Abstract
The aim of this work was to compare the fractal characteristics, Df and kf, the primary particle diameter, Dpp, the gyration diameter of aggregates, Dg, and the overlap coefficient, Cov, of carbon nanoparticle aggregates produced by an ethylene diffusion flame and sampled by means of four commonly used techniques. The first method involves a thermophoretic piston probe (TPP) which inserts a TEM grid into the flame. Three other methods were applied at the outlet of a dilution device, also inserted in the flame. The first of these used a nuclepore filtration sampler (NFS), and is based on filtration of particles onto a polycarbonate membrane. The second, post dilution method, the insertion particle sampler (IPS), inserts a TEM grid, perpendicular to the aerosol flow. Similar to TPP, the last method is a thermophoretic particle sampler (TPS) sampling directly onto a TEM grid. After collection, the samples are stored in the dark either, (1) in a nitrogen filled cell at low humidity or, (2) in ambient air for studying atmospheric ageing. Good agreement was observed between TPP, TPS, and IPS indicating that the dilution induced for TPS and IPS does not significantly change the morphology of soot. On the other hand, the NFS protocol tended to overestimate the overlap coefficient and the size of primary particles and aggregates. Finally, with regard to the aging effect, we found that kf and Dpp evolve slowly during storage in the atmosphere while Df, was insensitive to the storage conditions. However, the overlap coefficient increased and the gyration diameter decreased as a function of storage duration, while storage under nitrogen tended to reduce these changes.
NOMENCLATURE
![]() | = |
: amplitude of the size distribution of primary particle diameters and aggregate gyration diameters |
![]() | = |
: projected overlap coefficient of two overlapped primary particles |
![]() | = |
: mean projected overlap coefficient of a population of aggregates |
![]() | = |
: overlap coefficient of a population of aggregates |
![]() | = |
: distance between the centers of mass of two overlapped primary particles (nm) |
Df | = |
: fractal dimension |
Dg | = |
: gyration diameter of the aggregate (nm) |
![]() | = |
: modal gyration diameter of the aggregate (nm) |
Dpp | = |
: primary particle diameter (nm) |
![]() | = |
: mean primary particle diameter (nm) |
IPS | = |
: insertion particle sampler |
ka | = |
: empirical constant (1.095) |
kf | = |
: fractal prefactor |
N(Dpp) | = |
: primary particle diameters’ size distribution |
Nbpixel | = |
: number of pixels composing the TEM micrograph of the aggregate |
NFS | = |
: nuclepore filtration sampler |
Npp | = |
: number of primary particles composing an aggregate |
p(Dg) | = |
: probability density function of gyration diameters |
Rg2D | = |
: projected radius of gyration of the aggregate (nm) |
Sag | = |
: projected surface area of the aggregate (nm2) |
Spp | = |
: projected surface area of a primary particle (nm2) |
TEM | = |
: transmission electron microscopy |
TPP | = |
: thermophoretic piston probe |
TPS | = |
: thermophoretic particle sampler |
(![]() | = |
: coordinates of a given pixel on the TEM micrograph of the aggregate |
![]() | = |
: coordinates of the centre of mass of the aggregate |
GREEK SYMBOLS
α | = |
: empirical constant (1.155) |
ς1, ς2 | = |
: empirical constants (ς1=1.1±0.1 and ς2=0.2±0.02) |
σpp | = |
: standard deviation of the primary particle diameter size distribution (nm) |
σgeo | = |
: geometric standard deviation of the aggregate gyration diameter size distribution |
1. INTRODUCTION
Soot particles produced during combustion of hydrocarbons or organic matter are of strong interest in the fields of environmental science (global warming contribution, air pollution), fire science (radiative impact of soot particles on fire propagation), air filtration (clogging of high efficiency particulate air filters, CitationMocho and Ouf 2010), and combustion science (contribution of soot to the heat flux of flames, optimization of combustion processes). Since the pioneering work of CitationWitten and Sander (1981) and the application of the fractal theory of CitationMandelbrot (1973) by CitationJullien and Botet (1987), many investigations have been reported which describe the specific morphology of particles produced by diffusion flames (CitationDobbins and Megaridis 1987; CitationMegaridis and Dobbins 1990; CitationKöylü and Faeth 1992; CitationSorensen and Feke 1996; CitationOuf et al. 2008), by automotive engines (CitationKittelson 1998; CitationSachdeva and Attri 2008) and by aircraft engines (CitationColbeck et al. 1997; CitationPetzold et al. 1999; CitationPopovitcheva et al. 2000; CitationBarone et al. 2006). Because of their diffusion limited mode of aggregation, soot particles display well-known fractal morphology and several methods for analysis of their shape have been proposed in the literature (CitationKöylü et al. 1995; CitationBrasil et al. 1999; CitationXiong and Friedlander 2001; CitationBushell et al. 2002; CitationLee and Kramer 2004; CitationTian et al. 2006). Soot particles usually consist of small primary particles (diameter Dpp) that agglomerate to form irregular clusters. Their complex geometry can be described by a relationship, known as a fractal-law, between the number of primary particles in the agglomerate, Npp, which is proportional to the mass, and one particular agglomerate parameter, Rg, the radius of gyration. Three other parameters are included in this relationship, the primary particle diameter Dpp, the fractal dimension Df and the prefactor kf.
Even though in-situ methods are available for measurement of the fractal dimension Df, by measuring the angular light scattering for example (CitationSorensen et al. 1992; CitationSorensen 2001; CitationKim and Choi 2003), most studies take advantage of ex-situ methods which allow direct observation of the particles using Transmission Electron Microscope (TEM) projected images. In this case, the two-dimensional information can be extrapolated to determine three-dimensional properties by applying simple relationships (CitationMedalia and Heckman 1969; CitationSamson et al. 1987; CitationCai et al. 1993; CitationRogak et al. 1993; CitationKöylü et al. 1995; CitationNeimark et al. 1996; CitationPark et al. 2004; CitationIshiguro et al. 1997; CitationWentzel et al. 2003; CitationChen et al. 2006; CitationTian et al. 2006; CitationChandler et al. 2007; CitationChung et al. 2008).
Various methods are used in order to deposit nanoparticles onto TEM grids. The thermophoretic method, based on a rapid insertion (as short as 30 ms) of a TEM grid in the flame, was first introduced by CitationDobbins and Megaridis (1987) and has since been widely used (CitationCai et al. 1993; CitationKöylü et al. 1997; CitationTian et al. 2004; CitationLee et al. 2008). However, other sampling methods, involving insertion of a dilution probe into the flame or plume region, are also employed (CitationVan Hulle et al. 2002; CitationMatti Maricq et al. 2003; CitationZhao et al. 2003; CitationOuf et al. 2008; CitationTeng et al. 2009). In these methods, a sampling probe is inserted in the vicinity of the flame and rapid quenching is carried out in order to prevent agglomeration, nucleation or water condensation at the surface of the soot. At the outlet of these dilution systems, particles can either be deposited directly onto a membrane (CitationSpurny 1986; CitationColbeck et al. 1997; CitationOuf et al. 2008) or onto TEM grids using specific protocols primarily based on impaction, interception and diffusion processes (CitationSorensen and Feke 1996; CitationVan Hulle et al. 2002) or thermophoresis (CitationBang et al. 2003; CitationThomassen et al. 2006; CitationWen and Wexler 2007).
