Abstract
For effective sampling of airborne micro-organisms different techniques such as impingement, filtration and impaction are commonly used. Impaction is the method which allows best for direct collection on adhesive surfaces for immediate microscopic analysis. The efficiency of collection largely depends on the quality of the sampling surface to retain particles. This article compares the suitability of some traditional and new adhesive coatings to collect and retain airborne micro-organisms efficiently for subsequent fluorescence microscopy. Sacharomyces cerevisiae yeast cells were aerosolized in a test tubing system in the laboratory. Samples were collected by a round jet impactor in a defined round deposition area on glass slides coated with different adhesive materials. Particle distribution in the deposition area and collection efficiency as well as particle losses were estimated. Fluorescent staining with Mycoval and DAPI was used to quantify the amount of collected cells and cell losses due to the staining procedure. Filtration was taken as reference system. The collection efficiency of the tested adhesive surfaces ranged from 2 to 97%. Silicone sealants showed with 97% the highest collection efficiency. All particles were located in the central region of the deposition area. Cell losses due to the staining procedure were very small (<1%) and no background fluorescence was observed after DAPI staining. Even after sampling times of 45 min the collection efficiency was still above 90%. Longer collection (>50 min) resulted in increasing cell losses. Collection efficiency was little influenced by relative humidity of the air between 20% and 100%.
1. Introduction
Monitoring air quality including airborne dust and micro-organisms gained increasing importance during the last decades since knowledge grew that airborne particles seem to be associated with severe respiratory disorders in animal and man (CitationAndersen et al. 2004; CitationDonham et al. 2000; CitationJekel et al. 2001; CitationRadon et al. 2001). Consequently, there was a high demand for reliable, efficient, and fast methods to sample, quantify and identify airborne dust and micro-organisms.
Most current sampling techniques for airborne micro-organisms use filtration, impingement, or impaction methods (CitationGrinshpun et al. 2007). Filters can collect particles of different diameters very efficiently when the appropriate pore sizes are used but with increasing sampling time bacteria can be inactivated by desiccation according to their environmental resistance. Impingement in liquids avoids desiccation but the sampled micro-organisms may be stressed by the violent dispersion in the sampling liquid. Impaction collects micro-organisms directly on soft or hard surfaces like agar for incubation and colony growing or on adhesive or non-coated glass slides for further microscopic analysis.
In the last decades increasingly not culture-based techniques are used like epi-fluorescent microscopy (CitationMoschandreas et al. 1996; CitationTong and Lighthart 1999; CitationTerzieva et al. 1996; CitationChi and Li 2007; CitationChi and Li 2005) or fluorescent in situ hybridization (FISH) (CitationChi and Li 2005, Citation2006) in order to identify the collected micro-organisms qualitatively and quantitatively immediately at the place of sampling within minutes or a few hours. These methods are suitable to deliver the total microbial cell concentration in an air sample, they can detect how many micro-organisms are still viable without growth (CitationChi and Li 2005) and they can also determine the presence of non-biological particles (CitationParkes and Taylor 1985). For such microscopic investigations the airborne micro-organisms are usually sampled by direct impaction on glass slides or similar plain surfaces. Examples of commercially available samplers are the seven stage Ultimate Cascade Impactor (CitationMay 1975), the AeroTrapTM Total Fungal Sampler, Brandt Instruments Inc. USA, the MicroBiological Air Sampling System MBASS30, Umweltanalytik Holbach GmbH, Germany, or the Portable Aerosol Spectrometer 1.209, Grimm Aerosol Technik GmbH, Germany.
However, the direct impaction of airborne micro-organisms on plain surfaces encounters several limitations. Most important are the so called bounce-off and blow-off effects (CitationMarple et al. 1993) caused by the air velocity of the impacting air stream. CitationRao and Whitby (1978) reported that the collection efficiency of particle sampling on uncoated impaction surfaces reaches a maximum of 30–50% only. The impact on the dry surface can damage the viability of the micro-organisms either by physical force or by drying (CitationHatch and Wolochow 1969) or by electrostatic charges (CitationHorton et al. 1992). Another general problem is overloading when too many particles are deposited on one surface carrier (CitationChang et al. 1994).
In order to improve the retention of the aerosol particles and increase collection efficiency numerous types of greases and oils like Cedar oil or Vaseline (CitationMay 1945; CitationRao and Whitby 1978) have been tested as adhesive coatings with varying success such as 10% Vaseline solution in n-hexane (Comtois and Mandrioli 1999), Glycerin jelly (CitationDavies et al. 1951; CitationAdhikari et al. 2004), viscous oils and sticky tapes, Dow-Corning Hi-Vac silicone grease (CitationLundgren 1967; CitationStern et al. 1962), 10% Dow-Corning Hi-Vac silicone grease solution in n-hexane (CitationMitchell et al. 1988), 3M Kel-F polymer wax (CitationLundgren 1967), Glycerine/gelatine (12,5%), Silicone fluid/carbon tetrachloride (2%), Paraffin (Comtois and Mandrioli 1999), Silicone resin SR-516 diluted in toluene to 20–30% (CitationHogan 1970), or Dow Corning silicone oil (in 1% Hexane) (CitationRao and Whitby 1978). Some materials were found to be not stable enough, others were easily soluble in water or deteriorated quickly after sampling allowing not enough time for assessment and evaluation of the impacted particles. Ideal adhesives should be mass stable, insoluble in water, tacky even after exposure to water and drying, adherent to both natural and artificial particles, transparent in coating, easy to handle, and non-toxic. Crucially important is further that the surface does not react with fluorescent dyes avoiding background fluorescence which would make cell count and following analysis more difficult or even impossible. In recent years some new materials appeared including pressure sensitive adhesives, special silicone sealants, and glass slides with commercially prepared adhesive coatings. However, most of these surfaces were never used for bio-aerosol sampling before.
This article compares the retention efficiency of 29 well-known adhesive coatings including some new materials for aerosolized yeast cells in a laboratory test tubing system followed by direct fluorescence microscopic analysis. Two different filtration methods are used as reference systems.
