Abstract
Condensational growth is not typically assumed to be significant compared with adsorption for conversion of unburned hydrocarbons in the exhaust of diesel engines to the particulate phase. However, when partially premixed low temperature combustion (LTC) modes designed to simultaneously reduce soot and NO X emissions are implemented, unburned hydrocarbon (UHC) concentrations in the exhaust are an order of magnitude higher than for conventional combustion modes, increasing the likelihood of gas to particle conversion by condensation. In this work, two LTC operating conditions are compared with conventional diesel combustion using a multi-cylinder direct-injection diesel engine using low-sulfur fuel, a soy-based biodiesel and a 50% by volume biodiesel blend. Gaseous emissions of unburned hydrocarbons were measured and particulate samples were taken using a partial-flow dilution tunnel. Gravimetric analysis of the collected filters, Soxhlet extraction of particulate and speciation using GC-FID was performed for all operating conditions. Elemental carbon (EC) emissions were measured using a thermal optical analyzer and particle size distribution was analyzed using a differential mobility spectrometer. For increasing biodiesel concentration in the fuel, mass emissions of both EC and UHC decreased for all combustion modes compared with petroleum diesel. However, for biodiesel use in LTC modes of operation, particulate mass significantly increased following exhaust dilution. Low vapor pressure methyl esters found in the exhaust of biodiesel LTC increases heterogeneous condensation onto soot particles in the exhaust compared with unburned species from petroleum diesel fuel operation. A model estimating this condensation mechanism accurately predicts the experimental findings of increased mass of particulate for biodiesel operation.
1. INTRODUCTION
Particulate from diesel engines is primarily composed of carbonaceous soot formed in high temperature, fuel-rich zones of combustion, and organic matter attached to the soot particles along with contributions from sulfates, nitrates, and ash. Following combustion, gas to particle conversion of residual unburned fuel, lubricating oil, sulfur components or partially burned species occurs during cooling of the raw effluent through the exhaust system of the engine and upon dilution and quenching from ambient air as it enters the environment. Adsorption and condensation are mostly responsible for the growth of diesel particulate following dilution with adsorption commonly thought to be the primary mechanism for conventional combustion modes using petroleum diesel fuel (CitationPlee and MacDonald 1980). Condensation is a function of the vapor pressure and concentration of the contributing species whereas adsorption relates to surface bonding of species and is not a direct function of volatility.
The use of biodiesel, a blend of methyl esters resulting from transesterification of plant and animal-derived triglycerides, is well known to significantly reduce soot formation as well as the emissions of hydrocarbons and CO as is reviewed by CitationLapuerta et al. (2008) and others. Reductions in soot have been shown to be proportional to the quantity of oxygen in the fuel (González et al. 2001) with near zero emissions if 30–40% of the fuel is oxygen (CitationMiyamoto et al. 1998). Although overall PM mass has also been shown to decrease for biodiesel use in diesel engines utilizing conventional combustion strategies, organic fraction is well known to be higher for biodiesel than for petroleum diesel fuels especially at part load conditions (CitationDi et al. 2009; Lapuerta et al. 2007; CitationBagley 1998; CitationChang and VanGerpen 1998).
Partially premixed low temperature combustion (LTC) is a diesel engine operational strategy that defeats the well-known soot versus NOX tradeoff found in conventional combustion modes by simultaneously lowering combustion temperature and reducing local equivalence ratios. LTC is typically enabled in modern diesel engines by increasing exhaust gas recirculation (EGR) such that the time from injection of fuel to ignition is extended thus allowing extra time for pre-mixing (CitationJacobs et al. 2007). Such modes of operation are practical for use in low to moderate engine speeds and loads. Increased EGR also raises the specific heat of the gases in the cylinder, lowering peak combustion temperatures thus avoiding the formation of NO by thermal mechanisms. One downside of LTC operation is the over tenfold increase in unburned hydrocarbon (UHC) emissions caused by unreacted fuel escaping from over-lean areas of the cylinder as compared to conventional diesel combustion (CitationCook et al. 2008). Although soot from LTC modes is very low compared to conventional combustion, it is still formed as a result of high temperature fuel-rich mixtures in recirculation zones created by the vaporized fuel plume coming into contact with the piston bowl (Genzale et al. 2008).
The use of biodiesel and LTC together has shown to have a compounding benefit by lowering soot and UHC emissions at the same time (CitationZheng et al. 2008). However, little published work examines the particulate emissions resulting from the combination. It has been shown that particle size distribution for biodiesel LTC tends towards more accumulation mode particles compared to petroleum diesel (CitationNorthrop et al. 2009) indicating that some additional PM growth may occur due to the high levels of UHC in LTC operation converting from the gas to the particle phase. The work presented here explores this hypothesis by comparing the soot and PM emissions from two LTC modes as well as from conventional combustion. Semi-volatile, unburned fuel on the particles is identified using GC methods and a model is proposed to predict the contribution of heterogeneous condensation to PM mass.