FIG. 1 The burner and the experimental setup used to sample soot particles in the ethylene diffusion flame.
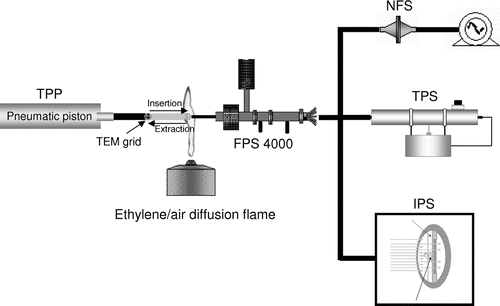
The main aim of this study is to investigate the possible influence of the sampling methods, currently widely used in the community, on soot morphological parameters (kf, Df, Dpp, Dg, Cov) determined using TEM images. To this end, four methods for sampling and deposition of soot onto TEM grids have been applied simultaneously to an ethylene diffusion flame.
Several studies using high resolution TEM have shown that monomers are composed of planar nano-crystallites contained in an outer shell and an inner core; this core may incorporate several fine spherical particles (CitationIshiguro et al. 1997; Vander Wal 1997; CitationWentzel et al. 2003). All these structures show some graphite-like order. Variations of the inter-planar spacing have been observed (CitationShaddix et al. 2005; CitationKis et al. 2006) and can be attributed to the nature and number of the molecules that are absorbed on the planar graphitic nano-structure. Initially, the organic matter content of soot particles is high and there is a low oxygen concentration. During storage, exchange of molecules with the air is possible, which could give rise to variations in the primary particle structure. Moreover, according to recent reports in the literature (CitationYezerets et al. 2003; CitationKandas et al. 2005; CitationBraun 2009), soot particle ageing may occur even under ambient conditions of temperature, pressure, humidity, and sunlight. More precisely, results have been reported on the ageing of soot, under ambient conditions, induced by ozone (CitationDecesari et al. 2002), by desorption and oxidation (CitationCheng et al. 2006; CitationBraun 2009; CitationCheng and Lehmann 2009), by water uptake (CitationPopovitcheva et al. 2008), or by photolysis of polycyclic aromatic hydrocarbons (PAH, Kim et al. 2009) and all these phenomena are able to change the microstructure and composition of such particles (CitationIshiguro et al. 1991; CitationHurt et al. 1993; CitationPopovitcheva et al. 2008; CitationKim et al. 2009). Hence, in the present work, the influence of storage duration, preservation conditions and sample preparation procedures, all of which could have influenced the soot morphological parameters, are also investigated by TEM analysis.
2. EXPERIMENTAL PROCEDURE
2.1. Diffusion Flame Burner
The soot production apparatus is shown in . It consists of an atmospheric hybrid Holthuis burner (previously McKenna burner) equipped with a 60 mm diameter porous bronze sintered matrix around a central 6.35 mm diameter tube allowing the introduction of an injector (CitationLemaire et al. 2009) or gas flow. In the present study, a diffusion flame was obtained by injecting ethylene at a rate of 1 L/min into the central part of the burner. An air flow of 38 L/min passed through the annular porous component of the burner in order to stabilize the flame. The flame height obtained was around 10 cm.
As depicted in , in-situ (Thermophoretic piston probe, TPP) and ex-situ sampling methods (dilution probe FPS 4000) were applied at the same location in the flame, at a height above the burner equal to 4 cm, in a region where the particle concentration is large. also shows three other sampling protocols, a thermophoretic particle sampler, TPS, an insertion particle sampler, IPS, and a Nuclepore filtration sampler, NFS, each implemented at the outlet of the dilution probe.
2.2. Sampling Procedures
Four sampling methods have been compared using this experimental device for soot production. The first involved inserting a TEM grid into the diffusion flame while the three other methods were applied at the outlet of the dilution probe. The techniques are mainly based on thermophoresis, or impaction, interception, and diffusion deposition on TEM grids, or filtration of soot particles by a polycarbonate membrane. Sampling protocols have been considered according to their distinct fields of application. The TPP device is widely used in the field of combustion science, in order to study soot particles inside flames. The three others devices (TPS, IPS, and NFS) are designed to sample particles under more diluted and moderate conditions, such as in flame plumes or in indoor or outdoor environments.
2.2.1. Thermophoretic Piston Probe (TPP)—Direct Flame Sampling
In the work reported here, a special Thermophoretic Piston Probe (TPP), similar to the one proposed by CitationDobbins and Megaridis (1987) and Köylü et al. (1997), was used to collect soot particles on a TEM grid (CitationCai et al. 1993; CitationKöylü et al. 1997; CitationTian et al. 2004; CitationLee et al. 2008). A TEM grid is inserted parallel to the flame axis using a pneumatic piston. The grid immobilization time at the center of the flame (10 to 1000 ms) is optically controlled (). Such an orientation promotes thermophoretic deposition of particles onto the grid, due to the temperature difference between the flame and the grid.
2.2.2. Dilution Device for Ex-Situ Sampling Systems
In contrast to the insertion of TEM grids using a thermophoretic piston, ex-situ techniques for measurements of size distribution (CitationMatti Maricq et al. 2003; CitationZhao et al. 2003; CitationTeng et al. 2009), mass concentration (CitationOuf et al. 2008), and optical properties (CitationZhu et al. 2000; Van-Hulle et al. 2002; CitationOuf et al. 2008), require continuous sampling at non-negligible flow rates. However, a rapid quenching of some physical and chemical phenomena (agglomeration, nucleation, and oxidation, for example) needs to be performed. In the present study, in order to compare the different methods used to deposit soot particles on TEM grids or membranes, soot particles were sampled in the flame using a two-stage dilution device () (DEKATI FPS 4000, Mikkanen et al. 2002; Ouf et al. 2008). The sampling probe was heated to 180°C to avoid condensation of volatile vapor and steam on its inner surfaces. The first dilution was performed with heated filtered air (180°C), passing through a porous tube to decrease the dew point of the sample. Then, a second dilution was applied to the sampled volume using cold air passed into an ejector-type dilution device. Therefore, the sample was at ambient temperature and pressure after leaving the probe and a dilution ratio of approximately 100 was used. In this study, three different methods were used to deposit soot particles onto TEM grids, as shown in , and these are detailed below.
2.2.3. Thermophoretic Particle Sampler (TPS)
The Thermophoretic Particle Sampler is a system specifically developed to collect particles at ambient conditions; in this study, from the exhaust of the dilution system. This system was developed by the Fraunhofer Institute of Toxicology (Germany) and it is composed of a heated (150°C) sampling tube in which a TEM grid is inserted parallel to the aerosol flow (3 L/min) and cooled using a Peltier module to 20°C. This device has been recently used and described by CitationThomassen et al. (2006). Using the TPS, soot particles are deposited directly onto TEM grids without any treatment. The main physical phenomenon responsible for particle deposition on the TEM grid is thermophoresis as previously discussed. Comparison of the two techniques (TPP and TPS) allows the effects of sampling and dilution to be investigated independently of other possible effects.
