Abstract
Pulmonary delivery of sustained release formulations needs drug encapsulation in a suitable matrix, as well as the generation of aerosols with high lung penetration and suitable release characteristics. Nanometer sized liposomes offer the potential for biocompatibility, controlled release and easy internalization in the lung. For uniform dose delivery and drug release kinetics, it is of interest to understand generation techniques to obtain aerosols containing nearly monodispered nanometer sized dry particles. Two aerosolization techniques, air-jet atomization and electrohydrodynamic atomization (EHDA) were studied to identify conditions under which the inclusion of one-liposome-per-drop could be achieved. In air-jet atomization, low lipid concentrations resulted in a unimodal aerosol with a median mobility diameter of 94 (± 3.5) nm, while higher concentrations led to larger median diameters, implying possible inclusion of multiple liposomes per drop. In EHDA, tuning drop sizes in the range of 130 to 200 nm, as well as the use of high lipid concentrations, resulted in a bimodal aerosol distribution, with peaks at 35 and 100 nm mobility diameters. TEM images of the liposome aerosol from EDHA showed fused liposomes, resulting in cylindrical structures with different physical diameters. It was hypothesized that deformation of liposomes to cylindrical structures in the micro-capillary liquid tip of the electrospray, and interactions along the axial or cross sectional surfaces led to dry particles with different mobility sizes.
1. INTRODUCTION
Introduction of drugs via inhalation is a potential non-invasive delivery mechanism. Pulmonary targeting finds application in delivery of drugs whose site of action is the lung (CitationWong et al. 2003), as well as in delivery of systemic drugs such as Insulin (CitationHuang and Wang 2006) and Interleukin 2 (IL-2) (CitationTen et al. 2002). One method to target the lung involves drug encapsulation in biocompatible nanometer-size matrices to obtain drug dispersity, higher bioavailability, controlled release properties, and enzymatic tolerance (CitationChattopadhyay et al. 2007). Nanometer sized carriers used for drug delivery include polymeric nanoparticles (CitationFu et al. 2002), solid lipid nanoparticles (CitationTriplett-II 2004), and liposomes (CitationHuang and Wang 2006). Liposomes are self-assembled structures made of phospholipids, which have been studied for controlled release of insulin (CitationHuang and Wang 2006) and various anti-cancer drugs (CitationGiovanella 2008) to the lungs.
Issues in liposome encapsulation for pulmonary targeting include production of dry particles, which would have high deposition efficiency in the pulmonary lung (CitationDesai et al. 2003), and leakages of drugs from rupture of liposomes during aerosolization (CitationKleemann et al. 2007). There are two modes in the pulmonary deposition efficiency of inhaled particles, one between 50 to 200 nm (CitationRogueda and Traini 2007) and the conventionally used particle mode between 1 and 3 μ m (CitationBalásházy et al. 2000; CitationDandekar et al. 2009; CitationHofmann and Koblinger 1990). Advantages of using nanometer sized particles include rapid dissolution in the alveolar fluid and preferential internalization by pulmonary epithelial cells, possibly aiding bioavailability (CitationBailey and Berkland 2009). It would be of interest to explore the generation of nanometer sized liposome particles for drug delivery and to understand changes in their size distribution and morphology during aerosol generation. Widely used aerosol generation techniques such as air jet atomization generated polydisperse dry particles of 1–5 μ m diameter (CitationKleemann et al. 2007) when applied to liposome suspensions of in-situ diameter 100–500 nm. Generation of large dry particles results from the inclusion of multiple liposomes in single atomized droplets and from the presence of salts used to buffer liposomes in suspension.
Aerosols containing a uniformly sized particle matrix, which carry a drug or a single drug-containing matrix, may provide uniform dose delivery and drug release kinetics (CitationDailey et al. 2003). Therefore it is of interest to understand the control of aerosol generation techniques to obtain uniform sized dry particles, generated from the evaporation of droplets, each containing a single liposome. The inclusion of liposomes or other particles in a droplet, during aerosol generation, is a purely random process, predicted by the Poisson distribution function (CitationEdd et al. 2008; CitationHogan and Biswas 2008; CitationHogan et al. 2005; CitationRaabe 1968). Techniques to achieve one liposome per droplet include increasing the number concentration of liposomes in suspension and tuning the droplet size to match the liposome in-situ diameter. A recent study (CitationEpstein et al. 2006) reported that the generation of nanometer sized dry particles, using air jet atomization, resulted from the evaporation of drops containing a single liposome. However, the distribution of liposomes in aerosolized droplets was not addressed in detail.
This article reports investigation of two methodologies (air-jet atomization and electrohydrodynamic atomization) to generate aerosols, containing nanometer-sized dry particles, from liposome suspensions. Issues addressed include the identification of conditions under which the inclusion of one-liposome-per-drop could be achieved. Electrohydrodynamic atomization (EHDA) allows control or tuning of the droplet size, through control of conductivity of the liquid used (CitationModesto-Lopez and Biswas 2010). In EDHA of liposome suspensions, a potential advantage could be the lower shear imposed on the aerosolized entity. This has been demonstrated by the use of EDHA for aerosolization of delicate biological molecules (CitationDavies et al. 2005; CitationHogan et al. 2006). The morphology and size distribution of the generated aerosols are studied to understand changes in liposome structure, which occur during aerosolization by the two techniques. The observed changes in particle structure are explained through a mechanistic approach.
