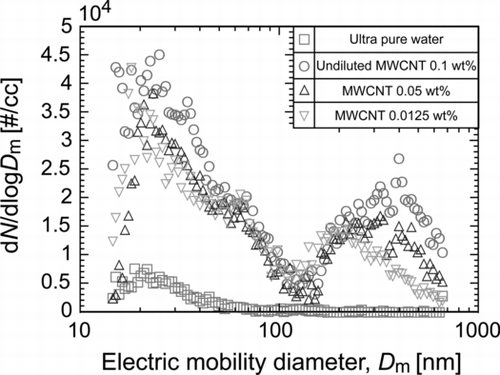
FIG. 4 The measured length, Lf of individual fibrous particles plotted against corresponding diameter, Df .
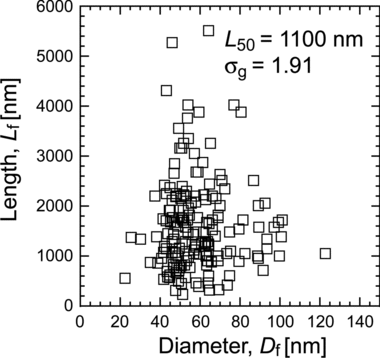
are scanning electron micrographs of DMA-classified MWCNT particles with mobility equivalent diameters of (a) 100, (b) 200, and (c) 300 nm, respectively. In order to avoid overlapping of MWCNT particles collected on the filter, the sampling time was adjusted according to the number concentration of MWCNT so that each viewing area of SEM contained only one or two MWCNT particles. By measuring more than 50 MWCNT particles, we obtained the length distributions of DMA-classified particles shown in . Since the diameters of raw MWCNT particles are almost uniform at around 60 nm as shown in , the difference in electrical mobility reflects the difference in length of MWCNT particles. clearly shows that DMA-classified 100 nm particles contain significant amount of multiply charged particles and that the length distribution is very similar to that of raw MWCNT particles (). The fractions of multiply charged particles were measured using a tandem DMA technique (preliminary results are provided in the supplementary information), and more than 50%, 29%, and 20% were multiply charged for 100 nm, 200 nm, and 300 nm particles, respectively.
FIG. 5 Scanning electron micrographs of DMA-classified particles with the mobility diameters of (a) 100, (b) 200, and (c) 300 nm.
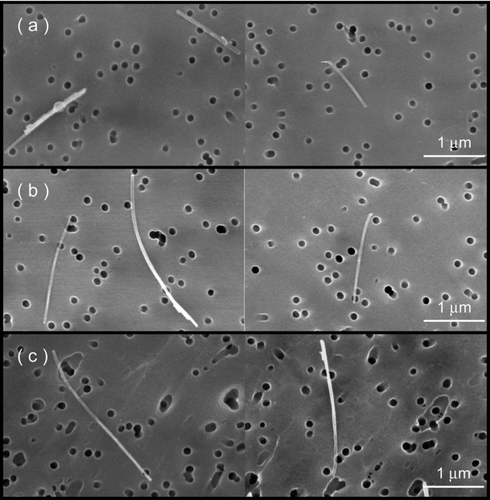
FIG. 6 Length distribution of DMA-classified MWCNT particles obtained from SEM images. The mobility diameters are (a) 100, (b) 200, and (c) 300 nm.
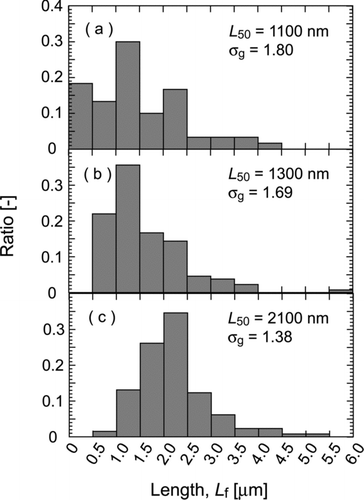
The distributions of Heywood diameter of raw MWCNT particles are shown in . As expected from the length distribution of , the Heywood diameter of DMA-classified 100 nm particles is widely distributed. The various characteristics of DMA-classified MWCNT particles are listed in . Only 300 nm MWCNT particles can be said to be monodispersed since the geometric standard deviation is 1.12. In , we also see the difference between the mobility diameter, Dm , and the projected area equivalent diameter, D prj . CitationKim et al. (2007) derived a relationship between Dm and D prj by considering the electrophoretic orientation of MWCNT particles in the DMA. They obtained following relationship experimentally as,
FIG. 7 Distributions of Heywood diameter of DMA-classified particles. The mobility diameters are (a) 100, (b) 200, and (c) 300 nm.