TABLE 1 Gaussian fit parameters for the four sampling protocols TPP, TPS, IPS, and NFS, with standard deviation in parentheses
2.2.4. Insertion Particle Sampler (IPS)
The second device was developed by CitationVan Hulle (2002) for sampling onto TEM grids in the exhaust of a dilution probe inserted in a diffusion flame. This method, with a grid inserted perpendicularly to the aerosol flow, is based, depending on the particle size, on interception, impaction or diffusion of particles directly onto TEM grids. No information is available on its collection efficiency and its size dependence, nevertheless this very simple device has been implemented in order to demonstrate its potential suitability for sampling soot particles in such situations.
2.2.5. Nuclepore Filtration Sampler (NFS)
In parallel to the TPS and IPS, a filter holder was used for sampling soot particles at the outlet of the particle dilution device (). Polycarbonate membranes with a pore size of 0.2 μm (Whatman Nuclepore) were used and a post-treatment was applied in order to transfer the soot particles from the membrane to the TEM grid.
In this experiment, TEM grids were observed without any particular preparation in the case of the TPP, TPS, and IPS procedures. However, a specific treatment needed to be applied to the Nuclepore membranes of the NFS procedure, following CitationSpurny (1986). After sampling, particles were transferred onto TEM grids (200 mesh standard copper grids, Agar Scientific) according to the following protocol. First, the membrane was coated with a graphite film by vacuum evaporation (Jeol JEE 400). Next, a piece of coated polycarbonate membrane was placed on the TEM grid and then partially dissolved using chloroform. In this way particles were sandwiched between polycarbonate membrane remnants and evaporated graphite film. The influence of such a treatment on the morphology and size of soot particles (primary particles and aggregates) will be discussed below.
2.3. Storage Procedure
During the experimental procedure, two batches of samples (grids or membranes) were collected for each sampling protocol. The first batch of samples was stored away from light to avoid photolysis as reported by CitationKim et al. (2009), in a chamber filled with nitrogen (100 mbar) at a relative humidity of 15% and at a mean temperature of 20°C. The second batch of samples was stored under ambient conditions in the laboratory (20°C, 65% RH and also away from light). Moreover, to investigate soot ageing effects, morphology was studied as a function of storage times up to 10 months in ambient air.
3. MORPHOLOGICAL ANALYSIS
3.1. TEM Image Production
After sampling onto TEM grids (200 mesh copper grid with Formvar/carbon film, Agar Scientific) and on polycarbonate membranes (Whatman Nuclepore, with a pore size of 0.2 μm), transmission electron microscopy was used to obtain soot images for determination of the primary particle size and the fractal dimension of the aggregates. Soot particles were studied using a JEOL 100CXII TEM at magnifications from × 72.000 to × 320.000 with an accelerating voltage of 100 kV. For each magnification, polystyrene latex spheres of known diameter, 102 ± 3 nm (Duke Scientific), observed under the same conditions as the soot, were used to calibrate the images and convert dimensions from pixel numbers to a nanometer scale. A Dualvision 3000W model 780 CCD camera (Gatan Inc.) coupled to the microscope generated digital images for analysis.
Approximately 100 to 200 micrographs of individual soot particles were collected for each experimental condition. These were taken randomly at various locations across the grid. The primary particle diameters were manually determined from these micrographs.
3.2. Image Analysis Procedure to Determine the Morphological Parameter
This section describes the morphological analysis procedure based on the TEM micrograph treatment. Two analyses were performed by two different operators, each using different analysis software, since it was anticipated that the results of some steps in the image analysis might be influenced by contributions from the software.
For spherule diameters, the differences were always less than the observed dispersion, which is shown below. No systematic biases were observed for the fractal dimension and prefactor. For this reason, the results presented have been obtained after merging the two data sets.
3.2.1. Determination of Primary Particle Diameters
Many authors have reported primary particle diameter measurements for a large variety of fuels (CitationDobbins and Megaridis 1987; CitationMegaridis and Dobbins 1990; CitationKöylü and Faeth 1992; CitationSorensen and Feke 1996; CitationKittelson 1998; CitationVan Hulle et al. 2002; CitationBarone et al. 2006; CitationOuf et al. 2008). The analysis procedures are based on ImageJ (Rasband 1997–2009) and Saisam (Microvision Instruments) software and the diameters of the primary particles are measured by superposing a circular shape on the apparent primary particles. From 700 to 1000 primary particles (cf. ) were analyzed for each experimental condition and a histogram was generated from these measurements using Sigmaplot (Systat software) or Saisam software. Finally a normal size distribution fit is applied to the experimental results according to Equation Equation1, where is the most probable diameter, σ
pp
is the standard deviation and A is the amplitude of the distribution (A is not presented hereafter):
3.2.2. Determination of Fractal Parameters
The second step for fractal analysis of aggregates consists of converting their TEM projected images into binary, black/white, images of the aggregates and background respectively, for a given threshold setting. In this study, TEM image segmentations were processed using Adobe Photoshop and Adobe Photoshop Elements software (Adobe Systems). Then, the binary images obtained were analyzed, with software developed in the Matlab environment, to determine the morphological characteristics on a projected image of the aggregates such as the maximum length, maximum height, width, projected area, perimeter, and Feret diameter.
Following the pioneering work of CitationWitten and Sanders (1981), the fractal nature of soot particles has been widely confirmed (CitationJullien and Botet 1987; CitationMegaridis and Dobbins 1990; CitationKöylü and Faeth 1992; CitationKöylü et al. 1995). A soot particle is an aggregate of spherical primary particles. The number of primary particles, Npp, composing this aggregate is related to the two characteristic diameters, the gyration diameter, Dg, and the primary particle diameter, Dpp, by:
It should be noted that various procedures are available in the literature to determine the fractal dimension and prefactor from projected images. For example, one procedure is based on the maximum length L of the aggregates (CitationKöylü et al. 1995; CitationBrasil et al. 1999) and another is based on the box-counting method (CitationXiong and Friedlander 2001). Nevertheless, the aim of the present work is not to compare these different procedures but, for a given procedure, to compare the effects of the sampling and storage methods. Hence, it was decided to use the approach, based on the gyration diameter Dg, as described by Köylü et al. (1995) which is widely used in the literature (CitationSorensen and Feke 1996; CitationHu et al. 2003; CitationPark et al. 2004; CitationOuf et al. 2008) and by the combustion research community.