2. MATERIALS AND METHODS
2.1. Preparation of Liposome Suspensions
Lipids used were 1,2-Dipalmitoyl-sn-Glycero-3-Phosphocholine (DPPC), 1,2-Dipalmitoyl-sn-Glycero-3-[Phospho-rac-(1-glycerol)] (Sodium Salt) (DPPG), and cholesterol, all purchased from Avanti Polar Lipids (USA). The composition of liposome was made of equimolar composition of DPPC, DPPG, and cholesterol. Sodium chloride was purchased from Sigma Aldrich (St. Louis, MO); potassium chloride (ACS grade, Fisher Scientific), di-sodium hydrogen orthophosphate anhydrous (Enzyme grade, Fisher Scientific), potassium di hydrogen phosphate (Enzyme grade, Fisher Scientific) were used to prepare the phosphate buffer (CitationNiven and Schreier 1990). A polycarbonate membrane, of 19 mm in diameter and 0.1 μ m porosity (Schleiger and Schuell, Dassel, Germany), was used to extrude liposome vesicles. Deionized water used was filtered through a Millipore water purification system.
The dehydration-rehydration method, which encapsulates larger mass of drug (CitationKirby and Gregoriadis 1984), was used to prepare liposomes. Liposomes were made from a lipid cake prepared by evaporating the organic solvent and hydrating with aqueous medium in a controlled temperature bath. The sizes of the resulting liposomes have multiple lipid layers and this multi-lamellarity was reduced by freeze thawing in liquid nitrogen and extruding through a 0.1 μ m polycarbonate membrane several times to prepare a monodispersed 160 nm sized liposome suspension. The liposome suspension had a lipid mass concentration of 10 mg mL–1 assuming no loss of lipid mass during preparation. A second assumption was made that the entire lipid mass forms liposomes and thus the extruded liposome number concentration in suspension was proportional to the lipid mass concentration. The liposome number concentration was calculated (CitationYamauchi et al. 2007) as 2 × 1011 units of liposome for 1 mg of liposome/mL of suspension. This was used to determine the probability of particles in a droplet generated by air jet atomization. The suspension was serially diluted with aqueous buffer or deionized water prior to aerosolization. As the electrospray facilitates control of the droplet size by manipulating conductivity, a trace amount of the volatile buffer, ammonium acetate solution (Fluka), was added to the liposome suspension made without buffer salts.
The suspension properties (hydrodynamic diameter and polydispersity index), of the extruded liposome, its conductivity and surface charge (zeta-potential) were measured using a Nano-ZS (Nanoseries, Malvern Instruments). Measurements were made at 25°C with a fixed angle of 173°. The sizes quoted are the z-average mean for the liposome hydrodynamic diameter (nm) and the calculation of the zeta-potential (mV) was done using measured electrophoretic mobility. The pH of the suspensions was measured by an Accument AB 15/15+ meter from Fisher Scientific. The image analysis of the liposome in suspension and after aerosolization was done using a JEOL JEM-2100F field emission scanning transmission electron microscope (FE-TEM). To view the morphology, liposome suspensions were drop casted on carbon-coated copper grids (400 mesh) and dehydrated in a desiccator. For better contrast, the liposomes were negatively stained with ammonium molybdate (2%, w/v). The liposomes that were collected after aerosolization on a TEM grid by impaction were not stained as the aqueous stain solution can rehydrate and modify the morphology of liposome.
2.2. Air Jet Atomization Setup
A TSI atomizer was used to aerosolize suspensions containing different concentrations of liposomes and buffer salts (). A Kr-85 neutralizer (TSI Model 3077) was used before the particles were measured with a scanning mobility particle sizer (SMPS, TSI Model 3080) using a long column (TSI Model 3081). The measured dry particles from the air jet aerosolization of 0.1% (v/v) sucrose showed that the droplets generated had a geometric mean diameter (GMD) of 770 nm and a geometric standard deviation (GSD) of 1.8.
Since the distribution of colloidal particles in droplets is considered to be Poisson distributed (CitationEdd et al. 2008; CitationHogan et al. 2006), the number of liposome particles in the selected droplet, N lipo , was obtained from the Poisson distribution function as follows
2.3. Electrospray Aerosolization System
A commercial electrospray aerosol generator (Model 3480, TSI, Inc.) was used, with capillaries of 40 μ m internal diameter (Polymicro Technologies), to generate the nanometer-sized droplets. The set up is shown in . The carrier gas flow was a mixture of 1.0 L min–1 of compressed air and 0.1 L min–1 of CO2, which prevented corona discharge at the tip of capillary. The generated droplets were then neutralized in a chamber. The aerosol characterization was done as described in section 2.2 using a scanning mobility particle sizer. To study the relationship between the shape and mobility diameter, the liposome aerosols of a specific mobility diameter were collected at the outlet of the DMA by fixing the electrode voltage and observing them by TEM.