2. EXPERIMENTAL
2.1. Test Engine and Fuels
Experimental testing was conducted using a multi-cylinder direct injection diesel engine suitable for use in a mid-sized passenger car. Specifications of the test engine are given in . The compression ratio of the engine was lowered from the stock engine configuration to enable LTC over a larger range of operation as described in CitationJacobs et al. (2005). Load and speed were controlled by a direct current dynamometer and the engine was equipped with a programmable electronic control unit (ECU) to allow management of parameters like EGR, fuel injection timing, injection pressure and duration. Real-time combustion monitoring was accomplished using a Redline CAS II data acquisition system manufactured by A&D Technology Inc. with Kistler 6041A piezo-electronic pressure transducers. Pressure data from cylinder number one were processed real-time by the internal combustion analysis software provided in the high speed system to determine combustion phasing information.
TABLE 1 Specifications of the test engine
Fuels tested included U.S. specification ultra low sulfur diesel (ULSD), 100% soy-based fatty acid methyl ester biodiesel (B100) and 50% by volume blend of ULSD mixed with biodiesel (B50). Physical and ignition properties of the three tested fuels are shown in . The biodiesel was used within three months of transesterification to avoid extensive fuel oxidation and exceeded the standards defined by ASTM 6751 at the time of the engine testing.
TABLE 2 Fuel properties
2.2. Operating Conditions and Test Procedure
Three steady state operating conditions were tested, each with the three fuels. The first was a conventional combustion condition utilizing pilot and main injections set in accordance with the stock calibration of the engine ECU. The second operating condition was a late injection LTC condition (LLTC) utilizing a single injection near to TDC but with elevated levels of EGR to sufficiently extend ignition delay. The third operating condition chosen was an early injection LTC strategy (ELTC). This mode, typically used a low engine loads, utilized an advanced single injection strategy and elevated levels of EGR to maximize premixing of fuel and air prior to combustion.
Control parameters set constant over the fuel sweep for a given operating condition included speed, load, EGR rate, injection pressure, intake manifold temperature, intake manifold pressure, and the crank angle degree of 50% mass fraction burned (CA50). It has been shown that by setting CA50 to maintain constant combustion phasing, differences in ignition quality can be normalized thus revealing fairer comparisons of emissions from fuels of varying chemical properties (CitationIckes et al. 2009). In the test procedure, an operating condition was set using the baseline ULSD fuel. For a new fuel at the same condition, injection timing was altered to maintain the same CA50, thus normalizing for differences in fuel ignition quality. For the conventional dual injection strategy where two injections were used, the dwell between injections was set constant as the timing was changed. shows the chosen constant engine parameters for the experimental testing with the 95% confidence interval error calculated from the standard deviation of all data taken over the fuel sweep for each operating condition.
TABLE 3 Engine parameters held constant for each operating condition
2.3. Emissions Measurement and Analysis
Gaseous UHC is defined here to represent semi-volatile species measured in an undiluted heated sample line held to 190°C. A flame ionization detector (FID) was used to measure total hydrocarbon (THC) concentration and a Fourier Transform Infrared (FT-IR) analyzer was used to measure primary non-fuel hydrocarbon species resulting from incomplete combustion in the engine. The UHC was calculated as the difference between the THC measurement and the sum of the non-fuel species detected with the FT-IR on a C1 basis. It has been shown previously and is assumed in this work that the bulk of gas phase UHC consists of unburned fuel escaping the combustion process (CitationHan et al. 2009).
Particulate was collected from the engine using a Sierra Instruments BG-2 partial flow dilution tunnel equipped with a 10 μm cyclone separator and 39 mm diameter filter holder implemented in accordance with ISO/DIS 16183. A dilution ratio of 10:1 and filter temperature of 47 ± 5°C were used for all filter sampling. For gravimetric analysis, Whatman 7592 ring-supported PTFE membrane filters with 2 μm pore size were used. For each data point, three filters were collected for gravimetric analysis. Conditioning and weighing of filters was done in accordance with EPA 40 CFR 1065 using a Mettler Toledo XP6U microbalance. Particulate was also loaded on quartz filters and organic versus elemental carbon (OCEC) analysis was performed using a thermal optical analyzer manufactured by Sunset Analytical. The analysis was only used to detect the elemental carbon (EC) mass on the quartz filters since proper post-conditioning of quartz filters was not possible at the time of testing. The EC measurement using this method was used to report the carbonaceous soot fraction of the particulate emissions. The methods used for the OCEC analysis were in accordance with NIOSH 5040 and those described in CitationBirch et al. (1996).
A DMS500 differential mobility spectrometer (DMS) manufactured by Cambustion Inc. was used for measuring PM size distribution. The DMS allowed real-time sampling of particle size and utilized an internal mass dilution ratio of air to exhaust gas of 5:1, the maximum allowable by the instrument. When taking samples from the DMS, 30 s of data were acquired at a sampling rate of approximately 10 Hz and consequently averaged per engine condition.
Soluble organic matter was extracted from the particulate filters using the Soxhlet extraction method. Dichloromethane (DCM) was used as the sole solvent as it has been shown to be effective in the removal of methyl ester compounds as well as species found in petroleum diesel fuel (CitationBagley et al. 1998). Extractions of three filters were run in tandem at a rate of 6 min per cycle for 15 h to yield approximately 150 total cycles. A known mass of three internal standard species were injected into the solvent prior to extraction for use in later GC-FID speciation. N-tetradecane was used for identification of hydrocarbons from C8 to C14, n-nonadecane was used to identify fuel and oil hydrocarbons from C15 to C30 and methyl-heptadecanoate (C17:0) was used in the identification of methyl ester species from biodiesel.