First the primary particle projected surface area, S pp , and the aggregate projected surface area, Sag, were directly determined from TEM micrographs, in pixels. The ratio Sag/Spp defines a two-dimensional number representative of the primary particles composing the aggregate. To obtain the three-dimensional number of primary particles that compose the aggregate, Npp, the following empirical relationship was used:
3.2.3. Determination of the Overlap Coefficient
A significant parameter for describing soot particles as agglomerate or aggregate is the overlap coefficient. By definition, an agglomerate is composed of primary particles bonded together by weak, Van der Waals type, forces and exhibiting a low degree of overlap. Conversely, an aggregate is composed of strongly bonded primary particles (covalent type bonds) with a high degree of overlap. CitationBrasil et al. (1999) defined the projected overlap coefficient Cov,P of two touching primary particles, with a mean diameter of and a distance between the centers of mass of
, as:
4. EXPERIMENTAL RESULTS
shows TEM micrographs of soot obtained using the four different sampling protocols. Note that the holes observed on the NFS micrograph are the 0.2 μm pores of the polycarbonate membrane. The density of deposited particles cannot be compared because the exposure times and physical processes involved are not the same in each case. Nevertheless, qualitative observation shows that the size of deposited particles depends on the chosen protocol. The TPP method presents the highest number of weakly agglomerated particles because these are taken directly from the flame. TPS and IPS exhibit medium sized particles which may be due to agglomeration in the dilution probe. Finally, the NFS micrograph clearly shows that the NFS protocol enables observation of the largest particles, which cannot be collected by the other techniques (TPP, TPS, and IPS).
FIG. 2 TEM micrographs of samples collected by TPP, TPS, IPS, and NFS and stored in a N2 atmosphere in the dark.
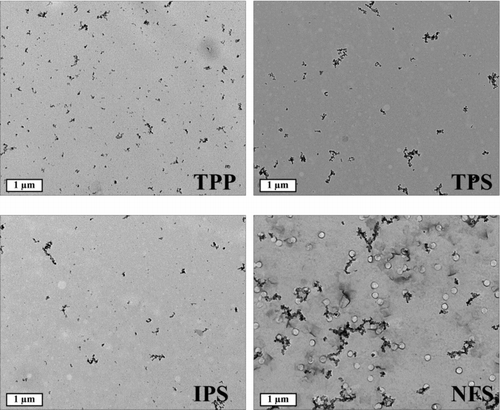
4.1. The Influences of Sampling Protocols
In order to compare morphological properties of the soot particles obtained from the four distinct procedures, the samples were stored in a chamber filled with nitrogen at a relative humidity of 15% and in the dark. For these samples, TEM micrographs were obtained after 60 to 100 days of storage in nitrogen.
4.1.1. Primary Particle Diameter and Overlap Coefficient
Following earlier authors (CitationHu et al. 2003; CitationTian et al. 2004; CitationOuf et al. 2008), the size distributions have been fitted by a Gaussian curve, and the parameters obtained, mean values, are given in . From consideration of the direct sampling of soot particles on TEM grids (TPP, TPS, IPS), the most probable primary particle diameter lies in the range from 24.4 to 26.9 nm and this is in good agreement with the values of 19 to 35 nm and 28.3 nm reported respectively for turbulent and laminar non-premixed ethylene/air flames by CitationHu et al. (2003) and CitationTian et al. (2004). However, it is clear that the NFS protocol tends to over-estimate primary particle diameter by more than 10 nm.
Few values are available in the literature for the overlap coefficient. However, the present results are in good agreement with Cov values reported by CitationWentzel et al. (2003) for diesel soot (ranging from 0.10 to 0.29) and with the values considered by CitationBrasil et al. (1999) in their numerical study (ranging from 0 to 0.33). As with the primary particle diameter, the NFS protocol over-estimates the overlap coefficient by a factor of 1.2 to 1.4.
shows that, for the primary particle diameter and overlap coefficient, the differences between TPP, TPS, and IPS results are small. Since no differences are observed, this suggests that no transformation process occurred which resulted in variation of the primary particle diameter during the early stages of the sampling in the dilution probe FPS.
4.1.2. Aggregate Size, Prefactor, and Fractal Dimension
Turning now to the aggregate size; presents the probability density function p(D g ) in terms of gyration diameter obtained for the different sampling protocols and their associated log-normal fitting (Equation (Equation10)):

For the TPP, good agreement is observed between present results and published results of CitationMegaridis and Dobbins (1990) and CitationHu et al. (2003) which, using the same sampling protocol, reported mean gyration diameters for soot from ethylene/air diffusion flames in the ranges 94–178 nm and 34–106 nm, respectively. From comparing modal diameters between thermophoretic sampler TPP and TPS (see ), it could be concluded that an agglomeration occurs in the dilution system prior to the TPS. However, as the temperature gradients and sampler characteristics are significantly different between these two methods, this discrepancy in diameter may also be explained by different collection efficiencies. For sampling at the outlet of the dilution device, the IPS size distribution returns lower diameters than TPS whereas the value from NFS is significantly larger than both of these (). Furthermore, the IPS result is in good agreement with the TPP measurement which is considered to be the reference method in the field of combustion aerosol science. Since NFS is a filtration based method, its collection efficiency is not as size dependent as the other methods. Hence, one may assume that it is the most representative sampler in terms of aggregate size distribution but, as will be reported in the next section, the post-treatment induced by the NFS, overestimates the primary particle diameter and is suspected to displacing the size distribution to larger diameters.
The conclusion on the effect of sampling method on size distribution is that modal gyration diameters depend on the sampling method and range from 79 to 267 nm. The discrepancies observed between gyration diameters could be due to the different collection efficiencies of the sampling devices for which few data are available in the literature. However, as the number of aggregates used for determining the gyration diameter size distribution is statistically low (around 200), the quantitative interpretation of the size distribution established by TEM must be considered cautiously.
FIG. 4 The number of primary particles in the aggregates as a function of the ratio between gyration and primary particle diameters.
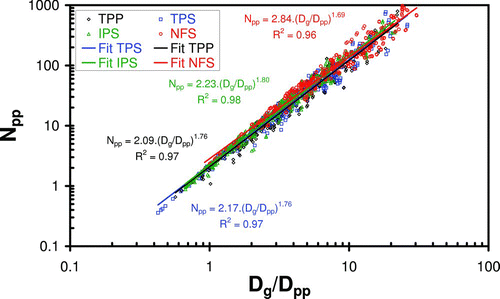
In a similar way the influence of sampling protocols on the prefactor, kf, and on the fractal dimension, Df, has been studied. As explained above, these parameters can be determined by plotting the logarithm of the number of primary particles composing the aggregate, Npp, as a function of the logarithm of the ratio of the aggregate gyration diameter and the primary particle diameter. For convenience, Npp is shown below as a function of Dg/Dpp () on a log-log scale. Even taking into account the scatter in the data points, it appears that the fractal parameters determined by the four sampling protocols are in good agreement and are not dependent on the sampling procedure. Linear fits, presented in , give the values obtained for Df and kf following the analysis procedure described above. The uncertainties in Df and kf are computed according to the statistical analysis described by CitationOuf et al. (2008).