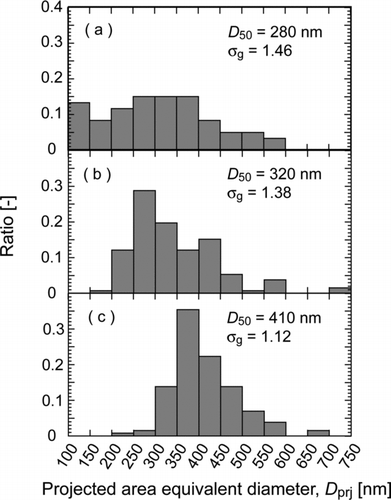
TABLE 1 Characteristics of DMA-classified MWCNT particles
The mobility diameters calculated from the average diameter and length of MWCNT by this equation are shown in the first column from the right of . The calculated mobility diameters (244, 272, and 344 nm) are larger than those of nominal mobility diameters (100, 200, and 300 nm) due to the fraction of multiply charged particles with larger size.
Filtration of Fibrous Aerosol
The filtration efficiencies of DMA-classified MWCNT particles through a fibrous filter were measured at various filtration velocities. shows the experimental setup for measuring the penetration of test aerosols. For a comparison of fibrous MWCNT particles, spherical polystyrene latex (PSL) standard particles were also generated by atomizing PSL suspension by a Collison atomizer. The flow rates of sheath gas and aerosol for the size classification by DMA were set at 10 and 1.5 L/min, which are the same as those for the characterization experiments of MWCNT particles. The generated particles were then electrically neutralized by 241Am neutralizer. The charged particle fractions remaining from the neutralization were removed by a parallel plate electrode condenser in order to eliminate the effect of electrostatic precipitation.
A medium performance glass fiber filter with average fiber diameter of 2.8 μm was used as a test filter. The packing density and the thickness are, respectively, 0.049 and 0.38 mm. The penetration through the filter was measured using two condensation particle counters (CPC; TSI model 3022A), which were connected to the inlet and outlet of test filter. The difference in the counting efficiency between two CPCs used in the experiments was calibrated by simultaneously measuring the number concentration of DMA-classified particles by two CPCs connected in parallel. The filtration velocities were changed from 0.05 to 0.5 m/s by changing the flow rate of clean dry air which was supplied from the top of filtration test apparatus for dilution.
shows the penetrations of (a) test fibrous MWCNT aerosol and (b) spherical PSL aerosol, respectively. The penetrations 200 and 300 nm particles of MWCNT are corrected with considering multiply charged fractions (raw data and correction procedures are shown in supplemental information). Note that the penetrations of particles with the mobility diameter of 30 and 50 nm in are those of contaminants as explained in . In the size range of Dm
< 100 nm, the penetration decreases with lowering both mobility diameter and filtration velocity, indicating that the dominant collection mechanism is Brownian diffusion. The penetration curves of Dm
< 100 nm in are almost the same as those of PSL particles in because the particles of Dm
< 100 nm produced from MWCNT suspensions in are not fibrous particles. The most penetrating particle sizes (MPPS) are 100 or 200 nm for both MWCNT particles and spherical PSL particles. When the particle size exceeds 200 nm, the penetration decreases with increasing particle size and decreasing the filtration velocity, suggesting that the gravity would be the predominant collection mechanism. By comparing Figures and b, the penetrations of 300 nm MWCNT particles are considerably lower than the spherical particles with the same electrical mobility diameters. In , the differences in penetration of 200 and 300 nm PSL particles due to filtration velocity diminish, whereas, in , those of MWCNT particles are significant. It is worth noting that the penetrations of MWCNT particles are lower than those of spherical particles of the same Heywood diameter (the mobility equivalent diameters Dm
= 100, 200, 300 nm correspond, respectively, to Heywood diameters 230, 320, 410 nm).
FIG. 9 Penetration curves of (a) MWCNT particles and (b) spherical PSL particles through medium performance filter. The solid lines are experimental curves.