The goal of the GC-FID analysis was the identification of unburned petroleum diesel fuel and biodiesel species in the PM. A Shimadzu GC-17A GC with FID was implemented using a pre-set temperature program at constant pressure. Four categories of species were identified: individual n-alkanes typically found in diesel fuel, unidentified light hydrocarbon molecules, methyl ester compounds from biodiesel and heavy hydrocarbon species assumed to be mostly from the engine lubricating oil. Quantitative analysis was performed using the well known response factor method (CitationMcNair and Miller 1997) where the factors for n-alkane species from diesel fuel were estimated using the methods of CitationScanlon and Willis (1985) and methyl ester species factors were from CitationUlberth et al. (1999). The responses for other species not identified were assumed to be unity.
2.4. Uncertainty
Uncertainty was estimated in the experimental results by considering both systemic and repeatability errors. Error bars reported in this article are a result of the root-sum-square of the uncertainties associated with a given variable reported on a 95% confidence interval basis. Repeatability uncertainty for gaseous emissions and engine performance was calculated from the variability of 50 points of sampling per data point over three repeated tests at the same engine operating condition. Repeatability for PM gravimetric data was established based on twice the standard deviation of three weighed filters per engine condition combined with repeated tests of the same condition. Propagation of error for calculated parameters such as brake specific emissions was estimated using the method of sequential perturbation (Figliola and Beasley 2006).
3. RESULTS AND DISCUSSION
3.1. Experimental Study
LTC modes of diesel engine operation have considerably higher emissions of UHC, assumed to consist mostly of unburned fuel, than conventional combustion due to large pockets of fuel and air mixture leaner than flammability limits. Such semi-volatile species escape the combustion process in the gas phase and have the potential to form particulate once cooled in the atmosphere. For the tested LLTC and ELTC conditions, UHC was greater than for conventional diesel combustion as illustrated in . ELTC had the highest UHC emissions due to the increased mixing of fuel and air caused by early injection of fuel. Extending ignition delay in this way contributes to more over-lean regions of the combustion chamber thus increasing the amount of injected fuel species outside lean flammability limits.
FIG. 1 Mass emissions of gaseous UHC on a C1 basis as measured in a 190°C sample line from FID and FT-IR as a function of biodiesel fraction in the fuel.
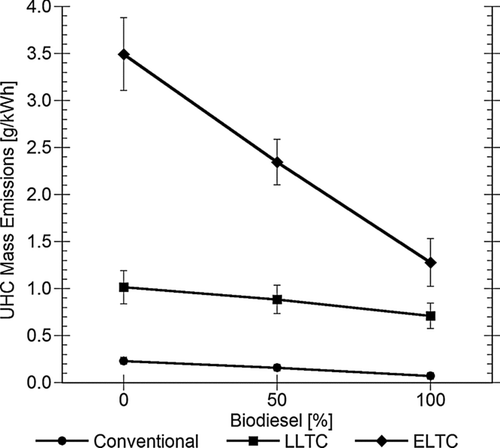
Emissions of UHC decreased with increasing biodiesel concentration in the fuel for a given operating condition which can be attributed mostly to the presence of fuel-borne oxygen contributing to oxidative processes both during combustion and in the expansion stroke. Among fuels for a given engine condition, the ignition delay was decreased with increasing biodiesel concentration since the B100 had higher cetane number than ULSD. This required advancing the injection timing for the biodiesel fuels to maintain a constant CA50. Shortening ignition delay also had the effect of lowering UHC emissions for increasing biodiesel due to reduced time allowed for over-mixing of fuel and air to occur.
As is characteristic of LTC operation, the mass emissions of EC were much lower for ELTC and LLTC than for conventional combustion as shown in . ELTC had lower combustion temperatures and leaner global equivalence ratio contributing to near zero EC emissions for the three fuels tested. For conventional diesel combustion, increasing the concentration of biodiesel in the fuel resulted in a marked decrease in EC emissions consistent with the findings of CitationBennett et al. (2008) among others, whereas reductions were less significant for the LTC modes. The combination of biodiesel and LTC has significant benefit for compounding reductions in EC emissions for the LLTC mode as illustrated in the figure.
FIG. 2 Mass emissions of PM from gravimetric analysis and EC from OCEC measurement as a function of biodiesel fraction in the fuel.
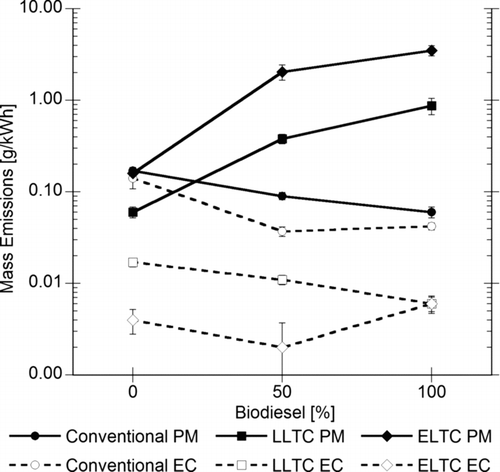
The mass emissions of PM decreases with EC as biodiesel content increases for conventional combustion as given in . This finding is in alignment with the established literature where it has been clearly shown that although the organic fraction of PM increases with increasing biodiesel, the decrease in EC emitted is dominant, thus lowering PM mass (CitationWu et al. 2009; CitationLapuerta et al. 2000; Rantanen et al. 1993). However, for ELTC and LLTC cases, the organic fraction is clearly more significant. Although EC mass emissions decreased for LLTC, the PM mass increased by almost tenfold from 0 to 100% biodiesel. The ELTC condition, with nearly negligible soot mass over the fuel range, had two orders of magnitude higher PM than EC emissions indicating a massive contribution of semi-volatile species. Two results are evident from the comparison of EC and PM mass emissions from the three engine operational modes. First, although LTC modes of combustion have very low EC emissions due to the premixed combustion process, the higher levels of gaseous UHC significantly contributes to PM mass after dilution. Second, as the content of biodiesel in the fuel increases, EC formation is reduced due to concurrent oxidative processes during combustion but PM mass is drastically increased indicating that the organic fraction becomes dominant.