From , it is evident that, when normalized according to the primary particle diameter, the gyration diameter for particles collected with the NFS tends to be larger than those collected with other samplers. This is consistent with the size distributions that were shown in . also shows that the fractal dimensions range from 1.69 to 1.80 and the fractal prefactors from 2.09 to 2.84. The NFS method provides the lowest value for Df and the highest value of kf. This is mainly due to applying the linear regression to larger aggregates for the NFS method than for the other protocols. These results are in good agreement with previous results of Köylü et al. (1997) and CitationHu et al. (2003) who reported Df= 1.72 with kf= 2.8 and Df= 1.74 with kf= 2.2, respectively. There is a controversy in the literature regarding kf and, while our present results are in agreement with previous works of Köylü et al. (1997), CitationHu et al. (2003), CitationWentzel et al. (2003), CitationTian et al. (2006), CitationOuf et al. (2008), some numerical simulations (CitationSorensen and Roberts 1997; CitationOh and Sorensen 1997; CitationBrasil et al. 1999) and experimental characterization (CitationCai et al. 1993; CitationCai et al. 1995; CitationSorensen and Feke 1996) refer to kf values less than 2. For numerical simulations the discrepancy observed with our results could be associated to the overlap coefficient which, according to CitationBrasil et al. (1999), tends to increase kf as it increases. Our measurements involved only one fuel (ethylene) and one method for calculating Df and kf. They are therefore not sufficiently comprehensive to resolve this discrepancy. Nevertheless, Df and kf were similar for the sampling methods that we used.
4.1.3. Analysis of the NFS Sampling Artefact
Discrepancies observed between direct sampling protocols (TPP, TPS, IPS) and Nuclepore filtration sampling (NFS) may be due to the transfer procedure from the Nuclepore to the TEM grid which consists of: (Equation1) carbon deposition on the membrane surface and (Equation2) dissolution of the membrane in chloroform. To separate the effects of these two treatments, a carbon deposition was applied to some TPP and TPS grids, and then the TPS grid was dissolved in chloroform. Morphology (Df, kf, Dpp, Dg) and mean distances between centers of mass of primary particles used for the Cov measurement, are reported in .
It is clear that the carbon deposition significantly influences the primary particle diameter and the mean distance between the centers of mass of the primary particles. For the TPP and the TPS methods, an increase in Dpp of 28 and 46% was identified whereas the influence of CHCl3 was not as significant, with less than 4% increase in primary particle diameter. The same effect has been observed for the overlap coefficient with an increase in this parameter of between 13 and 35% for the carbon deposition and of more than 21% for the CHCl3 dissolution. This means that carbon deposition and chloroform dissolution both have an impact on the observed size of monomers and the distances between them. In a same way a growth of 20 to 30 nm is observed in the gyration diameter of the aggregates. Simultaneously, an increase of 15 nm was observed in the diameter of calibrated polystyrene latex sphere (102 nm) after carbon deposition. The present study shows that the carbon coating and chloroform dissolution involved in the NFS method is responsible for the growth in apparent size of the particle. This cannot be corrected for since it seems to be dependent of the particle's nature or shape.
TABLE 2 Influence of the NFS transfer procedure on morphological parameters. This influence is analyzed by applying carbon deposition (C) to TPP and TPS grids (standard deviation for Dpp and geometric standard for Dg in parentheses)
TABLE 3 Evolution of morphological parameters as a function of storage duration in air and nitrogen; with standard deviation for Dpp and geometric standard deviation for Dg in parentheses. The initial values for TPP with nitrogen correspond to results analyzed in the previous section (; )
4.2. Storage Protocols Artefacts
The evolution of the morphological parameters (Dg, Dpp, Df, Cov, and kf) of soot particles, during long periods of storage at ambient conditions, was also investigated. Sampling was performed in similar ways to the previous analysis, “Sampling protocols influence,” however, two series of samplings (for both TPP and NFS) were undertaken and one set was stored in air, while the other was stored under nitrogen. Particle morphology was analyzed after approximately one, three, six, and ten months of storage in air under ambient temperature, pressure and relative humidity, while for nitrogen only two storage durations were investigated. Then, the determination of the morphological parameters was carried out as a function of storage duration in air; the results are presented in . The modal gyration diameter and its geometric standard deviation have been determined by log-normal fitting (Equation (Equation10)) applied to the probability density functions.
The results presented in show that the gyration diameter is sensitive to the storage environment. The observation that the gyration diameter decreases with increasing storage duration suggests that this evolution is more likely to be due to contraction of the primary particles than to collapse of the agglomerates. Nonetheless, no significant variations and no particular trends are observed for Df as a function of storage duration and corresponding values, measured under ambient conditions, are in agreement with the initial values obtained for samples stored under nitrogen. Therefore, the decrease in the gyration diameter may not be assumed to be due to a collapse of the aggregate alone but is more strongly related to a contraction of the primary particles.
For Dpp, because of the associated uncertainties and standard deviation, the trend of its variation is not clear; however, a slight decrease is observed during the first 100 days, followed by an increase. On the other hand, as shown in , a constant increase of the overlap coefficient as a function of the storage duration is observed for both samples in air. This increase appears to be a linear function of the storage duration and the slope is similar for the two different sampling methods, TPP and NFS. This indicates that the phenomenon is not mitigated by the specific treatment applied in the case of the NFS method (carbon deposition and chloroform dissolution). As the overlap coefficient increases, the primary particles coalesce with one another and this phenomenon explains the decrease in terms of gyration diameter. According to numerical studies (CitationSorensen and Roberts 1997; CitationBrasil et al. 1999) as the overlap coefficient increases, the prefactor kf is expected to increase. Nevertheless, for the present results, within the experimental uncertainties, the link between Cov and kf is not obvious and only a slight increase of the prefactor can be observed in .
FIG. 5 Evolution of the overlap coefficient as a function of the storage duration in air and nitrogen (three TEM micrographs are shown as an illustration of the increase in the overlap coefficient).
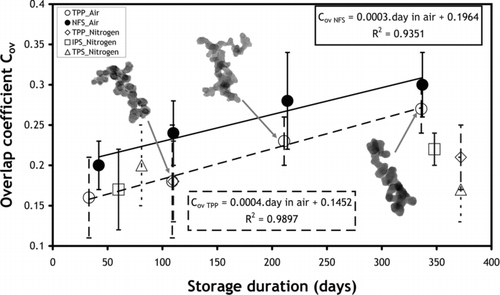
In parallel to the evolution of soot in ambient air, and also present the changes in the morphological parameters as a function of the storage duration in the low humidity, nitrogen filled chamber. Under such inert conditions, the changes observed over time in ambient air are significantly mitigated, demonstrating that humidity, oxygen and others gases present in the atmosphere may have a major influence on the morphology and the inner microstructure of soot particles and monomers. Nevertheless, we should note that for storage under nitrogen, the first analysis was carried out after more than 100 days, so no conclusion can be drawn on any potential evolution of morphological parameters during this first period.
