Abstract
Drug loading and processing conditions in a dry powder inhaler system are critical for performance of the system since it is these forces that must be overcome to properly redisperse respirable particles. In this work, we have investigated the effects of different loading forces for adhering micronized drug onto surfaces on the drug adhesion, detachment, and aerosol performance under controlled conditions. Drug loading onto a standardized surface was performed under three different loading conditions using turbula-mixer to actively induce powder–surface interactions. It was seen the drug loading increased with increased processing time. The presence of external press-on force also increased drug loading. Drug detachment studies performed using centrifugation method indicated that adhesion forces were the lowest at lower mixing time and increased with increasing press-on forces. Drug aerosolization performance from the surface was assessed using a prototype DPI and confirmed that external forces during drug loading and coating processing played an important role in drug dispersion. It was seen that the respirability of drug particles correlated with mixing times as well as the press-on forces.
Introduction
The aerosol dispersion performance of a dry powder inhaler (DPI) depends mainly on the inhaler device, the inhalation scheme and the formulation (Hickey et al. Citation1994; Telko and Hickey Citation2005; Smith and Parry-Billings Citation2003). In general, DPI formulations are interactive mixtures consisting of an active ingredient (drug) blended with larger carrier particles, such as α-lactose monohydrate (Bell et al. Citation1971). The carrier particles in the DPI formulations aid flow, fluidization, and enhance dispersion properties of the formulation (Smyth and Hickey Citation2005; Hickey et al. Citation2007).
The performance of these interactive mixtures is a function of the relative magnitudes of cohesive (drug–drug) and adhesive (drug–excipient) interparticulate forces (Hickey et al. Citation1994; Begat et al. Citation2004a, Citation2004b; Lohrmann et al. Citation2007; Louey et al. Citation2003; Dickhoff 2006; Jones et al. Citation2008). For example, strong adhesive forces may result in rapid and uniform mixing but may also prevent the release of the respirable drug particles from the carrier during inhalation (Hickey et al. Citation1994) Conversely, strong cohesive forces may lead to particle segregation and heterogeneity during mixing but may also facilitate carrier–drug deaggregation during inhalation (Saleem et al. Citation2008). By modifying this adhesive–cohesive force balance, we may enhance the efficiency of dispersion (Begat et al. Citation2004a, b).
The processing of these interactive mixtures plays a crucial role in DPI formulations. The most commonly used pharmaceutical process used in preparing these interactive DPI formulations is blending and mixing. The commonly used blending equipments for dry powder inhaler formulations are two stage V-shaped blender and Turbulamixer (Saleem et al. Citation2008). The blending process is highly critical and should be subject to substantial optimization work during development (Saleem et al. Citation2008). When mixing powders with different properties, particle sizes, and ratios, as is the case with DPI formulations, inadequate mixing can cause poor dose uniformity. In many cases, inadequate mixing cannot be overcome simply by increasing the mixing time (Dickhoff 2006). Mixer selection, rotation speed, capacity, and fill level are all subject to optimization, as they can all affect the blend homogeneity (Telko and Hickey Citation2005). What has been studied less is how blending conditions may affect the inter-particulate forces, which are a primary determinant of the fine-particle fraction (Lida et al. Citation2000). Different powders may have different mixing requirements, depending on the forces present between the various particles (Krycer and Hersey Citation1980; Staniforth et al. Citation1985). With cohesive materials, ordered mixing may be as important as randomization for producing pharmaceutical mixtures (Hersey Citation1975). For low concentration (drug-carrier ratio) blends, geometric dilutions are necessary pre-blending steps (Young et al. Citation2005). The flow properties of the components of the powder blend will play an important role in the efficiency of blending and, ultimately, in aerosol dispersion (Crowder and Hickey Citation2006; Shur et al. 2008).
When an aeroelastic object is placed in the path of an airflow, an aerodynamic phenomenon known as flutter may occur (Gallegos 2008). This type of fluid structure interaction is very common in nature and has been extensively investigated in the field of aeronautics; but thus far, flutter has been mainly examined as a detrimental process. We have developed a bench prototype, wherein this aeroelastic vibrational energy is used to disperse and deaggregate powders into an aerosol cloud suitable for inhalation therapy (Smyth and Truman 2008). In previous studies, we employed the technique of laser vibrometry to accurately and rapidly quantify vibrations in structures and a strong relationship between dimensionless frequency and dimensionless tension was obtained (Smyth et al. Citation2008). The study indicated that flutter frequency is independent of air speed at expected flow rates, and we can “tune” flutter using film properties such as film tension, mass, and length. It was also seen that the acceleration that was produced in the films in these non-optimized flow regimes was very high—in some cases exceeding 120 g's. These findings suggest we can tune our dispersion platform to match patient, drug, and device factors, and that this mechanism may be highly efficient for powder deaggregation (Donovan et al. Citation2009).