Particle size data taken with the DMS further confirm the PM mass findings. shows that for conventional diesel combustion, a bi-modal distribution exists where nucleation mode particles remain the same but agglomeration mode particles decrease with increasing biodiesel content. Jung et al. (2006) found that particles from biodiesel combustion have greater reactivity for oxidation leading to smaller accumulation mode particles. The finding of near constant nucleation mode particles however is inconsistent with Jung et al. (2006) as well as others (CitationAakko et al. 2003; CitationDi et al. 2009) where particles with diameters lower than 50 nm have been shown to increase for biodiesel operation. A possible reason for this disparity is that most published experimental studies set constant injection timing when testing fuels of varying cetane number whereas combustion phasing was held constant in the work reported here. Normalizing for fuel ignition quality by adjusting injection timing between fuels may have had the effect of equalizing formation of nucleated particles during combustion of the fuels tested.
FIG. 3 Particle size distribution, by number, taken by DMS for: (a) Conventional operating condition, (b) LLTC, and (c) ELTC.
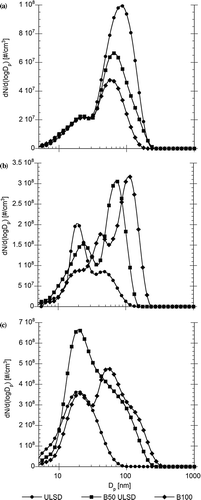
For LTC operation, the trends are again reversed as compared to conventional combustion as given in Figures and . Agglomeration mode particles increased in both size and number with increasing biodiesel content in the fuel for the LLTC case. Particles continued to increase and grow for the ELTC condition for both the B50 and B100 cases. Growth of agglomerated particles corresponds well to the increased PM mass data found from the gravimetric analysis and is assumed to be the result of the increased organic fraction as biodiesel concentration increases in the fuel. The number of nucleation mode particles for LTC increased compared with conventional combustion though biodiesel content in the fuel resulted in no clear trends for the smallest particles.
The total number of particles and the geometric mean particle diameter (GMD) for the distributions shown in provides a good overall view of the particle statistics resulting from the three modes of combustion. As illustrated in , the total number of particles is lowest for conventional combustion where the overall number of particles decreases slightly with increasing biodiesel, consistent with the findings of CitationLapuerta et al. (2003). For conventional diesel combustion, shows that the GMD decreases with increasing biodiesel, a similar finding to that from Heikkela et al. (2009). For LLTC and ELTC the trends are again opposite. LLTC has higher total particle count than conventional and the GMD increases considerably as biodiesel content increases. ELTC shows the same trends with increasing biodiesel with the total number of particles increasing threefold from 0 to 100% biodiesel fuel. From the particle size statistics, it is clear that for conventional diesel combustion, biodiesel has advantages in terms of lowering both PM mass and number of agglomeration mode particles. For LTC combustion however, agglomeration mode particles increase both in size and number with higher biodiesel concentration indicating a larger semi-volatile fraction.
FIG. 4 Particle statistics from DMS data for three modes of operation as a function of biodiesel content in the fuel (a) total number of particles, and (b) geometric mean particle diameter.
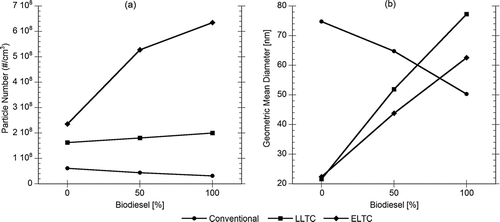
To identify the primary semi-volatile species that contribute to the increase in PM mass for biodiesel LTC, speciation of both the ULSD and B100 fuels was conducted using a GC-FID method. Raw data signals were converted to peak area distributions for identification. Eleven n-alkane species were identified in a ULSD sample as shown in and five methyl esters were found in a biodiesel fuel sample as illustrated in . From the extracted PM, shows that for conventional combustion, heavy hydrocarbons from the lubricating oil and non-n-alkane light hydrocarbons made up the majority of extracted species. With increasing biodiesel, raw unburned fuel contributed more to the extracted organics confirming published findings of increased soluble organic fraction for biodiesel PM. For LLTC and ELTC as shown in Figures and c, unburned methyl esters from biodiesel dominated the extracted species as biodiesel content in the fuel increased. The results of the PM extraction leads to the conclusion that the increase in PM mass and particle number and size with increasing biodiesel for LTC modes of combustion is due to high concentrations of gaseous unburned methyl esters in the raw exhaust converting to the particle phase after dilution.