2. Materials and Methods
2.1. Preparation of Test Aerosols
Saccharomyces cerevisia was chosen as test organism for our aerosol experiments because the yeast cells are nonpathogenic and easy to lable with the nontoxic fluorescent stain Mycoval. Cells were denaturated in 50% Ethanol (p. a.) for 30 min, centrifuged at 2000 g for 1 min, re-suspended in a 5% Mycoval solution (Helmut Hund GmbH, Wetzlar) and stained for 30 min. The stained cells were washed 3 times in ultra-pure water and counted in a Thoma chamber. Appropriate dilutions were prepared for the experiments with concentrations ranging between 350,000 and 3,500,000 cells/mL test aerosol suspension.
2.2. Experimental Setup for the Aerosol Measurements
The test aerosols were sprayed in the air stream of a laboratory-based aerosol test tubing system constructed of 125 mm Ø KGB PVC pipes (Ostendorf Kunststoffe GmbH and Co. KG, Vechta) and stainless steel boxes with a total air volume of 0.14 m2 (). Ambient air is sucked through the inlet into a humidity controller where the air can be conditioned in a range between 20% and 100% relative humidity according to experimental requirements. It contains two exchangeable cartridges. One cartridge is equipped with an ultrasonic nebulizer with five membranes (Alpine Super Power 5-Jet Pond Fogger), which was used with ultra-pure water with very low particle concentrations to increase relative humidity. The other is equipped with a particulate adsorbent KC-Trockenperlen Orange™ to decrease relative humidity (Engelhardt Process Chemicals GmbH, Nienburg). Before the aerosol is injected the humidity conditioned air passes a VP 10 filter (AAE VariPak®) to remove airborne impurities. For the injection of the test aerosol a “PariTurboBoy”-medical nebulizer (Pari GmbH, Starnberg) was used, operating at an air flow rate of 5 L/min, aerolizing 1 mL particle suspension in 3 min. The capacity of the nebulizer was 10 mL which was sufficient for a continuous spraying and sampling period of 30 min. Continuous monitoring with the Bioaerosol Spectrometer 1.209 showed that the aerosol generation was relatively stable (+/– 10%) in the first 20 min. In the last 10 min the number of aerolized particles rose steadily up to 120% compared to the number of particles at start (100%). Calculations showed that on the average approximately 45% of the cells got lost in the test system between injection and the sampling probe. This was calculated by the ratio of the concentration of cells which were nebulized (calculated from the counted cell number per mL and the time period of vaporizing) and the cell concentration which was counted on the reference filter.
The air enriched with the yeast cell aerosol flows through a stainless steel box (1000 mm × 300 mm × 300 mm) equipped with an ozone generator which is used for disinfection purposes after experiments. A laminar air flow is established by a baffle plate behind the box and before the air reaches the sampling and measuring section to allow isokinetic sampling. A second baffle plate marks the end of the measuring section. The air finally passes a stainless steel box where the aerosol can be inactivated by UV-radiation. This box houses also the fan (VENT-125L, Soler and Palau GmbH, Darmstadt) which provides the under-pressure in the tubing system and a constant air speed through the test system which can be adjusted between 0 and 3.0 m/s. Orientating measurements showed that a constant air velocity of 1.35 m/s was high enough to avoid losses of yeast cells by sedimentation and low enough to prevent their adhesion to the walls of the test system. The air leaving this box can either be discharged through an air exhaust pipe or can be fed back into the test tubing system. By aid of the ozone generator and the UV-lamps the tubing system can be disinfected. For this purpose air is re-circulated for about 30 min in the system with both instruments activated. This is particularly important after experiments when test aerosols containing pathogenic micro-organisms are used.
All samples were taken at a room temperature of 22°C +/–2°C. Ozone (OGW 2000, DiverseyLever, NL), flow rate (hot wire anemometer V1, Testo AG, Lenzkirchen), temperature, and relative humidity (Hydrolog with a Hydroclips-S sensor module, Rotronic GmbH, Ettlingen) were continuously measured during the experiments. Yeast cells were collected from the air-stream in the tube by an isokinetic sampling probe (Grimm Aerosol Technik GmbH and Co. KG, Ainring). This airstream was alternating either directed to a personal sampler which was used as reference system or a Portable Bio-aerosol Spectrometer 1.209 (Grimm Aerosol Technik GmbH and Co. KG, Ainring).
The Portable Bio-aerosol Spectrometer 1.209 which provides continuous particle detection in the size range of 0.25 μm to 32 μm in real time is equipped with a round jet impactor which allows collection of micro-organisms and particles from an air-stream on a microscopic glass slide forming round deposition spots. Such glass slides were prepared with different adhesive coatings in these experiments (explained below) with the aim to sample as much particles as possible in the deposition spot. Particle distribution in the deposition spot, collection efficiency, surface quality of the coating as well as particle losses and background fluorescence after fluorescent staining with DAPI were analyzed. All adhesives were tested 5 times (n = 5).
2.3. Impactor Characteristics
The sample volume of the Portable Bio-aerosol Spectrometer was fixed to 1.2 L/min by a built-in flow controller in the pump. The flow rate (Q) in the attached impactor () is 1.6 L/min which is composed of 1.2 L/min sample air containing the particles and an additional stream (0.4 L/min) of particle free rinse air. This clean air is branched off from the main air stream behind the impactor, passes a filter and rinses the optical components of the spectrometer.
The impaction velocity (vp) of the particles at the outlet of the impactor nozzle was 41.94 m/s (calculated from the flow rate and the diameter of the impactor nozzle). This velocity was close to the ideal sampling velocity for bio-aerosol of 40 m/s reported by CitationStewart et al. (1995). For the impactor jet diameter (Dj ) of 0.9 mm the cut-off point diameter (d 50) of the impactor is 0.8475 μm, calculated according to Equation (Equation1) with a viscosity (η) of air of 1.81 10–5 Pa·s (at 20°C), a standard particle density (ρ p ) of 1.0 g/cm3, and a Stokes number (Stk 50) of 0.24 for round jet impactors.
2.4. Reference Systems
As reference system for the collection of airborne particles a BGIA certificated (BGIA 2001) personal sampler GSP 3.5 (GSA Messgerätebau GmbH Neuss) equipped with 37 mm cellulose nitrate filter, pore size 0.45 μm (Sartorius AG, Göttingen) was used. It works with a sampling flow rate of 3.5 L/min. The flow rate was controlled with a Mass Flow Controller GFC 0–15 L/min (Analyt-MTC GmbH, Müllheim). The number of yeast cells sampled from air and counted on the filter surface by fluorescence microscopy was taken as 100%. The relatively large diameter of the filter requires a considerable time for evaluation. Therefore a polysulfon filter holder (Roth GmbH and Co. KG, Karlsruhe) equipped with a much smaller cellulose acetate filter (13 mm), pore size 0.45 μm (Sartorius AG, Göttingen) was tested as an alternative.