The droplet size was manipulated by regulating the liquid flow rate and the suspension conductivity. The liquid flow rate Q (nL min–1) through the capillary was calculated from the measured capillary pressure drop by the Poiseuille equation. To estimate the droplet size, sucrose solution of 0.1% (v/v) with conductivity (k) ranging from 0.1 to 10 mS cm–1 was electrosprayed and the residual particle size distribution was measured. The following equation was used to predict the droplet diameter (D D ),
2.4. Experimental Plan
To study the stability and properties of liposome suspensions the liposome hydrodynamic diameter, zeta potential, pH and electrical conductivity were measured prior to aerosolization studies for different lipid mass concentrations (, Test #1).
TABLE 1 Experimental plan for aerosolization studies of liposome in suspensions
The lipid mass concentration used for the atomization studies was varied from 0.01 to 1 mg mL–1 (, Test #2). This range was selected as the theoretical calculations show that a significant fraction of droplets will have a high probability of having single liposome particles per droplet. The effect of atomization of the liposome suspension is established by determining the size distribution, and observed changes in the morphology of the liposome by TEM.
Test #3 is based on use of the electrospray system. The sizes of the generated droplets were controlled by adding a trace amount of ammonium acetate solution to manipulate the conductivity of the liposome suspension water in the range of 0.9 to 11.4 mS cm–1. This resulted in the generation of 130 and 200 nm sized droplets, respectively. In the presence of buffer solutes, the conductivity was 1.82 mS cm–1, and resulted in the generation of 170 nm droplets. These droplets size were investigated if they restrict the inclusion of multiple liposomes per droplet at higher lipid mass concentration (10–0.5 mg mL–1) (, Test #3). The impact of electrospray on liposome morphology was examined by TEM.
3. RESULTS AND DISCUSSION
3.1. Properties of Liposome Suspensions
The stability of the liposome suspension in terms of size and drug retention within the liposome depends on the pH of the medium (CitationSabin et al. 2006) and thus buffer was used to maintain the pH at 7.4. In the absence of the buffer, the increase in the liposome number concentration provides more surface area containing amphoteric hydroxyl groups, which lower the pH by association with more hydroxyl ions (CitationJailani et al. 2008). Since the presence of the buffer solutes leaves residuals upon evaporation and impacts the dry aerosol size distribution, studies were conducted both in the presence and in the absence of the buffer medium (, Test #1).
The hydrodynamic diameter and the zeta potential of the liposomes suspensions were measured at different lipid mass concentrations. The change in lipid mass concentration or presence of buffer salts did not affect the hydrodynamic diameters, which were 160 (± 5) nm with a polydispersity index (relative variance) below 0.1 for all suspensions. Thus, the suspensions can be considered to contain monodisperse liposome particles (CitationYamauchi et al. 2007). The zeta potential for liposome suspensions in deionized water were measured to be between –15 mV and –45 mV, depending on the lipid concentrations. In the presence of buffer, the suspensions had a high concentration of the electrolytes, which controls the zeta potential to be approximately –30 mV, with a conductivity of 1.82 (± 0.02) mS cm–1. These values were independent of the lipid mass concentrations used, in good agreement with previous studies by Modesto-Lopez and Biswas using nanoparticles (2010).
The TEM images of the liposomes before aerosolization showed that they have a spherical shape and their diameters are close to the measured hydrodynamic diameter (, panels 1 and 2). The boundary membrane showed the presence of one distinct bilayer structure which is the expected unilamellar structure for extruded liposomes (CitationYamauchi et al. 2007). The images showed that the liposomes were distorted and shrunk on drying; with distinct folded structures observed on the membranous boundary. The bilayer structure shows lattice fringes (, inset) representing the network of nanochannels, where the dimensionality refers to the underlying crystal lattice of the lipids in the bilayer of liposomes (CitationMulet et al. 2009).
FIG. 1 Schematic diagram of the experimental system to measure size distribution of residual liposome (a) atomizer and (b) electrospray. (Figure provided in color online.)
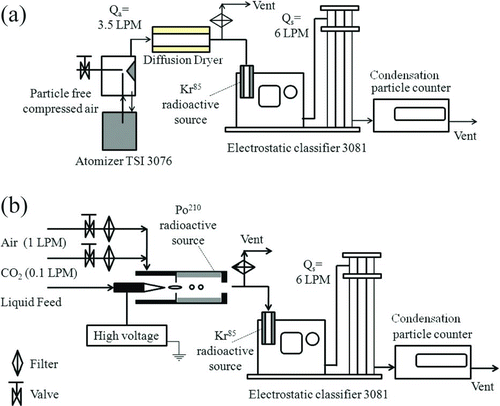
FIG. 2 HR-TEM images of liposome (1,2) before aerosolization with the crystalline arrangement of the lipid molecules in the bilayer (inset) and (3,4) air jet atomized liposome.
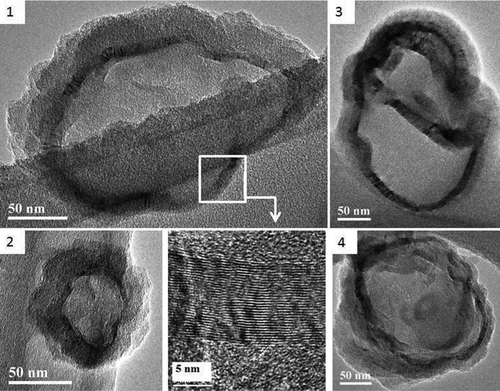
These properties of the liposome in suspension were used to design and understand the aerosolization experiments. The size and morphology of the liposomes in suspension were used for further experiments of its inclusion in generated droplets based on aerosol size distribution.