3.2. Condensation Model
Adsorption and condensation are the primary gas to particle conversion mechanisms thought to be responsible for PM growth from diesel engines. In a comprehensive study of PM sampling from diesel engines, CitationPlee and MacDonald (1980) explored the effects of dilution ratio and collection temperature on PM mass and composition. By comparing experimental data to simple models, they found that adsorption was the primary mechanism responsible for conversion of semi-volatile organics to the particle phase. Confirming this finding, CitationClerc and Johnson (1982) developed a more complex model for predicting the rate of adsorption onto PM prior to a dilution process.
Adsorption, while generally thought to be the dominant mechanism for conventional diesel modes with less than 50 ppm THC in the raw exhaust, is not considered to be responsible for the increases in organic particulate seen for biodiesel LTC. First, although the number of particles nearly tripled for the ELTC condition from 0 to 100% biodiesel as shown in , the number of sites for adsorption may be assumed to be linearly related to this increase and does not explain the greater than one order of magnitude increase in PM mass. Secondly, the methyl esters found in biodiesel have lower volatility than any species found in ULSD. This factor is not accounted for with adsorption since it is largely independent of species vapor pressure. Further, with adsorption, the soot particle sites cannot be assumed to have a much higher preference for biodiesel over petroleum diesel components leading to such large differences in soot mass for the same combustion condition. Lastly, although Plee and MacDonald found that adsorption best fit the trends in dilution ratio and temperature for their combustion condition, their modeling indicated that with increasing concentrations of semi-volatile species in the raw exhaust, like in LTC, condensation plays an increasingly important role.
Condensation is a logical explanation for the increase in PM mass found in the experimental results. This mechanism leads to both the heterogeneous nucleation of nano-sized droplets as well as the heterogeneous growth of existing particles. Based on the reported particle size distribution data, the increased mass of PM for biodiesel LTC was assumed to be mostly due to condensational growth of existing particles. Increasing concentration of low volatility methyl esters for LTC along with increasing mean particle size for biodiesel implies that these species are preferentially condensing over petroleum diesel. Confirming this idea, Durán et al. (2006) found that the adsorption mechanism was inadequate for completely explaining organic content on PM when biodiesel is used in conventional diesel combustion even with relatively lower THC concentrations as compared to this study.
A numerical model was developed which follows the enhanced condensational growth estimation given by Jacobson and Kittelson et al. (2005). An equation was developed by the authors expressing the rate of liquid concentration growth of a species on the surface of a particle as a function of the saturated vapor concentration, and the concentration of each species in the gas phase.
Equation (Equation3) is also an expression of Raoult's law which states that the saturation vapor pressure of a solute in solution is equivalent to the product of the saturation vapor pressure of the solute alone and its mole fraction in solution. Using Raoult's law allows for the interaction of multiple species in determining thermodynamic equilibrium of a two phase system. The set of non-linear equations given in Equation (Equation3) were solved together with mass conservation for a given number of species using the fsolve function in Matlab.
In the model, UHC in the exhaust was approximated by simplified raw fuel compositions. Sixteen species were considered; the four primary methyl esters in proportion to that found from the speciation of raw biodiesel shown from , C9-C19 n-alkanes in proportion to those from for petroleum diesel fuel, and water. Other semi-volatiles like olefins and aromatics contained in the diesel fuel were not considered to simplify the computations. The saturated vapor pressure for each species was calculated using the Antoine equation with coefficients for methyl esters given by CitationYuan et al. (2005). Other physical properties of methyl esters were taken from CitationYuan et al. (2003). The Antoine coefficients for n-alkanes were from Yaws’ Handbook (2003).
For a given condition, UHC concentration was divided by the dilution ratio and was assumed to be in proportion to the fuel used. For example, for B50 (50% biodiesel) the UHC was assumed to contain 50% by volume biodiesel and 50% of the n-alkane mixture. For the model, the Kelvin diameter (d*) was assumed to be 50 nm corresponding to the GMD range shown in . The experimental filter collection temperature of 45°C was used and the dilution ratio was assumed to be 10:1 as in the experimental testing. shows the results of the model for a sweep of UHC gas concentrations from 10 to 1000 ppm on a log scale for a range of concentrations of biodiesel. In the figure, results are plotted in terms of the mass fraction of fuel mass originally in the gas phase condensing into particulate. The fraction of fuel condensed for B0 (0% biodiesel) at the conditions shown is negligible up to 1000 ppm of total UHC. For increasing biodiesel in the fuel however, the fraction of UHC that condenses increases, asymptotically approaching complete conversion to the particle phase as UHC increases. For B100, nearly all of the biodiesel comprising the UHC condenses at 1000 ppm of UHC. Based on these results, the model predicts that methyl esters preferentially condense over n-alkanes from petroleum diesel fuels found in exhaust according to the proportion of biodiesel in the fuel.
FIG. 7 Fuel mass fraction condensed versus concentration of UHC in the exhaust for various biodiesel concentrations as estimated by the condensation model.