2.5. Adhesive Surfaces and Preparations
In order to look for the most effective sampling procedure commercially available coated and uncoated glass slides as well as own preparations were tested on sampling efficiency for yeast cells ().
TABLE 1 Adhesive surfaces and prepared coatings
2.6. Analysis of Particle Distribution and Collection Efficiency
After sampling, the yeast cells collected on the different adherent surfaces were stained with 4′.6-Diamidino-2- phenylindole-dihydrochlorid (DAPI) with a concentration of 30 μg/mL and counted under a fluorescence microscope H600/12 (Helmut Hund GmbH, Wetzlar) equipped with filter setups for Mycoval and DAPI. Microscopic fluorescent images were taken with a TK-C1481BEG color video camera (JVC® Deutschland GmbH, Friedberg). Under the microscope at a magnitude of 100-fold only an area of 1030 μm × 690 μm could be photographed with one picture. Thus, the single digital images were composed with image processing software (CorelDraw® 10, Corel USA) to display the whole impaction area with a diameter of 8 mm. The number of deposited cells on every field of a grid with 200 μm × 200 μm screen width and 8 mm edge length were counted. It was distinguished between the number of yeast cells in the central deposition spot with 1 mm diameter and the number of cells in the outer deposition area. Particles deposited on both filters were counted directly under the microscope.
The particle collection efficiency in percent (C eff) of the different surfaces was compared to the reference filter system according to Equation (Equation5). The number of yeast cells counted on the different surfaces (n(surface)) was divided by the impactor flowrate (Q(Impactor)= 1.2 L/min) and multiplied by the flowrate through the filters (Q(Filter)= 3.5 L/min) divided by the cells counted on the filter, multiplied by 100%.
2.7. Results
shows the total deposition area of 8 mm diameter on an uncoated impaction glass slide collected with the Portable Bioaerosol Spectrometer. A total of 1298 cells was counted, which represents only approximately 21% of the Saccharomyces cerevisiae cells sampled with the standard filtration method. The distribution of the particles in the sampling spot was not uniform. The majority of cells were deposited in a 5 mm wide ring-like area around the central point of the spot where only a few cells were observed. It seems that the particles were blown away from the near center area by the impacting air stream.
FIG. 3 Distribution of Saccharomyces cerevisiae cells deposited in the sampling spot on an uncoated glass slide after collection with a Portable Bioaerosol Spectrometer. The grey scale from 1 to >10 represents the number of deposited particles per 200 × 200 μm square. The total particle number is 1298.
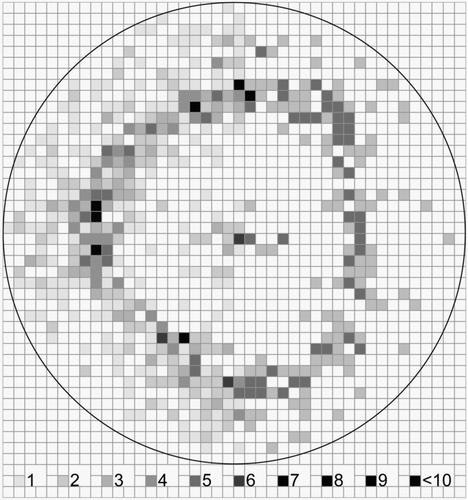
shows the distribution of Saccharomyces cerevisiae cells after impaction on an adhesive tape. The particle number was 2844 representing a sampling efficiency of approximately 45%. Clearly more particles were located in the centre of the deposition spot compared to the uncoated glass slide. The ring-like area around the center with more than 50% of the deposited particles also existed.
FIG. 4 Distribution of Saccharomyces cerevisiae cells deposited in the sampling spot on an adhesive tape (Tesa®) after collection with a Portable Bioaerosol Spectrometer. The grey scale from 1 to >10 represents the number of deposited particles per 200 × 200 μm square. The total particle number is 2844.
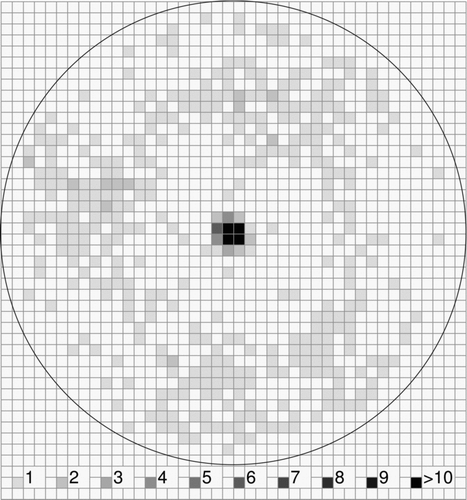
shows the distribution of Saccharomyces cerevisiae cells after impaction on a glass slide coated with a silicone sealant. 5996 cells were counted which gave a collection efficiency of about 95%. All cells without any exception as shown by microscopic analysis were deposited in the central spot with a diameter of approximately 1 mm.
FIG. 5 Distribution of Saccharomyces cerevisiae cells deposited in the sampling spot on a silicone sealant (“Sista” Küchensilikon) after collection with a Portable Bioaerosol Spectrometer. The grey scale from 1 to >10 represents the number of deposited particles per 200 × 200 μm square. The total particle number is 5996.
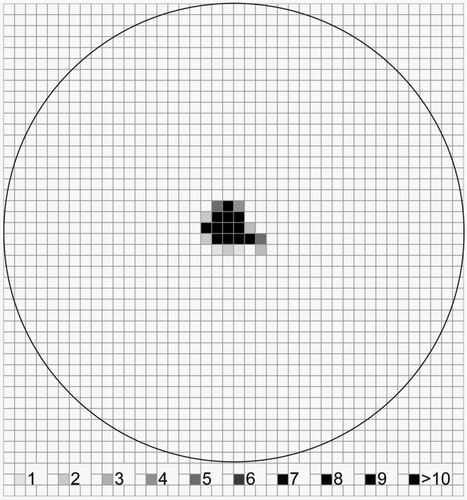
compares the sampling efficiencies for bio-aerosols of 29 different collection surfaces and the two filtration techniques used as reference systems (GSP 3.5, Polysulfon filter holder). The GSP 3.5 displayed the highest retention rates and was therefore taken as reference (100%). DAPI staining could not be used on both filters because of strong background fluorescence.