In this study, we investigate the effect of mixing and drug loading conditions on the drug adhesion and dispersion. We also investigated the effect of external press-on forces on drug adhesion. The effect of these formulation parameters on drug aerosol dispersion was also studied using prototype DPI. Because this device employs a film as the “carrier” surface, we could investigate the drug coating dynamics without confounding parameters inherent in lactose particulate systems such as surface morphology, rugosity, and three-dimensional surfaces of the particles.
Materials and Methods
Materials
The model drug employed in these studies was micronized budesonide (Spectrum Chemicals, Gardena, CA) and was used as received. Ethanol (100% v/v) was purchased from Sigma (St. Louis, MO). The standardized surface film used in this study is a polyolefin MPF film of 0.085 mm thickness. It was obtained from Dow Chemicals. The beads used in the study are made of plastic (0.13 g.cm–3) and stainless steel (1.1 g.cm–3) with an average diameter of 3.5 cm and was purchased from Precision max.
Bench Prototype
represents the prototype DPI. It consists of a nozzle located at the rear of the device, in line with the mouthpiece outlet. There are two film holders, which can be moved along the axis of the film. The standardized film surface is cut into a rectangular film with dimensions of 8 cm × 1 cm. Once the standardized film is loaded with drug, the film is then attached between the film holders at a tension of 2N and loaded in to the prototype DPI as depicted in . The resistance of the device was determined as described elsewhere (Srichana et al. 1998). Typically, a digital manometer recorded the pressure drop across the prototype DPI device for various flow rates. The mean pressure drop was plotted against the corresponding flow rate and the slope of the fitted line is reported as the device resistance. The resistance of the prototype was 0.07 (cm of H2O)0.5·lit–1·min for this particular configuration.
Physicochemical Characterization
Drug
The Model drug used in this study was micronized budesonide. The size and shape of the drug was determined using Scanning Electron Microscopy (SEM). Micronized budesonide samples were mounted on aluminum stubs with double-sided conducting carbon tapes and coated for 8 min with a 50/50 mixture of Au/Pd to minimize surface charging. The coating thickness was 15–17 nm. The samples were scanned at an accelerating voltage of 20 KV. The SEM pictures were then digitally processed using ImageJ software to get the average diameter. The SEM digital image consisting of 20–30 particles was first converted into a binary image at a predetermined threshold. The number of particles and the average particle size of the counted particles were obtained by the ‘analyze particles’ mode of the software.
The drug removed and retained on the film surface of substrate was analyzed spectrophotometrically. Budesonide was analyzed in ethanol at 244 nm using UV-Vis spectrophotometer (Infinite M 200 fluorophotometer, TECAN, USA). A calibration curve (r2= 0.998) was extrapolated to determine the concentration of budesonide.
Film
The surface of the film was analyzed using Atomic Force microscopy. Atomic Force Microscopy was performed on a Veeco MultiMode 8 equipped with a NanoScope IIId (Veeco Instruments, Santa Barbara, CA) mounted on an air vibration isolation table. RTESP silicon cantilevers (Veeco Instruments) with a nominal spring constant of 40 N/m were used in tapping mode. Instrument parameters were optimized for surface topography measurements. Surface roughness, Rq (RMS), was calculated (Equation (Equation1)) with the built-in roughness function in the software (version 5.31R1) after a first-order flattening of the image, with maximum dimensions of 17 μm × 17 μm.
Drug Loading Studies. Model drug was loaded onto a standardized surface as described below. The standardized surface film was fixed onto an insert that could be placed in a mixing chamber. The surface area of the standardized surface was 1 cm2. The assembly was then fixed on top of an open-top cylindrical mixing chamber as shown in . The mixing chamber contained micronized drug with four beads (plastic or stainless steel) or no beads at all. Beads were used to reduce drug aggregation and to induce different mixing forces. The assembly was then loaded in a turbulamixer (Type 2C, Glenmills Inc., NJ, USA) and mixed for different times at constant mixing speed of 40 rpm. A similar setup was used to load micronized drug onto a film for the prototype DPI. Instead of the standardized film surface attached to the film holder, an 8 cm × 1 cm polyolefin film attached to the film holder was used. This assembly was then loaded in a turbulamixer and mixed for different times and for different press-on forces (using beads) at a mixing speed of 40 rpm.
The press-on forces were estimated using the following assumptions: ignoring the frictional forces, falling motion of the beads and accounting for only the rolling motion of the beads as well as the total weight of the powder. It should be noted, that the objective of estimating these forces was to allow meaningful comparisons between the loading conditions as the assumptions for each loading condition would remain the same.
Centrifugal Detachment Studies
Drug detachment from the surfaces of the standardized material was performed using centrifugal methods (Podczeck et al. Citation1996; Podczeck 1998a. The drug loaded standardized film surface was then loaded onto a centrifuge tube and placed into an ultracentrifuge rotor (Hitachi Ltd, Tokyo, Japan). The drug-loaded film has its axis perpendicular to the rotational axis of the centrifuge. The standardized film surface was then subjected to a centrifugal force of 715·g (4000 rpm). The use of a centrifuge force to measure drug adhesion is based on the principle that the resulting force from the angular speed of rotation of a particle with a defined mass in a known distance to the rotation center will exceed the adhesion force between a population of particles and the surface above a critical centrifugal speed. The adhesion force is equal in magnitude, but with opposing signal to the applied centrifugal force at detachment (Krupp Citation1937; Podczeck 1998a; Podczeck et al. Citation1996).