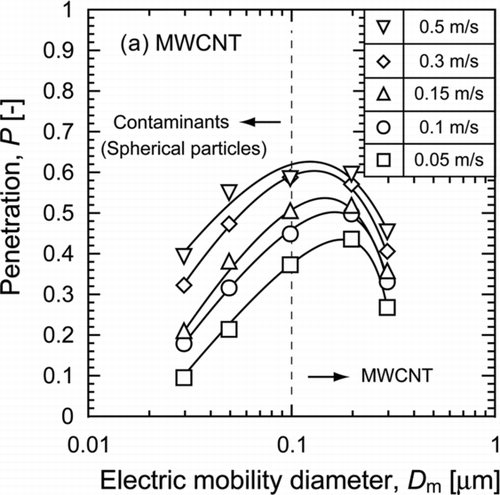
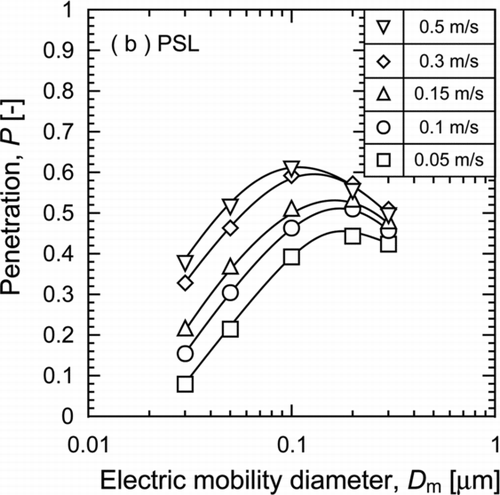
In order to find the filtration mechanisms, the experimental single fiber efficiencies, η, were obtained from the penetration by the following log-penetration law;
FIG. 10 Single fiber capturing efficiencies of fibrous particles with those predicted by interception.
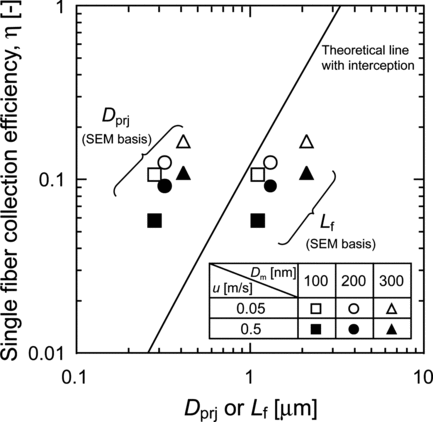
Because of the penetration dependence on particle size and filtration velocity, we first suspected that gravity would be the dominant collection mechanism. The single fiber efficiency due to gravitational settling for downward flow, η G , is given by (CitationTakahashi 1988)
Secondly, we assumed that interception would be the dominant collection mechanism. The single fiber efficiency due to interception is given by (CitationTakahashi 1988)
CitationAsgharian and Chen (2002) calculated the filtration efficiency of fibrous aerosol due to inertia and interception by considering the rotation of fibrous particles in a shear flow around a fiber. They reported that the fibrous particles align along the stream line upstream of a fiber but they may rotate by the shear force in the vicinity of a fiber. The inertial collection efficiency is expected to increase with the aspect ratio because longer fibers have larger mass. However they did not consider the effect of orientation on the interceptional collection because they treated relatively large particles. For the experimental parameters assigned in the present study, the Stokes number is calculated to be much smaller than theircase: 0.01 < Stk < 0.1, and the interception parameter based on the fiber diameter is one order of magnitude smaller than their case, i.e., R≈ 0.02. Therefore, our experimental conditions are not in the range of inertia. From these comparisons, it is considered that MWCNT particles may rotate or vibrate by the bombardments of gas molecules and that the shear force exerted on the fiber may suppress the rotation and vibration depending on the aspect ratio.
The preferred orientation of MWCNT fibers can be estimated simply by setting the particle diameter Dp in Equation (Equation5) as:
where θ is the orientation angle measured from the main stream direction. The estimated preferred orientation angles, θ, are shown in as a function of the mobility diameter and the filtration velocity. The angle lies between 40 and 60 degrees and it decreases with increasing particle size (i.e., length) and increasing the filtration velocity, which is quite reasonable because a longer fiber needs more torque to rotate and larger shear forces are exerted on fibrous particles at a higher velocity. However, the reason of such large orientation angle: 40°<θ< 60°, is still uncertain. This phenomenon might be explained by further investigation on the rotation dynamics of fibrous aerosols.