FIG. 6 Particle (yeast cells) deposition efficiency (collected with a Portable Bioaerosol Spectrometer) in the central deposition spot (d = 1 mm, dark grey bars) and outer area (d = 8 mm, light grey bars) of the impaction zone and retained particles in the whole deposition spot following DAPI staining (black bars) for different adherent surfaces compared to filtration. Each bar represents the mean results of five independent experiments.
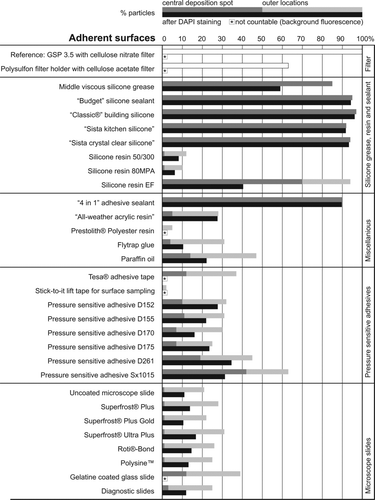
A distinction is made between cells deposited in the central deposition spot on the adherent surfaces and cells deposited in the outer area. Cell losses after staining with DAPI are indicated. DAPI staining could not be used on both filters because of strong background fluorescence. This was also true for the polyester resin, the adhesive tapes and the gelatine glass slide. Highest retention rates (up to 97%) and virtually no losses by staining were seen with different adhesive silicone sealants. The cells were nicely deposited in the central spot (), easy to count and no background fluorescence was observed.
Second best sampling results were obtained by the “4 in 1” adhesive sealant which showed a collection efficiency of 90% and negligible cell losses after staining. Middle viscous silicone grease was found with a collection efficiency of 85%, but after staining only 58% of the cells could be recovered. A part of the cells seems to be washed away by the staining procedure. Similarly, silicone resin EF had 95% collection efficiency, but more than 20% of the cells were located in the outer area and about 50% of the cells were lost by staining. The three silicone resins showed low collection efficiencies of only 12% (Silicone resin 50/300) and 10% (80MPA). Most of the cells were located in the outer area and there were high cell losses after DAPI staining. “All-weather acrylic resin” had relatively low cell losses after staining but low collection efficiency (28%) and high deposition in the outer area of the impaction zone. All other tested coating or adhesive materials such as “Prestolith®” polyester resin (5% collection efficiency), water resistant flytrap glue (30%), and paraffin oil (about 50%) revealed distinctly lower sampling efficiencies than the silicone sealants and only a few particles were located in the central deposition spot. This hold also true for the eight pressure sensitive adhesives and the “Tesa®” adhesive tape. The lowest sampling efficiency was achieved with the “stick-to-it” lift tape (<3%). Among the commercially available glass slides none of the products (Superfrost® Plus, Superfrost® Plus Gold, Superfrost® Ultra Plus, Polysine™, Diagnostic Slides, Roti® -Bond) reached a satisfying results. Collection efficiencies ranged between 21 and 31%. Most particles were deposited in the outer zone of the impaction area. Cell losses after DAPI staining reached nearly 50%. The gelatine coated glass slide had a collection efficiency of almost 40% with more particles in the central region.
The results indicate that silicone sealants were highly efficient for collecting airborne yeast cells. However, silicone sealants can cure with time which may decrease sampling efficiency and which may consequently increase losses due to the DAPI staining procedure.
The curing process of silicone sealants depends predominantly on air humidity. In order to assess the influence of relative humidity and the sampling time used in these experiments on the efficiency of yeast cell deposition and recovery, the number of deposited cells were counted on one of the silicone sealants (“Sista Küchensilikon”) after different sampling times and relative humidities of 20%, 60%, and 100%. The number of deposited cells rose almost linear from 1 to 30 min sampling time (). Relative humidity displayed only a modest influence on the collection efficiency. Most cells were collected at 100%, less at 20%, and fewest at 60% relative humidity. However, the results were within the standard deviation and did not differ significantly. Corresponding tests with the reference filter system for 30 min sampling showed comparable results.
FIG. 7 Number of particles deposited on a silicone sealant coated Diagnostic Slide (“Sista Küchensilikon”) after different sampling times for a relative humidity of —▴ — 20%, … • … 60%, and —▪ — 100% (Mean values of three independent experiments). Δ, ○, and □ represent the number of particles after a sampling time of 30 min with a personal dust sampler GSP 3.5 equipped with a 37 mm filter (pore size 0.45 μm). Each experiment was repeated 3 times.
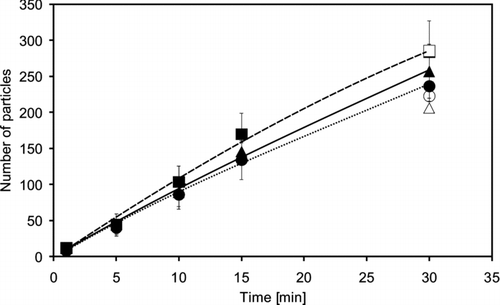
shows the influence of the time spent between preparation of the coating and start of the sampling procedure (storage or curing time) on the deposition efficiency of a typical silicone sealant and the cell losses by DAPI staining after sampling.
FIG. 8 Number of Sacharomyces cerevisiae yeast cells deposited on a silicone sealant coated diagnostic slide (“Sista” Küchensilikon) after 1 minute sampling time (◊) and cell losses after a DAPI staining procedure (⧫. Silicone was cured for different times before sampling.