To our knowledge, only a few workers have reported such an evolution of the morphological parameters of soot particles for long duration storage under ambient conditions. Recently, CitationBraun (2009) proposed a general model of ageing of soot in the atmosphere and suggested that, during the first weeks of soot ageing, PAH trapped on primary particles may be released and lost through desorption, decreasing the volume of primary particles. After this first step, CitationBraun (2009) suggested that decomposition of the remainder of the soot particle may occur through chemical reaction with the air and humidity, forming a thin graphitic layer at the surface of the primary particles. This two-step model of desorption followed by oxidation of soot, may explain the initial decrease of the gyration and the primary particle diameters as observed in our study (). Furthermore, CitationPopovitcheva et al. (2008) have described a “swelling” of the microstructure of soot due to water uptake under high relative humidity (more than 80%) and, as a consequence, an increase in the primary particle diameter. This “swelling” phenomenon is also observed in the present work for long storage duration (greater than 100 days) for which the primary particle diameter and the overlap coefficient increase.
5. CONCLUSIONS
The aim of the present work was to investigate the influence of the sampling methods and storage conditions on the morphological parameters (Dpp, Dg, Df, kf, and Cov) of soot produced by a laminar ethylene diffusion flame and sampled using four different devices (TPP, TPS, IPS, NFS).
-
Good agreement has been observed in the values from TPP, TPS, and IPS, which are based on sampling particles directly onto TEM grids. This indicates that the dilution induced upstream of TPS and IPS does not significantly change the morphological parameters of soot.
-
Results obtained from direct sampling of soot onto TEM grids (TPP, TPS and IPS) show good agreement for Dpp, Df, kf, when compared to previous work.
-
These results allow comparison of the reliability of these different procedures for sampling soot particles in different conditions (TPP for sampling in flames and TPS, IPS in more diluted conditions). However, a significant difference in aggregate size distributions was demonstrated, revealing different collection efficiencies.
-
The transfer of soot from a Nuclepore membrane (NFS) to a TEM grid induces a significant overestimate of primary particle diameters by more than 10 nm and a corresponding increase in gyration diameters. This non negligible increase is due to the evaporated graphite film. At the same time, the overlap coefficient also seems to be affected by both the evaporated graphite film and the chloroform dissolution.
-
The effects of storage in air or under inert gas and the effects of storage duration on morphological parameters have also been investigated. Further, the evolution of morphological parameters as a function of storage duration in air was determined for TPP and NFS procedures. With regards to the ageing of particles, a significant increase of the overlap coefficient was observed for ambient conditions, while Dpp only changed slightly. This effect was clearly reduced when particles were kept in a chamber filled with nitrogen, at a relative humidity of 15 % and a mean temperature of 20°C. On the other hand, the method used to measure Df and kf from TEM images does not reveal any significant dependency of the storage conditions on fractal law parameters. The observed soot contraction, due to ageing in ambient air, demonstrates the physical and chemical phenomena that occur under ambient conditions. This could be explained by PAH and transfer of O2 and H2O within the internal structure of the soot, in agreement with several reports in the literature. Nevertheless, further investigations (microstructure characterization by HRTEM and measurement of adsorption/desorption of PAH) must be undertaken in order to describe more precisely the evolution of morphological and structural properties of soot in the atmosphere.
In conclusion, in view of the results of this present work, it can be asserted that it is better to sample soot particles directly onto TEM grids, by thermophoresis or by diffusion or impaction/interception, rather than by filtration onto polycarbonate membranes. The samples must be stored in a cell filled with inert gas at low humidity and in the absence of light. Finally, TEM analysis of samples should be performed as soon as possible and not after more than two months of storage in ambient air. Lastly, it can be concluded that the different protocols are suitable for sampling particles produced by different combustion processes (gaseous burner, aircraft engines, diesel, fire, etc.). Nevertheless, with regards to atmospheric ageing, the present results must be applied with caution to other soot sources especially considering the large variability in physico-chemical composition of soot produced by different processes and conditions. Moreover, since the present work only aimed to determine sampling and storage influences on the morphological parameters, further investigations will now be conducted using high resolution TEM of the microstructure in the inner core and outer shell of soot particles, together with chemical analyses (EDX, FTIR), in order to improve our understanding of soot particle ageing under ambient conditions.
This work has been supported by the ANR program BLAN-06-346 (SOOT). The authors would like to thank M. Maugendre and L. Lucas from CORIA for their assistance during the sampling of soot particles and S. Ducourtieux from LNE for his participation in carbon deposit analysis.
REFERENCES
- Bang , J. J. , Trillo , E. A. and Murr , L. E. 2003 . Utilization of Selected Area Electron Diffraction Patterns for Characterization of Air Submicron Particulate Matter Collected by a Thermophoretic Precipitator. . J. Air Waste Manag. Assoc. , 53 : 227 – 236 .
- Barone , T. L. , Lall , A. A. , Zhu , Y. , Yu , R.-C. and Friedlander , S. K. 2006 . Inertial Deposition of Nanoparticle Chain Aggregates: Theory and Comparison with Impactor Data for Ultrafine Atmospheric Aerosols. . J. Nanoparticle Research. , 8 : 669 – 680 .
- Bau , S. , Witschger , O. , Gensdarmes , F. , Rastoix , O. and Thomas , D. 2010 . A TEM-Based Method as an Alternative to the BET Method for Measuring Off-Line the Specific Surface Area of Nanoaerosols. . Powder Technol. , 200 : 190 – 201 .
- Brasil , A. M. , Farias , T. L. and Carvalho , M. G. 1999 . A Recipe for Image Characterization of Fractal-Like Aggregates. . J. Aerosol Sci. , 30 : 1379 – 1389 .
- Braun , A. 2009 . Two-Process Model for the Atmospheric Weathering, Oxidation and Ageing of Diesel Soot. . Geophys. Res. Lett. , 36 : L07810
- Bushell , G. C. , Yan , Y. D. , Woodfield , D. , Raper , J. and Amal , R. 2002 . On Techniques for the Measurement of the Mass Fractal Dimension of Aggregates. . Adv. Colloid Interface Sci. , 95 : 1 – 50 .
- Cai , J. , Lu , N. and Sorensen , C. M. 1993 . Comparison of Size and Morphology of Soot Aggregates as Determined by Light Scattering and Electron Microscope Analysis. . Langmuir. , 9 : 2861 – 2867 .
- Cai , J. , Lu , N. and Sorensen , C. M. 1995 . Analysis of Fractal Cluster Morphology Parameters: Structural Coefficient and Density Autocorrelation Function Cutoff. . J. Colloid Interface Sci. , 171 : 470 – 473 .
- Chandler , M. F. , Teng , Y. and Köylü , Ü. Ö. 2007 . Diesel Engine Particulate Emissions: A Comparison of Mobility and Microscopy Size Measurements. . Proc. Combust. Inst. , 31 : 2971 – 2979 .
- Chen , Y. , Shah , N. , Huggins , F. E. and Huffman , G. P. 2006 . Microanalysis of Ambient Particles from Lexington, KY, by electron microscopy. . Atmos. Environ. , 40 : 651 – 663 .
- Cheng , C. H. , Lehmann , J. , Thies , J. E. , Burton , S. D. and Engelhard , M. H. 2006 . Oxidation of Black Carbon by Biotic and Abiotic Processes. . Org. Geochem. , 37 : 1477 – 1488 .