3.2. Air Jet Atomization of Nanometer Sized Liposome
The air jet atomization of liposome suspensions was studied for inclusion of one particle per droplet for generation of nanometer sized aerosols. Since, the liposomes were preserved in the buffer medium, the impact of solutes was assessed by systematically varying the lipid mass concentration and observing the dry aerosol particle size distribution (, Test #2).
3.2.1. Distribution of Liposomes in Droplets
Since larger droplets have a higher probability of containing multiple liposomes, the largest droplet size was used to calculate the number of liposomes in the droplet at the different lipid mass concentrations. The lipid concentrations were varied from 0.01 to 1 mg mL–1. The probabilities of the number of liposomes likely to get encapsulated in a 3 μ m diameter droplet are shown in at different concentrations of liposome suspensions in the presence and absence of buffer salts.
FIG. 3 Calculated fraction of droplets containing a certain number of liposomes for a 3 μ m sized droplet.
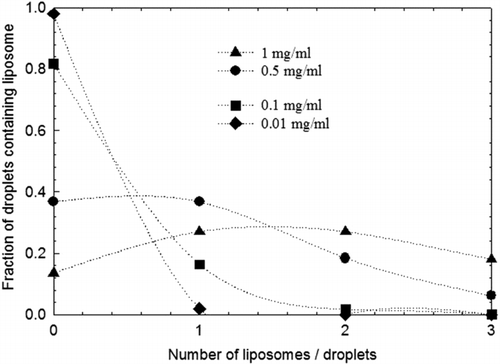
As the constant output atomizer generates a residual particle from the pure water (CitationEpstein et al. 2006), the aerosol size distribution from deionized water was considered to be the background (). The aerosol size distribution of the liposome particles was unimodal, with a geometric standard deviation (GSD) of 1.7. At a lipid mass concentration of 0.01 mg mL–1, the probability calculations show a small fraction of the atomized droplets occupied with liposome and thus, the aerosol size distribution will be independent of the presence of the liposomes. The number concentration of generated aerosols was significantly above the background level when a 0.1 mg mL–1 of lipid mass concentration suspension was atomized. The size distribution had a single mode at 94 (± 3.5) nm mobility diameter (). At 0.1 mg mL–1 lipid mass concentration, the Poisson distribution function predicts 16% of the generated droplets have one liposome, while 2% have multiple liposomes (). Since the smaller droplet sizes will have a lower probability for inclusion of multiple liposomes and these calculations were based on the largest droplet diameter, the atomization of 0.1 mg mL–1 lipid suspension would generate droplets with 98% having one or no liposomes. This finding is consistent with CitationEpstein et al. (2006) who reported a dry particle mode at 100 nm mobility diameter, when 200 nm size liposomes in suspension were aerosolized. Similarly, the Poisson distribution function predicts that atomization of 0.5 mg mL–1 lipid concentration generates 37% of the aerosol droplets with inclusion of one liposome, 23% have multiple liposomes (). Since a significant fraction of the droplets contained multiple liposomes, the resulting aerosol contained larger particle sizes with a geometric mean diameter (GMD) of ∼ 132 nm. The fraction of droplets that contain one or more liposomes increased from approx 20% to 63%, resulting in a close to tripling in the number of particles (), which explains the proportionate increase in the total aerosol number concentration by about a factor of two (). Further increase in the lipid mass concentration to 1 mg mL–1 did not significantly change the number concentration as predicted by calculations. Since, lipids act as surfactants, a high lipid mass concentration could influence the atomized droplet size distribution through surface tension effects (CitationMcCallion et al. 1996) and invalidate the assumptions made for the theoretical calculations.
TABLE 2 Geometric mean size and total number concentration for aerosolized liposome suspension by atomizer
3.2.2. Influence of the Buffer Solute
The atomization of the liposome suspension in an aqueous buffer medium showed a difference in the aerosol size distribution from liposomes in deionized water (). An additional mode at a size of 55 nm () was observed; and this mode most probably originated from droplets containing no liposomes ending up as dry residual salt particles. The Poisson distribution calculations show that at a lipid mass concentration of 0.1 mg mL–1 82% of the droplets would contain no liposome.
FIG. 4 Normalized aerosol particle number concentration on atomization of the liposome suspension in phosphate buffer.