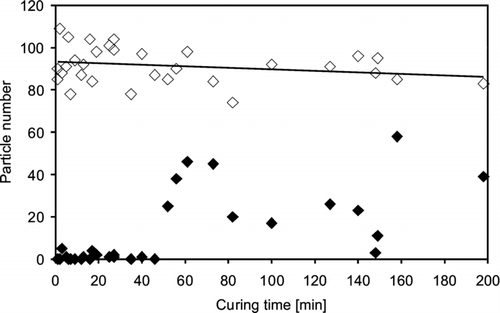
For the experiments microscope slides were coated with silicone sealants and then immediately clamped into the fixture of the impactor. This procedure took only 30 s and does not give the silicone much time for curing. The sampling time was 1 minute. The collection efficiency of the silicone surface dropped only slightly with increasing curing (storage) time between 1 and 200 min. Cell losses by DAPI staining were very low up to curing times of approximately 50 min. Longer storage times led to high irregular particle losses. It seems that the curing process hardened the surface of the silicone sealant and the staining liquid washed away those particles which were not sufficiently impacted. This indicates that slides freshly coated with silicone sealants of the type “Sista” Küchensilikon can be stored up to 50 min before sampling without risking significant losses. The sampling time of 30 min used in the experiments described further above is clearly covered by this time frame.
In summary, the results revealed considerable differences in the collection efficiency, particle distribution in the deposition area and cell losses after DAPI staining of the different adhesive surfaces. Compared to filtration the silicone sealants showed the highest collection efficiency with approximately 95% with all particles located in the central region of the deposition area as well as negligible cell losses and no observable background fluorescence after DAPI staining. During a sampling time of 200 min there was nearly no decline of the number of collected particles on the silicone sealants. Relative humidity had only a slight influence on the collection efficiency. After a curing time of 50 min the amount of cell losses due to DAPI staining rose sharply.
3. Discussion
The sampling efficiency of impactors for qualitative and quantitative microscopic analysis of airborne micro-organisms largely depends on the retention properties of the impaction surfaces. In these investigations 29 surface materials of different origins were tested on their suitability to collect aerolized yeast cells as model organisms from an air stream of a laboratory test tubing system for immediate microscopic analysis with and without DAPI staining.
3.1. Laboratory Test System
A specially constructed test tubing system was used. There are existing various types of laboratory test systems for bio-aerosol experiments (CitationYakovlev 1996; CitationWitschger et al. 1998; CitationMitchell et al. 1988). However, all systems have some limitations, mostly related to a lack of homogeneity and stability in the generated aerosol and to high cell losses in the system itself. Here the aerosol generation was relatively stable (+/– 10%) in the first 20 min, then the number of aerolized particles rose steadily up to 120% after 30 min. Possibly slightly more liquid was nebulized and the cell concentration increased over time or some enrichment effects may have happened in the tubing system. In case of longer sampling times the nebulizer was refilled during operation by an integrated funnel. This can have also increased the particle concentration in the solution and may help to explain some variations in the results.
The approximately 45% cell losses between injection and the sampling probe may have happened by sedimentation in the first stainless steel box (), by impaction in the 90° curve, the first baffle plate or in the 90° curve of the isokinetic sampling probe.
3.2. Reference Filter System
In order to compare the sampling efficiency of the different coating materials with a standard method the GSP 3.5 filter system with fibrous cellulose nitrate filters was used which is well investigated and recommended as reference system by various authors (CitationSpaan et al. 2006; CitationPredicala and Mahhirang 2003; CitationWitschger et al. 1998; CitationSmith and Bird 2002; CitationKenny et al. 1997). The cellulose nitrate filters gained wide acceptance as collection surface because they appeared to have reduced particle bounce (CitationHu 1971; CitationRao and Whitby 1978; CitationSahu et al. 2005). CitationBurton (2007) reported that their physical collection properties was found in the range of >93%. From earlier experiences with cellulose nitrate filters were knew their excellent suitability for the collection and count of Mycoval labelled yeast cells.
The second filtration device (13 mm) was chosen to investigate whether a filter with a smaller diameter has advantages for faster microscopic analysis. However, it turned out that the collection efficiency was only 63% compared to the GSP 3.5. It is assumed that the quite different filter holder design (the air flows through small channels orthogonal to the main flow before passing the filter) and the non-conical air inlet have influenced the air stream and the sampling efficiency negatively.
3.3. Impactor and Assay
Available impactors differ by a number of parameters that affect their efficiency in collection and also the viability of the collected micro-organisms (CitationHenningson and Ahlberg 1994). The round jet impactor used in our investigation as a tool to evaluate the coating materials was chosen because construction and design promised high sampling efficiency, an even distribution of collected particles on the sampling spot and the option for immediate subsequent microscopic analysis. The impact velocity (calculated 41.94 m/s) during collection provides survival of micro-organisms (CitationStewart et al. 1995). This is not relevant for our questions, but certainly useful, for example, prospective recovery experiments. A serious problem can occur by overloading the sampling surface. Overloading can also enhance re-bound effects when because of high particle numbers monolayers are formed on the surfaces and the new particles can't stick anymore (CitationMarple et al. 1993).
CitationTsai and Cheng (1995) showed that the time-varying collection efficiency of a carrier also depends on the surface thickness. Deposited particles change the surface condition such that the particle collection efficiency decreases with time for a coated impaction surface while it increases with time for an uncoated impaction surface. After heavy loading, the collection efficiency eventually approaches nearly the same asymptotic value whether the impaction surface is coated or not (CitationTsai and Cheng 1995). Therefore the continuous monitoring by the Portable Aerosol Spectrometer was important to recognize and avoid overloading. The concentrations of the yeast cells were kept low enough to avoid overlapping of cells on the adherent surfaces and on the filter providing a comfortable cell counting with the microscope.
The design of the sampling surfaces can also have an influence on collection efficiency. CitationTsai and Cheng (1995) found that an impaction surface with an inverted conical cavity alleviates particle bounce and re-entrainment problems during particle collection process, resulting in much greater collection efficiency than conventional design, which uses flat impaction surfaces. Due to the viscosity of the silicone the air stream blows a flat conical “cavity” into the surface which probably enhances the collection efficiency compared to the uncoated and flat glass slides.
3.4. Deposition Spot
The distribution of Saccharomyces cerevisiae cells in the impaction area varied considerably in Figures –. This was true for the number of collected cells and for the distribution in the sampling spot. Most cells were found on the diagnostic slide coated with silicone sealants and all cells were deposited in the centre of the sampling spot. The uncoated microscope slide and the adhesive tape revealed a much lower sampling efficiency and the cells were deposited in a ring-like area around the central spot.