- Cheng , C. H. and Lehmann , J. 2009 . Ageing of Black Carbon Along a Temperature Gradient. . Chemosphere. , 75 : 1021 – 1027 .
- Chung , A. , Lall , A. A. and Paulson , S. E. 2008 . Particulate Emissions by a Small Non–Road Diesel Engine: Biodiesel and Diesel Characterization and Mass Measurements Using the Extended Idealized Aggregates Theory. . Atmos. Environ. , 42 : 2129 – 2140 .
- Colbeck , I. , Atkinson , B. and Johar , Y. 1997 . The Morphology and Optical Properties of Soot Produced by Different Fuels. . J. Aerosol Sci. , 28 : 715 – 723 .
- Decesari , S. , Facchini , M. C. , Matta , E. and Mirce , M. 2002 . Water Ssoluble Organic Compounds Formed by Oxidation of Soot. . Atmos. Environ. , 36 : 1827 – 1832 .
- Dobbins , R. A. and Megaridis , C. M. 1987 . Morphology of Flame–Generated Soot as Determined by Thermophoretic Sampling. . Langmuir. , 3 : 254 – 259 .
- Hu , B. , Yang , B. and Köylü , U. O. 2003 . Soot Measurements at the Axis of an Ethylene/Air Non-Premixed Turbulent Jet Flame. . Combust. Flame. , 134 : 93 – 106 .
- Hurt , R. H. , Sarofim , A. F. and Longweel , J. P. 1993 . Gasification-Induced Densification of Carbons: From Soot to Form Coke. . Combust. Flame. , 95 : 430 – 432 .
- Ishiguro , T. , Suzuki , N. , Fujitani , Y. and Morimoto , H. 1991 . Microstructural Changes of Diesel Soot During Oxidation. . Combust. Flame. , 85 : 1 – 6 .
- Ishiguro , T. , Takatori , Y. and Akihama , K. 1997 . Microstructure of Diesel Soot Particles Probed by Electron Microscopy: First Observation of Inner Core and Outer Shell. . Combust. Flame. , 108 : 231 – 234 .
- Jullien , R. and Botet , R. 1987 . Aggregation and Fractal Aggregates , Singapore : World Scientific Publishing Co Pte Ltd .
- Kandas , A. W. , Gokhan Senel , I , Levendis , Y. and Sarofim , A. F. 2005 . Soot Surface Area Evolution During Air Oxidation as Evaluated by Small Angle X–Ray Scattering and CO2 Adsorption. . Carbon. , 43 : 241 – 251 .
- Kim , H. W. and Choi , M. 2003 . In Situ Line Measurement of Mean Aggregates Size and Fractal Dimension Along the Flame Axis by Planar Light Scattering. . J. Aerosol Sci. , 34 : 1633 – 1645 .
- Kim , D. , Kumfer , B. M. , Anastasio , C. , Kennedy , I. M. and Young , T. M. 2009 . Environmental Aging of Polycyclic Aromatic Hydrocarbons on Soot and its Effect on Source Identification. . Chemosphere. , 76 : 1075 – 1081 .
- Kis , V. , Posfai , M. and Labar , J. 2006 . Nanostructure of Atmospheric Soot Particle. . Atmos. Environ. , 40 : 5533 – 5542 .
- Kittelson , D. B. 1998 . Engines and Nanoparticles: A Review. . J. Aerosol Sci. , 29 : 575 – 588 .
- Köylü , Ü. Ö. and Faeth , G. M. 1992 . Structure of Overfire Soot in Buoyant Turbulent Diffusion Flames at Long Residence Times. . Combust. Flame. , 89 : 140 – 152 .
- Köylü , Ü. Ö. , Faeth , G. M. , Farias , T. L. and Carvalho , M. G. 1995 . Fractal and Projected Structure Properties of Soot Aggregates. . Combust. Flame. , 100 : 621 – 633 .
- Köylü , U. O. , McEnally , C. S. , Rosner , D. E. and Pfefferle , L. D. 1997 . Simultaneous Measurements of Soot Volume Fraction and Particle Size/Microstructure in Flames Using a Thermophoretic Sampling Techniques. . Combust. Flame. , 110 : 494 – 507 .
- Lee , J. , Altman , I. and Choi , M. 2008 . Design of Thermophoretic Probe for Precise Particle Sampling. . J. Aerosol Sci. , 39 : 418 – 431 .
- Lee , C. and Kramer , T. A. 2004 . Prediction of Three-Dimensional Fractal Dimensions Using the Two-Dimensional Properties of Fractal Aggregates. . Adv. Colloid Interface Sci. , 112 : 49 – 57 .
- Lemaire , R. , Maugendre , M. , Schuller , T. , Therssen , E. and Yon , J. 2009 . Original Use of a Direct Injection High Efficiency Nebulizer for the Standardization of Liquid Fuels Spray Flames. . Rev. Sci. Instrum. , 80 : 105105
- Mandelbrot , B. 1973 . Les Objets Fractals: Forme, Hasard et Dimension , Paris : Flammarion .
- Matti Maricq , M. , Harris , S. J. and Szente , J. J. 2003 . Soot Size Distributions in Rich Premixed Ethylene Flames. . Combust. Flame. , 132 : 328 – 342 .
- Medalia , A. I. and Heckman , F. A. 1969 . Morphology of aggregates—II. Size and Shape Factors or Carbon Black Aggregates from Electron Microscopy. . Carbon. , 7 : 567 – 582 .
- Megaridis , C. M. and Dobbins , R. A. 1990 . Morphological Description of Flame-Generated Materials. . Combust. Sci. Technol. , 71 : 95 – 109 .
- Mikkanen , P. , Lamminen , E. , Ojanen , J. and Niemelä , V. 2002 . “ Sample Conditioning with Dilution and/or Adsorption of Volatile Species in Automotive Exhaust. ” . In 6th International Aerosol Conference Taipei, Taiwan
- Mocho , V. M. and Ouf , F. X. 2010 . “ Clogging of Industrial Pleated High Efficiency Particulate Air (HEPA) Filters in the Event of Fire ” . In Nucl. Eng. Des.
- Neimark , A. V. , Köylü , Ü. Ö. and Roesner , D. E. 1996 . Extended Characterization of Combustion Generated Aggregates: Self-Affinity and Lacunarities. . J. Colloid Interface Sci. , 180 : 590 – 597 .
- Oh , C. and Sorensen , C. M. 1997 . The Effect of Overlap Between Monomers on the Determination of Fractal Cluster Morphology. . J. Colloid Interface Sci. , 193 : 17 – 25 .
- Ouf , F. X. , Vendel , J. , Coppalle , A. , Weill , M. and Yon , J. 2008 . Characterization of Soot Particles in the Plumes of Over-Ventilated Diffusion Flames. . Combust. Sci. Technol. , 180 : 674 – 698 .
- Palotás , A. B. , Rainey , L. C. , Sarofim , A. F. , Vander Sande , J. B. and Ciambelli , P. 1996 . Effect of Oxidation on the Microstructure of Carbon Blacks. . Energy Fuels. , 10 : 254 – 259 .