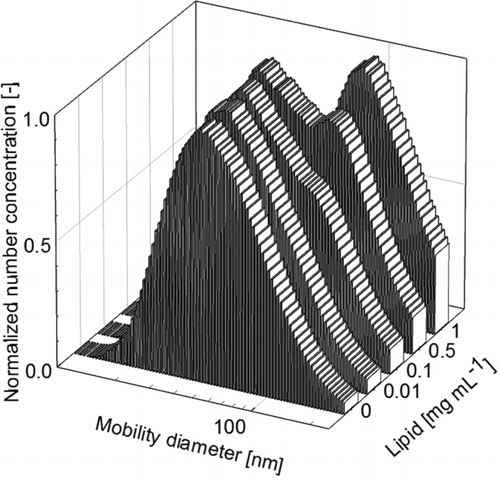
TEM images of the atomized liposomes showed that the spherical morphology of the unilamellar liposomes was preserved upon aerosolization. However, the mobility diameter of the liposome aerosol was lower than the hydrodynamic diameter, which was explained by the shrinkage in the size of the aerosolized liposome upon droplet evaporation (CitationEpstein et al. 2006) and by the distinct folded structures in the bilayer observed in the TEM images (, panels 3 and 4). , panel 3, shows that the inclusion of two liposomes in a droplet could lead to an agglomerated structure as the droplet evaporates; and this was verified by the larger mobility diameter of ∼ 132 nm measured by the SMPS (). A careful study of the agglomerated liposome images shows that the inter-lamellar attachments of the liposome were not completely fused due to absence of osmotic forces. Thus, the respective encapsulated space remained distinctly compartmentalized in the agglomerated structure. This is known in liposome agglomeration as hemifusion or the coalescence of the contacting monolayers of the membranes without mixing of the encapsulated aqueous space (CitationChanturiya et al. 1997; CitationMulet et al. 2009).
3.3. Electrospray Generated Liposome Aerosols
As atomization was not successful in generating droplets containing one liposome particle, and the influence of the large number of buffer only droplets; an electrospray technique was used to more effectively control the droplet size.
3.3.1. Electrospray at Different Initial Droplet Sizes
The lipid concentrations were changed from 0.5 to 10 mg mL–1, the ranges selected to ensure designed operation of the electrospray in the cone jet mode. At the low lipid mass concentration, a significant number of droplets were generated without liposome. These formed residual particles containing only buffer solute. Thus, at lipid mass concentration lower than 1 mg mL–1, a larger particle number concentration is measured in presence of buffer than in deionized water. This difference in aerosol count diminishes with higher lipid mass concentration from reduction in the number of droplets containing no liposome (). On comparing the suspension concentration to particle number concentration, shows that the increase in the particle number concentration is proportionally less than the increase in the lipid mass concentration. Further studies were focused on electrospray induced liposome agglomeration at the high lipid mass concentration in suspension.
TABLE 3 Dry particle number concentration of liposomes for different size electrosprayed droplets and mass concentration of lipids
When buffer solution (lipid concentration 0 mg mL–1) was electrosprayed, the generated aerosols were monodispersed with a GMD of 14.5 nm and a GSD of 1.14 (). The presence of the liposome in the dilute suspensions generates two peaks in addition to the buffer salt peak generated from the droplets without liposomes (). The bimodal aerosol size distributions from the liposome in suspension had peak mobility diameters of 35 and 100 nm. The number concentration of these two modes was observed to increase with raising the lipid mass concentration because of an increase in the number of liposomes in the suspension.
FIG. 5 Normalized aerosol particle number concentration on electrospraying the liposome suspension in phosphate buffer (droplet size is 170 nm).
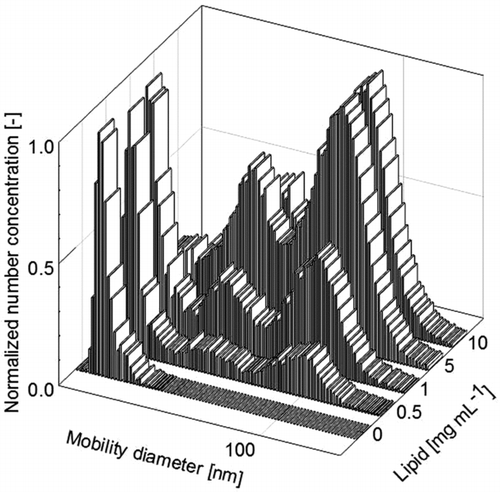
To estimate the effect of different droplet size and micro capillary diameter of electrospray, the aerosol size distribution at different lipid mass concentrations were compared at three different conductivities (). The diameter of the micro capillary, which was calculated (CitationGaòan-Calvo 1994) to be in the range of 75 to 150 nm, scaled proportionately with droplet diameter. Thus, the constricted path could induce morphological changes and agglomeration. At a lipid mass concentration of 5 mg mL–1, the aerosol size distribution remains statistically indistinguishable at the three different initial droplet sizes (). At a lipid mass concentration of 5 mg mL–1, the salt peak becomes insignificant as all droplets are occupied by liposomes, thereby decreasing the effect of soluble buffer salt on the aerosol particle size distribution. The bimodal distribution of the resultant liposome aerosol was therefore related to agglomeration induced in the flow path.
3.3.2. Morphology and Mobility Diameter of Electrosprayed Liposomes
The shape of the particles impact the measured mobility diameter (CitationZelenyuk and Imre 2007). To determine the shape of the particles at the two observed peaks in the mobility distribution, they were collected for TEM imaging. The existing understanding on the difference in physical (TEM) and mobility diameters (SMPS) could be due to the charge distribution (CitationKim et al. 2005) or different orientation in DMA based on particle morphology (CitationZelenyuk and Imre 2007), which does not seems to explain the present observations of electrosprayed liposomes. However, for freely tumbling cylindrical structures like carbon nanotubes, it had been demonstrated that the drag force is proportional to its smaller diameter (width) of the cylindrical structure when the aspect ratio of the spheroid id below 8 (CitationKim et al. 2007).