This can be explained by the impactor's design and the airflow within (). Particles were impinged in the central deposition spot, but due to the air-stream and the missing adherence of the glass surface the cells bounced- or blown-off with the airflow. In front of the o-ring sealing there is a dead space regarding the airflow velocity and therefore particles were deposited in this area. The adhesive tape provided a stickier surface for the cells and blow-off of particles impacted in the central spot was reduced. Nevertheless, more than 50% of the deposited cells were still located in the ring-like area. In contrast, all cells deposited on the silicone coated diagnostic slide were located in a central spot and the number of collected particles was the highest for all tested surfaces. Therefore, the problem of cell losses due to particle bounce- or blow-off which was found to lead to some limitations for the direct impaction of particles onto plain surfaces (CitationRao and Whitby 1978; CitationMarple et al. 1993) can be regarded as negligible when applying silicone.
3.5. Different Sticky Surfaces
To improve the retention of aerosol particles on surfaces, numerous types of more or less suitable adherent coatings have been investigated as indicated in the introduction. Nevertheless, one of the most promising ones is silicone. Silicone grease with different viscosities is traditionally used for the collection of micro-organisms and showed relatively high collection efficiencies (CitationComtois and Mandrioli 1997; CitationLundgren 1967; CitationStern et al. 1962; CitationMitchell et al. 1988) which was also found to be true also in this investigation. However, staining procedure resulted in cell losses, which is a disadvantage of this sampling medium. Hogan has found that silicone resin diluted in toluene to 20–30% was a suitable adhesive for coating aerosol collecting foils which were exposed to ambient weather conditions for periods of approximate 30 days (CitationHogan 1970). The author used a silicon resin (SR-516, General Electric, USA) which is not commercially available anymore. Therefore different other resins were tested here. Silicone resin EF (Evonik Tego Chemie GmbH) showed high collection efficiencies (>95%) for the yeast cells and remained sticky for a longer period of time. Most of the collected cells were localized in the central spot, but some were located in the outer area. In addition, after a staining with DAPI more than 50% of the collected cells got lost.
No former data on the collection of airborne micro-organisms or other particles on silicone sealants could be found in literature. The lack of experiments is probably due to the fact, that silicone sealants cure and provide a sticky surface only for a certain time. Surprisingly they seem to be perfect for the collection of airborne yeast cells with a collection efficiency of about 95% and very low cell losses after a DAPI staining procedure, at least when they are not completely cured. The results indicate that a sampling time of about 50 min is possible thenceforward the silicones get contact to the air. From this point the collection efficiency still dropped slowly, but the cell losses during staining rose sharply (). Vulcanization of the silicone sealants takes place through moisture in the air, but the results indicate that there is no clear influence of the relative humidity at least during up to 30 min sampling time (). Due to technical limits of our test system, relative humidities from 0% to 20% as well as the influence of different temperatures could not be tested.
Comtois and Mandrioli (1999) found that Vaseline seemed to be more efficient than silicone for spore capture. Vaseline was not tested here, but chemically comparable Paraffin oil was not suitable for an effective collection due to only 47% collection efficiency and more than 50% cell losses after staining. Moreover, microscopic investigation showed that the oily liquid was blown away by the air stream and the yeast cells impacted on the plain glass slide. CitationRao and Whitby (1978) investigated the particle collection on Dow Corning silicone oil (in 1% Hexane)–coated glass plates. With oil-coated plates, collection efficiency curves rise sharply from 0–100%. However with uncoated plates, efficiency curves rise to a value of 30–50% and drop sharply thereafter. This is in accordance with the findings, that the collection efficiency for both the uncoated glass slide and the commercially available coated but non-sticky microscope slides was under 50%. Furthermore, most of the collected particles were washed off during the staining procedure. The permanent positive charge of the Superfrost® Plus microscope slides was also not strong enough to keep the particles attached to the glass. The pressure sensitive adhesives except SX 1015 showed also collection efficiencies under 50%, but in contrast to the glass slides, more particles could be counted here after staining.
Remarkable is the collection efficiency of the “4 in 1” adhesive sealant of approximately 90% with all particles in the central deposition spot and negligible cell losses after DAPI staining. This indicates a chemical relationship to the silicone sealants. According to the product spec sheet the Flextec® polymer consists of polyether chains of different length with high reactive silanes at their ends respectively. These silanes presumably play a key role for the effective collection of airborne micro-organisms since they are also part of all the silicone sealants in a similar form. Silanes have the general formula RSiX3, where R is an organo-functional group and X is a hydrolysable group, typically an alkoxy group. When silanes are hydrolyzed interactions with a substrate are possible either via hydrogen or covalent bonds (CitationZhang et al. 2000). They are well established as adhesion promoters and surface modifiers. Organo-functional silanes have been employed for the covalent attachment of bacteria (CitationShriver-Lake 1998; CitationShriver-Lake et al. 2002; CitationBearinger et al. 2009) and bio-molecules like DNA (CitationYali et al. 1999; CitationZhang et al. 2000) and proteins (CitationGuo and Zhu 2007) to materials such as glass, silica, or quartz.
4. Conclusions and Outlook
There exist great differences between the tested adhesive surfaces regarding collection efficiency, particle distribution in the deposition area and cell losses after a DAPI staining procedure. In our experiments the different silicone sealants showed the highest collection efficiencies of about 95% compared to the reference filter system. All collected particles were located in the central region of the deposition area. Cell losses after a DAPI staining procedure were negligible when the coated slides were used within 50 min after preparation and background fluorescence was not observed. Relative humidity had only a slight influence on the collection efficiency, therefore silicone sealants could possibly be used as an ideal adhesive coating for the sampling of airborne micro-organisms under different environmental conditions.
An outstanding advantage is that results can be obtained within min. The sample preparation (coating of a diagnostic slide) takes only about 30 s. In high contaminated areas, such as animal housings or organic waste dumps a sampling time of less than 1 min and a flow rate of 1.2 L/min is sufficient to collect high amounts of airborne micro-organisms. The easy application and the small dimensions of the sampling equipment in general make the impaction on silicone-coated glass slides and the following microscopic analysis also a pre-eminent method for personal sampling in work environments. In environments with low concentrations of airborne micro-organisms (e.g., hospitals, clean-room technology) sampling times up to 50 min are possible. After longer sampling times the silicone starts to cure resulting in higher cell losses after staining. A considerable advantage of the tested silicone sealants is their high hydrophobicity. Therefore DAPI fluorescent staining can be applied immediately after sampling and the stain can be removed without any problems after one minute. Furthermore only a low amount of staining solution (10 μL) is necessary due to the small impaction area of approximately 1 mm in diameter. This facilitates also microscopic investigations even with high magnification.