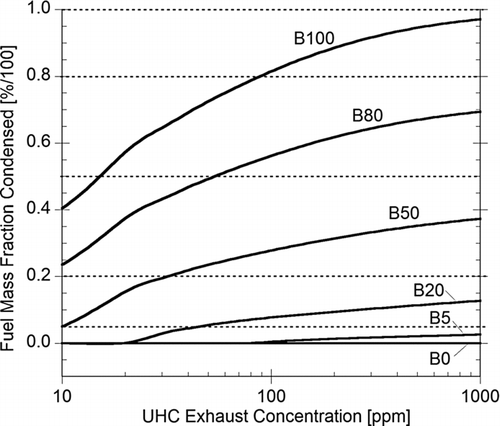
To validate the model using experimental results, the mass of condensed UHC on PM is shown in versus the UHC concentration in the raw exhaust for both the model and all recorded experimental data points. The experimental condensed mass was assumed to be the difference between the total mass of PM and the mass of EC. From the figure, it is apparent that the condensation model accurately predicts the increase in PM mass found in the experimental results both in trend and magnitude when biodiesel is used in combustion modes with high UHC emissions like LTC. If raw exhaust concentrations of UHC are below 100 ppm as in conventional modes of diesel combustion, little difference in condensed species between fuels is apparent. This corresponds well to both the findings of CitationPlee and MacDonald (1980) where condensation was assumed to be negligible compared with adsorption. It also confirms most published findings of similar or lower PM mass for biodiesel and petroleum diesel for conventional combustion.
FIG. 8 Condensed mass as estimated by condensation model compared to experimental results for three fuels and three operating conditions combined.
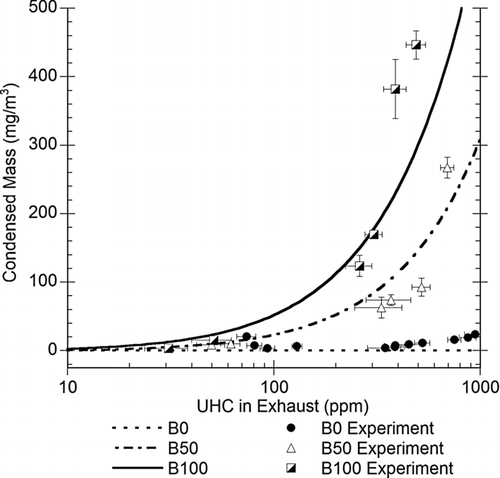
In actual vehicle applications, dilution ratio increases and temperature of the exhaust decreases as the exhaust plume disperses from the tailpipe over time. The model developed in this work was used to examine these effects and the results are shown in where the fuel mass condensed based is plotted versus UHC in the exhaust for B100 fuel at 45°C and various dilution ratios and at 100:1 dilution for various temperatures. The model predicts that no condensation occurs at UHC gas concentrations of less than 40 ppm for a dilution ratio of 100:1 as seen in . For a 1000:1 dilution ratio, no condensation occurs below 400 ppm of UHC which suggests that PM mass would decrease over time as the exhaust becomes more dilute. However, decreasing dilution temperature has a counteracting effect on condensation. shows that for a constant dilution ratio of 100:1 and temperature of 40°C, fuel species begin to condense at a gas phase UHC concentration of 10 ppm. As the temperature decreases to 15°C, 40% of the UHC is converted to the particle phase at the same UHC concentration. Based on these results, it is estimated that condensation of biodiesel species on the PM will continue as the exhaust plume disperses into the environment.
FIG. 9 Fuel mass fraction condensed versus concentration of UHC in the exhaust as calculated by condesation model for B100 fuel (a) variation in dilution ratio from 10:1 to 1000:1 for constant temperature of 45°C (b) variation in temperature from 15°C to 45°C for a constant dilution ratio of 100:1.
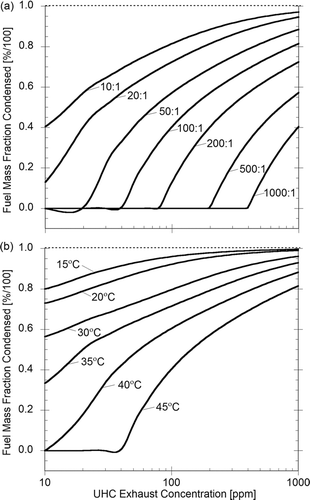
Aftertreatment systems implemented on vehicles also will reduce the amount of biodiesel condensing onto the PM in the exhaust. For example, diesel oxidation catalysts (DOC) convert a portion of the UHC in the exhaust before dilution with the atmosphere occurs. For LTC operating conditions where exhaust temperatures are relatively low, such catalysts do not convert all UHC in the exhaust (CitationNorthrop et al. 2007). With biodiesel use, unconverted methyl esters in the exhaust could still contribute significantly to the tailpipe PM emissions for these applications.
3.3. CONCLUSIONS
Experiments were conducted on a diesel engine at a conventional operating condition and early and late partially premixed low temperature combustion modes for three fuels; a U.S. specification ultra-low sulfur diesel fuel, soy-based biodiesel, and a 50% blend of the two neat fuels. UHC emissions, considered to consist mainly of unburned fuel remaining from the combustion process increased from conventional combustion to LLTC and from LLTC to ELTC as over-leaning of the fuel and air mixture in the cylinder increased. The primary conclusion from the work is that for partially premixed LTC of biodiesel, excessive gas-phase unburned methyl esters in the raw exhaust condense onto a relatively smaller quantity of soot after dilution with atmospheric air. This condensation process resulted in over an order of magnitude increase in PM emissions for B100 for the LLTC condition as compared to a petroleum diesel fuel. For the ELTC condition, PM emissions were almost 100 times higher for B100 over ULSD.