The TEM images showed liposomes as cylindrical objects. Over 15 different liposome aerosols were analyzed to understand the extent of variation in the length of the cylinders (). Liposome particles with a 100 nm mobility diameter had a length of 587 (± 273) nm and the width of 178 (± 71) nm in TEM images. Similarly, liposomes with 35 nm mobility diameter were observed to have a length of 641 (± 445) nm and the width of 110 (± 35) nm. The average width of the liposome is greater for particles classified to be 100 nm than 35 nm. Thus, soft colloids like liposomes tend to acquire the cylindrical shape of the liquid capillary at the tip of the electrospray. Since the diameter of the liquid capillary is smaller than the in-situ diameter of the liposomes, the movement of these colloidal structures in the liquid cylinder could be restricted, leading to the observed liposome agglomeration.
FIG. 7 The cartoon (top) and TEM images (bottom) of single and paired entry of liposome in the electrosprayed cone jet, which causes head to tail and side by side agglomeration of liposome leading to 100 nm (left) and 35 nm (right) mobility diameter.
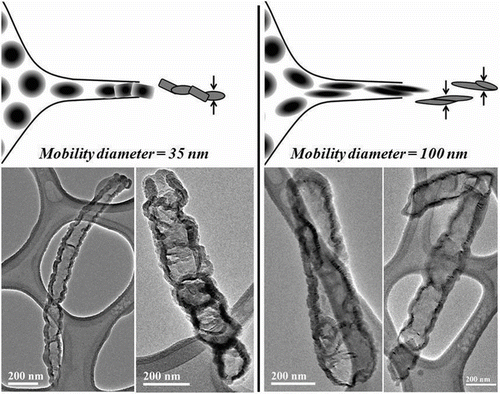
The TEM images suggest that there exist two ways for the liposomes to enter the Taylor cone geometry and agglomerate within the length of the liquid cylindrical structure. A sizable fraction of liposomes get hemifused, along their cross section area to form a long cylindrical structure. A second way of agglomeration, leading to the observed hemifusion through the length of the deformed liposome structure, might occur as two liposomes are forced through the electrospray capillary simultaneously (). These two different ways of agglomeration of cylindrical protein structures have been proposed for human carbonic anhydraze II. The agglomeration was induced by high concentrations of colloidal particles where the inter-particle distance was reduced. The dimension of the smaller axis suggests a head-to-tail assembly, and the larger diameters suggest a side by side juxtaposition of two primary cylindrical structures (CitationCeolin et al. 2001). The mean length suggest the agglomeration of four to five liposomes into a long cylindrical structure ().
Since quantitative studies on inclusion of one liposome per droplet are not yet available, the current work shows that such objectives could be possible by manipulating the properties of liposome suspensions and choice of aerosolization technique. shows a comparison of the two aerosol generation techniques used to generate dry aerosols for obtaining one particle per droplet. The dry particle size distribution of electrosprayed liposome with lipid mass concentration of 5 mg mL–1 is compared with the atomized liposome suspension with lipid mass concentration of 0.1 mg mL–1; made in a buffer medium and in deionized water. The buffer salt had a significant effect in the atomization system, as the dry particle size distributions were very different in presence of the buffer salt (). This indicates that a sizable fraction of generated droplets remained without liposome. The electrospray of the liposome suspensions at a lipid mass concentration of 5 mg mL–1 were observed to have the same size distribution independent of the buffer salts. Although electrospraying induces morphological changes and agglomeration in soft colloids like liposomes, a smaller mobility diameter and the control on liposome inclusion in the droplets could be of advantageous for encapsulated drug delivery to the alveolar region. Further studies are necessary to study these effects of agglomeration on the encapsulation of drugs.
4. CONCLUSIONS
Studies were conducted on two different aerosolization methods, atomization and electrohydrodynamic atomization, for nanoparticle aerosol generation from 160 nm extruded liposome suspensions. Atomization generated micrometer size droplets, which had multiple units of liposomes at high suspension concentrations. Atomization of lower concentration suspensions (0.1 mg mL–1 lipids) could generate aerosols comprising of a single liposome. However, at this liposome concentration only 15% of the droplets had liposomes, with the remaining fraction only resulting in the formation of buffer salt particles. Electrohydrodynamic atomization was used to more effectively control the droplet size, and reduce the fraction containing no liposome particles. This enables the aerosolization of high lipid mass concentration (5 mg mL–1) suspensions at which the effect of solutes (buffer salts) was minimized. The electrosprayed liposomes had a bimodal size distribution with peaks at 35 and 100 nm, respectively. The bimodality was due to two different agglomeration pathways resulting in cylindrical structures with two different diameters. The liposomes can agglomerate by head-to-tail assembly, and by a side-by-side juxtaposition of two primary cylindrical structures. The measured mobility diameters corresponded to the diameter of the two cylindrical structures that were aligned in the DMA. These results have important implications on aerosolization technique for generating nanometer aerosol from liposome suspension.