In future other fluorescent dyes and staining procedures should be tested on silicone sealants. An interesting approach would be a staining with, for example, DAPI and PI to discriminate viable and non-viable bacteria. In this regard the relatively low particle velocity in the tested impactor of approximately 40 m/s is favorable for the life-collection of micro-organisms (CitationStewart et al. 1995). For this purpose silicone sealants with a neutral curing system and a pH of 7.0 are probably more suitable to sample sensitive micro-organisms than acetic sealants. Future experiments should also include direct application of FISH techniques, antibody assays or molecular beacons on the silicone surface. This could help to discriminate and identify the collected airborne micro-organisms within short time.
The presented results were obtained with cells of Saccharomyces cerevisiae. Future investigations should demonstrate the usefulness of the tested sampling surfaces for other micro-organisms with smaller sizes and different biological properties. First experiments with vegetative bacteria and spores of Bacillus subtilis, Staphylococcus aureus, and different mold spores indicate promising results.
Thanks go to the Polymer Latex Company in Marl for providing the pressure sensitive adhesives and to the Evonik Tego Chemie GmbH for providing the silicone resins.
REFERENCES
- Adhikari , A. , Sen , M. M. , Gupta-Bhattacharya , S. and Chanda , S. 2004 . Volumetric Assessment of Airborne Fungi in Two Sections of a Rural Indoor Dairy Cattle Shed . Environ. Intern. , 29 : 1071 – 1078 .
- Andersen , C. I. , Von Essen , S. G. , Smith , L. M. , Spencer , J. , Jolie , R. and Donham , K. J. 2004 . Respiratory Symptoms and Airway Obstruction in Swine Veterinarians: A Persistent Problem . Am. J. Ind. Med. , 46 ( 4 ) : 386 – 92 .
- Bearinger , J. P. , Dugan , L. C. , Wu , L. , Hill , H. , Christian , A. T. and Hubbell , J. A. 2009 . Chemical Tethering of Motile Bacteria to Silicone Surfaces . Biotechniques , 46 ( 3 ) : 209 – 216 .
- BGIA . 2001 . “ Institute for Occupational Safety of the German Social Accident Insurance: Geräte ” . In zur Probenahme der einatembaren Staubfraktion (E–Staub). Lfg.27
- Burton , N. C. 2007 . Filter sampling of airborne microbial agents – Evaluation of filter materials for physical collection efficiency, extraction, and comparison to traditional bioaerosol sampling. , University of Cincinnati . Dissertation
- Chang , C.-W. , Hwang , Y.-H. , Grinshpun , S. A. , Macher , L. M. and Willeke , K. 1994 . Evaluation of Counting Error Due to Colony Masking in Bioaerosol Sampling . Appl. Environ. Microbiol. , 60 ( 10 ) : 3732 – 3738 .
- Chi , M.-C. and Li , C.-S. 2005 . Fluorochrome and Fluorescent in Situ Hybridization to Monitor Bioaerosols in Swine Buildings . Aerosol Sci. Technol. , 39 : 1101 – 1110 .
- Chi , M.-C. and Li , C.-S. 2006 . Analysis of Bioaerosols from Chicken Houses by Culture and Non-Culture Method . Aerosol Sci. Technol. , 40 : 1071 – 1079 .
- Chi , M.-C. and Li , C.-S. 2007 . Fluorochrome in Monitoring Atmospheric Bioaerosols and Correlations with Meteorological Factors and Air Pollutants . Aerosol Sci. Technol. , 41 : 672 – 678 .
- Comtois , P. and Mandrioli , P. 1997 . Pollen Capture Media: A Comparative Study . Aerobiologia , 13 : 149 – 154 .
- Davies , C. N. , Aylward , M. and Leacey , D. 1951 . Impingement of Dust from Air Jets . Arch. Ind. Hyg. Occup. Med. , 4 : 354 – 397 .
- Donham , K. J. , Cumro , D. , Reynolds , S. J. and Merchant , J. A. 2000 . Dose–Response Relationships Between Occupational Aerosol Exposures and Cross-Shift Declines of Lung Function in Poultry Workers: Recommendations for Exposure Limits . J. Occup. Environ. Med. , 42 ( 3 ) : 260 – 9 .
- Grinshpun , S. A. , Buttner , M. P. and Willeke , K. 2007 . “ Sampling for Airborne Microorganisms ” . In Manual of Environmental Microbiology , 3rd , Edited by: Hurst , C. J. , Crawford , R. L. , Garland , J. L. , Lipson , D. A. , Mills , A. and Stetzenbach , L. D. 939 – 951 . Washington, D.C. : ASM Press .
- Guo , A. and Zhu , X.-Y. 2007 . “ The Critical Role of Surface Chemistry in Protein Microarrays ” . In Functional Protein Microarrays in Drug Discovery , Edited by: Predki , P. 53 – 71 . Boca Raton : CRC Press .
- Hatch , M. T. and Wolochow , H. 1969 . “ Bacterial Survival: Consequences of the Airborne State ” . In An Introduction to Experimental Aerobiology , Edited by: Dimmick , R. L. and Akers , A. B. 267 – 295 . New York : Wiley Interscience .
- Henningson , E. W. and Ahlberg , M. S. 1994 . Evaluation of Microbiological Aerosol Samplers: A Review . J. Aerosol. Sci. , 25 ( 8 ) : 1459 – 1492 .
- Hogan , A. W. 1970 . Evaluation of Silicone Adhesive as an Aerosol Collector . J. Appl. Meteorol. , 10 : 592 – 595 .
- Horton , K. D. , Ball , M. H. E. and Mitchell , J. P. 1992 . The Calibration of California Measurements PC-2 Quartz Crystal Cascade Impactor (QCM) . J. Aerosol Sci. , 23 ( 5 ) : 505 – 524 .
- Hu , J. N. H. 1971 . An Improved Impactor for Aerosol Studies—Modified Andersen Sampler . Environ. Sci. Technol. , 5 ( 3 ) : 251 – 253 .
- Jekel , J. F. , Katz , D. L. and Elmore , J. G. 2001 . Epidemiology, biostatistics and preventive medicine. , 2nd ed. , 238 Elsevier : New York .