Particulate emissions for conventional combustion decreased with increasing biodiesel primarily due to the reduction in soot emissions, confirming known trends. Soot decreased for all operating conditions with increasing biodiesel content in the fuel. From speciation of extracted PM using GC-FID, the primary contributors to increased PM emissions for biodiesel LTC were unburned methyl ester species.
Condensation is the primary mechanism by which unburned biodiesel in the gas phase converts to the particle phase following dilution for LTC operation. A mathematical model based on Raoult's Law was developed to predict the amount of condensed unburned fuel on the PM given the dilution ratio, filter collection temperature and concentration of individual fuel species in the raw exhaust. High molecular weight methyl ester components contained in biodiesel have lower vapor pressure and were found to reach near 95% conversion to the particle phase with concentrations of 1000 ppm of UHC in the undiluted exhaust for a 10:1 dilution ratio and 47°C collection temperature. The condensation model accurately predicted the mass increase of PM both in trend and magnitude as the concentration of unburned biodiesel in the raw exhaust increased.
Acknowledgments
This research was primarily funded by the General Motors, University of Michigan Collaborative Research Laboratory. The authors wish to thank P. Szymkowicz and the Diesel Engine Systems Group at General Motors Global Research and Development for their support of the work. The authors also wish to acknowledge the US-EPA National Vehicle and Fuel Emissions Laboratory for allowing access to particulate filter weighing facilities. S. Batterman and S. Chernyak from the School of Public Health at the University of Michigan were instrumental in conducting OCEC analyses and concentrating extracted samples in support of the research.
REFERENCES
- Aakko , P. and Nylund , N. O. 2003 . Particle Emissions at Moderate and Cold Temperatures using Different Fuels . SAE Technical Paper Series , 2003-01-3285
- Bagley , S. T. , Gratz , L. D. , Johnson , J. H. and McDonald , J. F. 1998 . Effects of an Oxidation Catalytic Converter and a Biodiesel Fuel on the Chemical, Mutagenic, and Particle Size Characteristics of Emissions from a Diesel Engine . Environ. Sci. Technol. , 32 : 1183 – 1191 .
- Bennett , M. , Volckens , J. , Stanglmaier , R. , McNichol , A. P. , Ellenson , W. D. and Lewis , C. W. 2008 . Biodiesel Effects on Particulate Radiocarbon (14C) Emissions from a Diesel Engine . J. Aerosol Sci. , 39 : 667 – 678 .
- Birch , M. E. and Cary , R. A. 1996 . Elemental Carbon-Based Method for Monitoring Occupational Exposures to Particulate Diesel Exhaust . Aerosol Sci. and Tech. , 25 : 221 – 240 .
- Chang , D. Y. and Van Gerpen , J. H. 1998 . Determination of Particulate and Unburned Hydrocarbon Emissions from Diesel Engines Fueled with Biodiesel . SAE Technical Paper Series , 982527
- Clerc , J. C. and Johnson , J. H. 1982 . A Computer Heat Transfer and Hydrocarbon Adsorption Model for Predicting Diesel Particulate Emissions in Dilution Tunnels . SAE Technical Paper Series , 821218
- Cook , D. J. , Pitsch , H. and Nentwig , G. 2008 . Numerical Investigation of Unburnt Hydrocarbon Emissions in a Homogeneous-Charge Late Injection Diesel-Fueled Engine . SAE Technical Paper Series , 2008-01-1666
- Di , Y. , Cheung , C. S. and Huang , Z. 2009 . Comparison of the Effect of Biodiesel-Diesel and Ethanol-Diesel on the Particulate Emissions of a Direct Injection Diesel Engine . Aerosol Sci. and Tech. , 43 : 455 – 465 .
- Durán , A. , Monteagudo , J. M. , Armas , O. and Hernandez , J. J. 2006 . Scrubbing Effect on Diesel Particulate Matter from Transesterified Waste Oil Blends . Fuel , 85 : 923 – 928 .
- Figliola , R. and Beasley , D. 2000 . Theory and Design for Mechanical Measurements , (3rd ed.) , New York : John Wiley and Sons .
- Genzale , C. L. , Reitz , R. D. and Musculus , M. P. 2009 . Effects of Spray Targeting on Mixture Development and Emissions Formation in Late Injection Low-Temperature Heavy-Duty Diesel Combustion . Proc. Combustion Inst. , 32 : 2767 – 2774 .
- Gonzalez , D. M. , Piel , W. , Asmus , T. , Clark , W. , Garbak , J. Liney , E. 2001 . Oxygenates Screening for Advances Petroleum-Based Diesel Fuels: Part 2. The Effect of Oxygenate Blending Compounds on Exhaust Emissions . SAE Technical Paper Series , 2001-01-3632
- Han , M. , Assanis , D. N. and Bohac , S. V. 2009 . Sources of Hydrocarbon Emissions from Low-Temperature Premixed Compression Ignition Combustion from a Common-Rail Direct Injection Diesel Engine . Combust. Sci. Technol. , 181 : 496 – 517 .