This work was supported in part by grants from Indo-US Joint Center for Nanoparticle Aerosol Science and Technology (NAST) funded by the Indo-US Science and Technology Forum (IUSSTF), New Delhi, India and grants from the Aerosol and Air Quality Research Laboratory (AAQRL) at Washington University in St. Louis. We also acknowledge partial support by the McDonnell Academy Global Energy and Environmental Partnership (MAGEEP). The authors thank Manoranjan Sahu, doctoral student (Washington University in St. Louis) for helpful discussions.
REFERENCES
- Bailey , M. M. and Berkland , C. J. 2009 . Nanoparticle Formulations in Pulmonary Drug Delivery . Med. Res. Rev. , 29 : 196 – 212 .
- Balásházy , I. , Németh , I. , Alföldy , B. , Szabó , P. P. , Hegedus , C. S. , Hofmann , W. , Pálfalvi , J. , Fehér , I. and Török , S. 2000 . Aerosol Deposition Modeling in Human Airways and Alveoli . J. Aerosol Sci. , 31 : 482 – 483 .
- Ceolin , M. , Colombo , U. S. , Frate , M. C. , Clerico , E. , Anton , E. and Ermacora , M. R. 2001 . Head-to-Tail and Side-by-Side Oligomerization of Human Carbonic Anhydrase II: A Small Angle X-Ray Scattering Study . Inter. J. Biol. Macromol. , 28 : 143 – 150 .
- Chanturiya , A. , Chernomordik , L. V. and Zimmerberg , J. 1997 . Flickering Fusion Pores Comparable with Initial Exocytotic Pores Occur in Protein-Free Phospholipid Bilayers . Proc. Natl Acad. Sci. USA. , 94
- Chattopadhyay , P. , Shekunov , B. Y. , Yim , D. , Cipolla , D. , Boyd , B. and Farr , S. 2007 . Production of Solid Lipid Nanoparticle Suspensions Using Supercritical Fluid Extraction of Emulsions (SFEE) for Pulmonary Delivery Using the AERx System . Adv. Drug Deliv. Rev. , 59 : 444 – 453 .
- Chen , D. R. and Pui , D. Y. H. 1997 . Experimental Investigation of Scaling Laws for Electrospraying: Dielectric Constant Effect . Aerosol Sci. Technol. , 27 : 367 – 380 .
- Dailey , L. A. , Schmehl , T. , Gessler , T. , Wittmar , M. , Grimminger , F. , Seeger , W. and Kissel , T. 2003 . Nebulization of Biodegradable Nanoparticles: Impact of Nebulizer Technology and Nanoparticle Characteristics on Aerosol Features . J. Controlled Release. , 86 : 131 – 144 .
- Dandekar , P. , Venkataraman , C. and Mehra , A. 2009 . Pulmonary Targeting of Nanoparticles Drug Matrices . J. Aerosol Med. , Submitted
- Davies , L. A. , Hannavy , K. , Davies , N. , Pirrie , A. , Coffee , R. A. , Hyde , S. C. and Gill , D. R. 2005 . Electrohydrodynamic Comminution: A Novel Technique for the Aerosolisation of Plasmid DNA . Pharma. Res. , 22 : 1294 – 1304 .
- Desai , T. R. , Hancock , R. E. W. and Finlay , W. H. 2003 . Delivery of Liposomes in Dry Powder Form: Aerodynamic Dispersion Properties . Euro. J. Pharma. Sci. , 20 : 459 – 467 .
- Edd , J. F. , Di Carlo , D. , Humphry , K. J. , Koster , S. , Irimia , D. , Weitz , D. A. and Toner , M. 2008 . Controlled Encapsulation of Single-Cells into Monodisperse Picolitre Drops . Lab on a Chip. , 8 : 1262 – 1264 .
- Epstein , H. , Afergan , E. , Moise , T. , Richter , Y. , Rudich , Y. and Golomb , G. 2006 . Number-Concentration of Nanoparticles in Liposomal and Polymeric Multiparticulate Preparations: Empirical and Calculation Methods . Biomaterials. , 27 : 651 – 659 .
- Fu , J. , Fiegel , J. , Krauland , E. and Hanes , J. 2002 . New Polymeric Carriers for Controlled Drug Delivery Following Inhalation or Injection . Biomaterials. , 23 : 4425 – 4433 .
- GaÒan-Calvo , A. M. 1994 . The Size and Charge of Droplets in the Electrospraying of Polar Liquids in Cone Jet Mode and the Minimum Droplet Size . J. Aerosol Sci. , 25 : S309 – S310 .
- Giovanella , B. C. , Knight , J. V. , Waldrep , J. C. , Koshkina , N. , Gilbert , B. and Wellen , C. W. 2008 . Small Particle Liposome Aerosols for Delivery of Anti-Cancer Drugs US Patent 7,341,739. ed., Research Development Foundation, Carson City, NV (US), United States
- Hofmann , W. and Koblinger , L. 1990 . Monte-Carlo Modeling of Aerosol Deposition in Human Lungs: Deposition Fractions and Their Sensitivity to Parameter Variations . J. Aerosol Sci. , 21 : 675 – 688 .
- Hogan , C. J. and Biswas , P. 2008 . Monte Carlo Simulation of Macromolecular Ionization by Nanoelectrospray . J. Amer. Soc. Mass Spectrom. , 19 : 1098 – 1107 .