- Kenny , L. C. , Aitken , R. , Chalmers , J. C. , Fabries , J. F. , Gonzalez–Fernandez , E. , Kromhout , H. , Liden , G. , Market , D. , Riediger , G. and Prodi , V. 1997 . A Collaborative European Study of Personal Inhalable Aerosol Sampler Performance . Am. Ocap. Hyt. , 41 ( 2 ) : 135 – 153 .
- Lundgren , D. A. 1967 . An Aerosol Sampler for Determination of Particle Concentration of Size and Time . J. Air Pollut. Control Assoc. , 17 : 225 – 229 .
- Marple , V. A. , Rubow , K. L. and Olson , B. A. 1993 . “ Inertial, Gravitational, Centrifugal, and Thermal Collection Techniques ” . In Aerosol Measurement, Principles, techniques and applications , Edited by: Willeke , K. and Baron , P. A. 229 – 260 . New York : Wiley .
- May , K. R. 1945 . The Cascade Impactor: An Instrument for Sampling Coarse . Aerosols. J. Sci. Instrum. , 22 : 187 – 195 .
- May , K. R. 1975 . An “Ultimate” Cascade Impactor for Aerosol Assessment . J. Aerosol Sci. , 6 : 413 – 419 .
- Mitchell , J. P. , Costa , P. A. and Waters , S. 1988 . An Assessment of an Andersen Mark–II Cascade Impactor . J. Aerosol Sci. , 19 ( 2 ) : 213 – 221 .
- Moschandreas , D. J. , Cha , D. K. and Quian , J. 1996 . Measurement of Indoor Bioaerosol Levels by a Direct Counting Method . J. Environ. Eng. , 122 ( 5 ) : 374 – 378 .
- Parkes , R. J. and Taylor , J. 1985 . Characterization of Microbial Populations in Polluted Marine Sediments . J. Appl. Bacteriol. Symp. Suppl. , : 155 – 173 .
- Predicala , B. Z. and Maghirang , R. G. 2003 . Field Comparison of Inhalable and Total Dust Samplers for Assessing Airborne Dust in Swine Confinement Barns . Appl. Occ. Environ. Hyg. , 18 ( 9 ) : 694 – 701 .
- Radon , K. , Danuser , B. , Iversen , M. , Jörres , R. , Monso , E. , Opravil , U. , Weber , C. , Donham , K. J. and Nowak , D. 2001 . Respiratory Symptoms in European Animal Farmers . Eur. Respir. J. , 7 ( 4 ) : 747 – 54 .
- Rao , A. K. and Whitby , K. T. 1978 . Non-ideal Collection Characteristics of Inertial Impactors I. Single Stage Impactors and Solid Particles . J. Aerosol Sci. , 9 : 77 – 86 .
- Sahu , A. , Grimberg , S. J. and Holsen , T. M. 2005 . A Static Water Surface Sampler to Measure Bioaerosol Deposition and Characterize Microbial Community Diversity . Aerosol Sci. , 36 : 639 – 650 .
- Shriver-Lake , L. C. 1998 . “ Immobilized Biomolecules in Analysis ” . In A Practical approach , Edited by: Cass , T. and Ligler , F. S. 1 Oxford : Oxford University Press .
- Shriver-Lake , L. C. , Gammeter , W. B. , Bang , S. S. and Pazirandeh , M. 2002 . Covalent Binding of Genetically Engineered Microorganisms to Porous Glass Beads . Anal. Chim. A. , 470 : 71 – 78 .
- Smith , J. P. and Bird , A. J. 2002 . Relationship of Sampling Efficiency for Manikin-Mounted Personal Samplers to Efficiency Measurements Made Independent of Manikin . J. Aerosol Sci. , 33 ( 9 ) : 1235 – 1259 .
- Spaan , S. , Wouters , I. M. , Oosting , I. , Doekes , G. and Heederik , D. 2006 . Exposure to Inhalable Dust and Endotoxins in Agricultural Industries . J. Environ. Monit. , 8 : 63 – 72 .
- Stern , S. C. , Zeller , H. W. and Schekman , A. I. 1962 . Collection Efficiency of Jet Impactors at Reduced Pressures. . Ind. Eng. Chem. Fundamen. , 1 ( 4 ) : 273 – 277 .
- Stewart , S. L. , Grinshpun , S. A. , Willeke , K. , Terzieva , S. , Ulevicius , V. and Donnelly , J. 1995 . Effect of Impact Stress on Microbial Recovery on an Agar Surface . Appl. Environ. Microbiol. , 61 ( 4 ) : 1232 – 1239 .
- Terzieva , S. , Donnelly , J. , Ulevicius , V. , Grinshpun , S. A. , Willeke , K. , Stelma , G. N. and Brenner , K. P. 1996 . Comparison of Methods for Detection and Enumeration of Airborne Microorganisms Collected by Liquid Impingement . Appl. Environ. Microbiol. , 62 ( 7 ) : 2264 – 2272 .
- Tong , Y. and Lighthart , B. 1999 . Diurnal Distribution of Total and Culturable Atmospheric Bacteria at a Rural Site . Aerosol Sci. Technol. , 30 : 246 – 254 .
- Tsai , Ch.-J. and Cheng , Y.-H. 1995 . Solid Particle Collection Characteristics on Impaction Surfaces of Different Designs . Aerosol Sci. Tech. , 23 ( 1 ) : 96 – 106 .
- Witschger , O. , Willeke , K. , Grinshpun , S. A. , Aizenberg , V. , Smith , J. and Baron , P. A. 1998 . Simplified Method for Testing Personal Inhalable Aerosol Samplers . J. Aerosol Sci. , 29 ( 7 ) : 855 – 874 .
- Yakovlev , S. A. 1996 . Test Rig for Checking Aerosol Devices and Systems for Monitoring the Cleanliness of an Industrial Atmosphere . Meas. Tech. , 39 ( 2 ) : 141 – 145 .
- Yali , C. , Lin , G. and Zuhong , L. 1999 . Using Silane Coupling Reagents in Fabrication of DNA Arrays on Glass Support . Moi Crysi. Liq. Clysf. , 337 : 477 – 480 .
- Zhang , G. , Zhou , Y. , Wu , X. , Yuan , J. and Ren , S. 2000 . Covalent Attachment of DNA to Glass Supports Using a New Silane Coupling Agent and Chemiluminescent Detection . J. Tongji Med. Univ. , 20 ( 2 ) : 89 – 91 .