- Heikkilä , J. , Virtanen , A. , Rönkko , T. , Keskinen , J. , Aakko-Saksa , P. and Murtonen , T. 2009 . Nanoparticle Emissions from Heavy-Duty Engine Running on Alternative Diesel Fuels . Environ. Sci. Technol. , 43 : 9501 – 9506 .
- Ickes , A. M. , Bohac , S. V. and Assanis , D. N. 2009 . Effect of Fuel Cetane Number on a Premixed Diesel Combustion Mode . Int. J. Engine Res. , 10 : 251 – 263 .
- Jacobs , T. J. and Assanis , D. N. 2007 . The Attainment of Premixed Compression Ignition Low-Temperature Combustion in a Compression Ignition Direct Injection Engine . Proc. Combust. Instit. , 31 : 2913 – 2920 .
- Jacobs , T. J. , Bohac , S. V. , Assanis , D. N. and Szymkowicz , P. G. 2005 . Lean and Rich Compression Ignition Combustion in a Light Duty Diesel Engine . SAE Technical Paper Series , 2005-01-0166
- Jacobson , M. Z. , Kittleson , D. B. and Watts , W. F. 2005 . Enhanced Coagulation Due to Evaporation and its Effect on Nanoparticle Evolution . Environ. Sci. Tech. , 39 : 9486 – 9492 .
- Lapuerta , M. , Armas , O. and Rodriguez-Fernandez , J. 2008 . Effect of Biodiesel Fuels on Diesel Engine Emissions . Prog. Energy and Combust. Sci. , 34 : 198 – 223 .
- Lapuerta , M. , Armas , O. , Ballesteros , R. and Carmona , M. 2000 . Fuel Formulation Effects on Passenger Car Diesel Engine Particulate Emissions and Composition . SAE Technical Paper Series , 2000-01-1850
- Lapuerta , M. , Hernandez , J. J. , Ballesteros , R. and Durán , A. 2003 . Composition and Size of Diesel Particulate Emissions from a Commercial European Engine Tested with Present and Future Fuels . Proc. Instn. Mech. Eng., Part D: J. Automobile Engineer. , 217 : 907 – 919 .
- McNair , H. M. and Miller , J. M. 1997 . Basic Gas Chromotography. , New York : John Wiley and Sons .
- Miyamoto , N. , Ogawa , H. , Nurun , N. M. , Obata , K. and Teruyoshi , A. 1998 . Smokeless, Low NOx, High Thermal Efficiency, and Low Noise Diesel Combustion with Oxygenated Agents as Main Fuel . SAE Technical Paper Series , 980506
- Northrop , W. F. , Jacobs , T. J. , Assanis , D. N. and Bohac , S. V. 2007 . Deactivation of a Diesel Oxidation Catalyst due to the Exhaust Species from Rich Premixed Compression Ignition in a Light-Duty Diesel Engine . Int. J. Engine Res. , 8 : 487 – 498 .
- Northrop , W. F. , Bohac , S. V. and Assanis , D. N. 2009 . Premixed Low Temperature Combustion of Biodiesel and Blends in a High Speed Compression Ignition Engine . SAE Technical Paper Series , 2009-01-0133
- Plee , S. L. and MacDonald , S. J. 1980 . Some Mechanisms Affecting the Mass of Diesel Exhaust Particulate Collected Following a Dilution Process . SAE Technical Paper Series , 800186
- Rantenen , L. , Mikkonen , S. , Nylund , L. , Kociba , P. , Lappi , M. and Nylund , N. O. 1993 . Effect of Fuel on the Regulated, Unregulated and Mutagenic Emissions of DI Diesel Engines . SAE Technical Paper Series , 932686
- Scanlon , J. T. and Willis , D. E. 1985 . The Calculation of FID Response Factors Using the Effective Carbon Number Concept . J. Chromatographic Sci. , 23 : 333 – 340 .
- Ulberth , F. , Gabernig , R. G. and Schrammel , F. 1999 . Flame Ionization Detector Response to Methyl, Ethyl, Propyl, and Butyl Esters of Fatty Acids . J. Am. Org. Chem. Society , 76 : 263 – 266 .
- Wu , F. , Wang , J. , Chen , W. and Shuai , S. 2009 . A Study on Emission Performance of a Diesel Engine Fueled with Five Typical Methyl Ester Biodiesels . Atm. Env. , 43 : 1481 – 1485 .
- Yaws , C. L. 2003 . Yaws’ Handbook of Thermodynamic and Physical Properties of Chemical Compounds , Knovel. Available online at: http://knovel.com/web/portal/browse/display?_EXT_KNOVEL_DISPLAY_bookid=667&VerticalID-0
- Yuan , W. , Hansen , A. C. and Zhang , Q. 2003 . Predicting the Physical Properties of Biodiesel for Combustion Modeling . Trans. of the ASAE , 46 : 1487 – 1493 .
- Yuan , W. , Hansen , A. C. and Zhang , Q. 2005 . Vapor Pressure and Normal Boiling Point Predictions for Pure Methyl Esters and Biodiesel Fuels . Fuel , 84 : 943 – 950 .
- Zheng , M. , Mulenga , M. C. , Reader , G. T. , Wang , M. , Ting , D. S.-K. and Tjong , J. 2008 . Biodiesel Engine Performance and Emissions in Low Temperature Combustion . Fuel , 87 : 714 – 722 .