- Park , K. , Kittelson , D. B. , Zachariah , M. R. and McMurry , P. H. 2004 . Structural Properties of Diesel Exhaust Particles Measured by Transmission Electron Microscopy (TEM): Relationships to Particle Mass and Mobility. . Aerosol Sci. Technol. , 38 : 881 – 889 .
- Petzold , A. , Döpelheuer , A. , Brock , C. A. and Schröder , F. 1999 . In Situ Observations and Model Calculations of Black Carbon Emission by Aircraft at Cruise Altitude. . J. Geophys. Res. , 104 : 171 – 181 .
- Popovitcheva , O. B. , Periantseva , N. M. , Trukhui , M. E. , Rulev , G. B. , Shonija , N. K. , Ya Buriko , Y. , Starik , A. M. , Demirdjan , B. , Ferry , D. and Suzanne , J. 2000 . Experimental Characterization of Aircraft Combustor Soot: Microstructure, Surface Area, Porosity and Water Adsorption. . Phys. Chem. Chem. Phys. , 2 : 4421 – 4426 .
- Popovitcheva , O. B. , Periantseva , N. M. , Shonija , N. K. , DeMott , P. , Koehler , K. , Petters , M. , Kreidenweis , S. , Tishkova , V. , Demirdjan , B. , Ferry , D. and Suzanne , J. 2008 . Water Interaction with Hydrophobic and Hydrophilic Soot Particles. . Phys. Chem. Chem. Phys. , 10 : 2332 – 2344 .
- Rasband , W. S. 1997–2009 . ImageJ, U. S. National Institutes of Health, Bethesda, Maryland, USA, http://rsb.info.nih.gov/ij/
- Rogak , S. N. , Flagan , R. C. and Nguyen , H. V. 1993 . The Mobility and Structure of Aerosol Agglomerates. . A. Sci. Technol. , 18 : 25 – 47 .
- Sachdeva , K. and Attri , A. K. 2008 . Morphological Characterization of Carbonaceous Aggregates in Soot and Free Fall Aerosol Samples. . Atmos. Environ. , 42 : 1025 – 1034 .
- Samson , R. J. , Mulholland , G. W. and Gentry , J. W. 1987 . Structural Analysis of Soot Agglomerates. . Langmuir. , 3 : 272 – 281 .
- Shaddix , C. , Palotas , A. , Megaridis , C. , Choi , M. and Yang , N. 2005 . Soot Graphitic Order in Laminar Diffusion Flames and a Large-Scale JP-8 Pool Fire. . Int. J. Heat Mass Transfer. , 48 : 3604 – 3614 .
- Sorensen , C. M. , Cai , J. and Lu , N. 1992 . Light-Scattering Measurements of Monomer Size, Monomers Per Aggregate, and Fractal Dimension for Soot Aggregates in Flames. . Appl. Opt. , 31 : 6547 – 6557 .
- Sorensen , C. M. and Feke , G. D. 1996 . The Morphology of Macroscopic Soot. . A. Sci. Technol. , 25 : 328 – 337 .
- Sorensen , C. M. and Roberts , G. 1997 . The Prefactor of Fractal Aggregates. . J. Colloid Interface Sci. , 186 : 447 – 452 .
- Sorensen , C. M. 2001 . Light Scattering by Fractal Aggregates: A Review. . A. Sci. Technol. , 35 : 648 – 687 .
- Spurny , K. R. 1986 . Physical and Chemical Characterization of Individual Airborne Particles , 40 – 71 . New York : John Wiley and Sons .
- Teng , Y. , Köylü , Ü. Ö. , Hagen , D. E. and Whitefield , P. D. 2009 . Performance of the Mobility Sizing Technique Relative to Independent Diagnostics for the Characterization of Polydisperse Soot Aggregates. . Combust. Sci. Technol. , 181 : 1526 – 1548 .
- Thomassen , Y. , Koch , W. , Dunkhorst , W. , Ellingsen , D. G. , Skaugset , N.-P. , Jordbekken , L. , Drablos , P. A. and Weinbruch , S. 2006 . Ultrafine Particles at Workplaces of a Primary Aluminium Smelter. . J. Environ. Monit. , 8 : 127 – 133 .
- Tian , K. , Liu , F. , Thomson , K. A. , Snelling , D. , Smallwood , G. J. and Wang , D. 2004 . Distribution of the Number of Primary Particles of Soot Aggregates in a Nonpremixed Laminar Flame. . Combust. Flame. , 138 : 195 – 198 .
- Tian , K. , Thomson , K. A. , Liu , F. , Snelling , D. R. , Smallwood , G. J. and Wang , D. 2006 . Determination of the Morphology of Soot Aggregates Using the Relative Optical Density Method for the Analysis of TEM Images. . Combust. Flame. , 144 : 782 – 791 .
- Van Hulle , P. 2002 . “ Caractérisation des aérosols émis par la combustion des hydrocarbures: Application à la mesure de l'indice de réfraction des suies ” . In PhD Thesis , France : University of Rouen .
- Vander , Wal R. 1997 . A TEM Methodology for the Study of Soot Particle Structure. . Combust. Sci. Technol. , 126 : 333 – 357 .
- Wen , J. and Wexler , A. S. 2007 . Thermophoretic Sampler and its Application in Ultrafine Particle Collection. . A. Sci. Technol. , 41 : 624 – 629 .
- Wentzel , M. , Gorzawski , H. , Naumann , K.-H. , Saathoff , H. and Weinbruch , S. 2003 . Transmission Electron Microsopical and Aerosol Dynamical Characterization of Soot Aerosols. . J. Aerosol Sci. , 34 : 1347 – 1370 .
- Witten , T. A. and Sander , C. M. 1981 . Diffusion Limited Aaggregation, a Kinetic Critical Phenomenon. . Phys. Rev. Lett. , 47 : 1400 – 1403 .
- Xiong , C. and Friedlander , S. K. 2001 . Morphological Properties of Atmospheric Aerosol Aggregates. . Proc. Nat. Acad. Sci. U. S. A. , 98 : 11851 – 11856 .
- Yezerets , A. , Currier , N. W. , Eadler , H. A. , Suresh , A. , Madden , P. F. and Branigin , M. A. 2003 . Investigation of the Oxidation Behaviour of Diesel Particulate Matter. . Catal. Today. , 88 : 17 – 25 .
- Zhao , B. , Yang , Z. , Johnston , M. V. , Wang , H. , Wexler , A. , Balthasar , M. and Kraft , M. 2003 . Measurement and Numerical Simulation of Soot Particle Size Distribution Functions in a Laminar Premixed Ethylene-Oxygen-Argon Flame. . Combust. Flame. , 133 : 173 – 188 .
- Zhu , J. , Choi , M. Y. , Mulholland , G. W. and Gritzo , L. A. 2000 . Measurement of Soot Optical Properties in the Near-Infrared Spectrum. . Int. J. Heat Mass Transfer. , 43 : 3299 – 3303 .