TABLE 2 Orientation angle, θ, obtained by fitting the experimental data
CONCLUSIONS
Three sizes of test MWCNT particles were successfully generated by atomizing MWCNT aqueous suspension followed by the mobility classification with DMA. The DMA-classified 100 nm and 200 nm particles contained a fairly large fraction of multiply-charged fibrous particles with a larger length. The 300 nm particles were monodisperse in length so that it is suitable for studying aerodynamics of fibrous particles with nanometer-sized diameters. These test aerosols were applied to evaluate the filtration efficiency of MWCNT aerosol. It was found that 300 nm MWCNT particles are more effectively captured by fibrous filter compared to the spherical particles with the same mobility. Furthermore, the interception accompanied with the preferred orientation of fibrous particles was found to be the dominant capturing mechanism for fibrous aerosol in this size range.
The authors are grateful to Mr. Yuki Takeuchi for his assistance in building the experimental setup. This work was supported by New Energy and Industrial Technology Development Organization of Japan (NEDO) Grant, “Evaluating risks associated with manufactured nanomaterials (P06041).”
uast_a_487881_sup_14325016.zip
Download Zip (40.4 KB)[Supplementary materials are available for this article. Please go to the publishers online edition of Aerosol Science and Technology to view the free supplementary files.]
REFERENCES
- Asgharian , B. and Cheng , Y. S. 2002 . The Filtration of Fibrous Aerosols . Aerosol Sci. Technol. , 36 : 10 – 17 .
- Baron , P. A. , Deye , G. J. , Chen , B. T. , Schwegler-Berry , D. E. , Shvedova , A. A. and Castranova , V. 2008 . Aerosolization of Single-Walled Carbon Nanotubes for an Inhalation Study . Inhal. Toxicol. , 20 ( 8 ) : 751 – 760 .
- Ellinger-Ziegelbauer , H. and Pauluhn , J. 2009 . Pulmonary Toxicity of Multi–Walled Carbon Nanotubes (Baytubes) Relative to α -Quartz Following a Single 6 h Inhalation Exposure of Rats and a 3 Months Post-Exposure Period . Toxicol. , 266 : 16 – 29 .
- Gradon , L. , Grzybowski , P. and Pilacinski , W. 1988 . Analysis of Motion and Deposition of Fibrous Particles on a Single Filter Element . Chem. Eng. Sci. , 43 ( 6 ) : 1253 – 1259 .
- Hinds , W. C. 1982 . Aerosol Technology , 164 New York : Wiley-Interscience .
- Iijima , S. 1991 . Helical Microtubules of Graphitic Carbon . Nature , 354 : 56 – 58 .
- Kim , S. H. , Mulholland , G. W. and Zachariah , M. R. 2007 . Understanding Ion-Mobility and Transport Properties of Aerosol Nanowires . J. Aerosol Sci. , 38 : 823 – 842 .
- Lam , C. W. , James , J. T. , McCluskey , R. , Arepalli , S. and Hunter , R. L. 2006 . A Review of Carbon Nanotube Toxicity and Assessment of Potential Occupational and Environmental Health Risks . Crit. Rev. Toxicol. , 36 ( 3 ) : 189 – 217 .
- Myojo , T. , Oyabu , T. , Nishi , K. , Kadoya , C. , Tanaka , I. , Ono-Ogasawara , M. , Sakae , H. and Shirai , T. 2009 . Aerosol Generation and Measurement of Multi-Wall Carbon Nanotubes . J. Nanoparticle Res. , 11 : 91 – 99 .
- Spurny , K. R. 1986 . On the Filtration of Fibrous Aerosols . Sci. Total Environ. , 52 : 189 – 199 .
- Takahashi , T. 1988 . Theory of Air Filtration and Electrostatic Effects . Earozoru kenkyu , 3 ( 4 ) : 283 – 291 .
- Warheit , D. B. 2009 . Long-Term Inhalation Toxicity Studies with Multiwalled Carbon Nanotubes: Closing the Gaps or Initiating the Debate? . Toxicol. Sci. , 112 ( 2 ) : 273 – 275 .