- Hogan , C. J. , Kettleson , E. M. , Lee , M. H. , Ramaswami , B. , Angenent , L. T. and Biswas , P. 2005 . Sampling Methodologies and Dosage Assessment Techniques for Submicrometre and Ultrafine Virus Aerosol Particles . J. Appl. Microbiol. , 99 : 1422 – 1434 .
- Hogan , C. J. , Kettleson , E. M. , Ramaswami , B. , Chen , D. R. and Biswas , P. 2006 . Charge Reduced Electrospray Size Spectrometry of Mega- and Gigadalton Complexes: Whole Viruses and Virus Fragments . Anal. Chem. , 78 : 844 – 852 .
- Huang , Y. Y. and Wang , C. H. 2006 . Pulmonary Delivery of Insulin by Liposomal Carriers . J. Control. Release. , 113 : 9 – 14 .
- Jailani , S. , Franks , G. V. and Healy , T. W. 2008 . ZetaPpotential of nanoparticle Suspensions: Effect of Electrolyte Concentration, Particle Size, and Volume Fraction . J. Amer. Ceramic Soc. , 91 : 1141 – 1147 .
- Kim , S. H. , Mulholland , G. W. and Zachariaha , M. R. 2007 . Understanding Ion-Mobility and Transport Properties of Aerosol Nanowires . Aerosol Sci. , 38
- Kim , S. H. , Woo , K. S. , Liu , B. Y. H. and Zachariah , M. R. 2005 . Method of Measuring Charge Distribution of Nanosized Aerosols . J. Colloid and Interface Sci. , 282 : 46
- Kirby , C. and Gregoriadis , G. 1984 . Dehydration-Rehydration Vesicles: A Simple Method for High-Yield Drug Entrapment in Liposomes . Bio-Technology. , 2 : 979 – 984 .
- Kleemann , E. , Schmehl , T. , Gessler , T. , Bakowsky , U. , Kissel , T. and Seeger , W. 2007 . Iloprost-Containing Liposomes for Aerosol Application in Pulmonary Arterial Hypertension: Formulation Aspects and Stability . Pharma. Res. , 24 : 277 – 287 .
- McCallion , O. N. M. , Taylor , K. M. G. , Thomas , M. and Taylor , A. J. 1996 . The influence of Surface Tension on Aerosols Produced by Medical Nebulisers . Intl J. Pharma. , 129 : 123 – 136 .
- Modesto-Lopez , L. B. and Biswas , P. 2010 . Role of the Effective Electrical Conductivity of Nanosuspensions in the Generation of TiO2 Agglomerates with Electrospray . J. Aerosol Sci , 41 : 790 – 804 .
- Mulet , X. , Gong , X. , Waddington , L. J. and Drummond , C. J. 2009 . Observing Self-Assembled Lipid Nanoparticles Building Order and Complexity through Low-Energy Transformation Processes . ACS Nano. , 3 : 2789 – 2797 .
- Niven , R. W. and Schreier , H. 1990 . Nebulization of Liposomes: Effects of Lipid-Composition . Pharma. Res. , 7 : 1127 – 1133 .
- Raabe , O. G. 1968 . The Dilution of Monodisperse Suspensions for Aerosolization . American Indust. Hyg. Assoc. J. , 29 : 439 – 443 .
- Rogueda , P. G. A. and Traini , D. 2007 . The Nanoscale in Pulmonary Delivery. Part 1: Deposition, Fate, Toxicology, and Effects . Expert Opin. Drug Deliv. , 4 : 595 – 606 .
- Sabin , J. , Prieto , G. , Ruso , J. M. , Hidalgo-Alvarez , R. and Sarmiento , F. 2006 . Size and Stability of Liposomes: A Possible Role of Hydration and Osmotic Forces . Euro. Physical J. E. , 20 : 401 – 408 .
- Ten , R. M. , Anderson , P. M. , Zein , N. N. , Temesgen , Z. , Clawson , M. L. and Weiss , W. 2002 . Interleukin-2 Liposomes for Primary Immune Deficiency Using the Aerosol Route . Intl. Immunopharmacol. , 2 : 333 – 344 .
- Triplett-II , M. D. 2004 . “ Enabling Solid Lipid Nanoparticles Drug Delivery Technology by Investigating Improved Production Techniques ” . In Chemical Engineering , 172 Columbus : The Ohio State University .
- Wong , J. P. , Yang , H. M. , Blasetti , K. L. , Schnell , G. , Conley , J. and Schofield , L. N. 2003 . Liposome Delivery of Ciprofloxacin Against Intracellular Francisella Tularensis Infection . J. Controlled Release. , 92 : 265 – 273 .
- Yamauchi , M. , Tsutsumi , K. , Abe , M. , Uosaki , Y. , Nakakura , M. and Aoki , N. 2007 . Release of Drugs from Liposomes Varies with Particle Size . Biological & Pharmaceutical Bulletin. , 30 : 963 – 966 .
- Zelenyuk , A. and Imre , D. 2007 . On the Effect of Particle Alignment in the DMA . Aerosol Sci. Technol. , 41 : 112 – 124 .