Aerosol Dispersion Studies
Dispersion performance was assessed using a prototype DPI (), in which the drug-loaded surfaces were exposed to standardized flow conditions (60 lpm (8.85 m.s–2) for 4 s) and quantified using the twin stage liquid impinger (TSI). The TSI consists of two stages. Stage 1 contains 7 ml of ethanol while stage 2 contains 30 ml of ethanol. This produced an aerodynamic cut-off mass median aerodynamic diameter (MMAD) of 6.4 μm between the two stages at 60 lpm. The fine particle fraction (FPF) is the fraction of the emitted dose possessing aerodynamic diameter less than 6.4 microns. Therefore, the particle fraction collected in stage 2 of the TSI is tabulated as FPF.
Results and Discussion
Physicochemical Characterization
1. | Drug: A SEM image of the drug shown in suggests that particles of micronized budesonide are irregular plates with low aspect ratios. Image J analysis of the SEM images determined the size of the budesonide particles was 3.8 ± 0.7 μm. | ||||
2. | Film: represents the topography of the film using AFM. Optically visible striations were present in the film, and most likely due to the stretching of the film during manufacturing. The surface roughness on both sides of the film varied between 85 ± 20 nm, indicating a smooth surface relative to the size of the drug particle size scale. Hence we expect that these surface irregularities of the film are not sufficient enough to shield the drug particles from experiencing the press-on forces during mixing as it was seen with coarse lactose particles (Smyth and Hickey Citation2005; Lida et al. Citation2000a; Podczeck 1998b; Zeng et al. 2000; Dickhoff et al. 2005). |
Drug Loading
1. | Effect of time: The drug coating was analyzed for three different milling times: 1, 10, and 30 min. As seen in Table 3, the drug loading increases with time irrespective of the presence of beads. In the absence of beads, drug loading increased from 108.89 ± 19.13 μg·cm–2 at 1 min to 320.98 ± 25.85 μg·cm–2 at 30 min. A similar trend could be seen in the presence of beads. In case of plastic beads, drug loading increased nearly 10 fold as the loading time increased from 1 to 30 min. In the case of high-density stainless steel beads, an additional 3-fold increase in drug loading is achieved in the same time frame. The observation that drug coating on the surface of the films increases with time for all three loading conditions is important. The surface morphology has been shown to have initial roughness on the order of 100 nm, which may play a role in particle coating (Begat et al. Citation2004b). However, the surface area of the films being coated is quite small with respect to the surface area of the drug particles. A monolayer of particles on the square centimeter of film has been theoretically estimated to be equivalent to 98.94 mcg of drug. Therefore, the interpretation that surface morphology may have significant effects of drug loading over time may be less important. As we see from , after 1 min all loading conditions have exceeded this theoretical monolayer threshold. The authors believe that increase in drug loading with time is related to formation of drug–drug aggregates on the film surface as more particles are adhered over time. Studies in lactose have previously shown drug adhesion is a dynamic phenomenon (Saleem et al. Citation2008). Drug adhesion can increase with increase mixing times and also drug can be redistributed following initial adhesion. In particular, this was also observed in our studies with the steel bead condition where drug loading tended to decrease between 10 and 30 min, even though they are statistically insignificant (p < 0.1). Previous studies on mixing budesonide on to lactose carrier particles have shown that as mixing time increases, there is a tendency for drug aggregation on the lactose surface (Dickhoff et al. Citation2003, Citation2005). The drug loading also reaches saturation as the time increases. This is due to the fact that film surface is saturated with a monolayer and further mixing induces a formation of drug aggregates on the film surface. Similar effects were observed in previous studies with lactose carrier particle that during mixing, drug particles subjected to relatively low adhesive forces may be detached from the carrier particles again and be relocated into areas with higher bonding capacity (de Boer et al. Citation2005). As a result the number of particles attached to active sites increases with increasing mixing time (de Boer et al. Citation2005). Moreover the mixing action of the turbulamixer may induce some electrostatic charges on to the drug particles via triboelectrification, which may enhance adhesion between drug–drug and drug–standardized surface, and this would increase with time (Saleem et al. Citation2008). TABLE 1 Effect of time on drug loading | ||||
2. | Effect of Mixing Forces: shows the effect of mixing forces on the drug loading. Beads are used to reduce drug aggregation on the film surface as well as to impart additional press-on forces. In the absence of beads, i.e. at an press-on force of 2 × 10–4N, the drug loading is lower with a maximum loading at 30 min of 320.98 ± 25.84 μg·cm–2. Drug loading reaches saturation at around 30 min. However, in the presence of low-density plastic beads, at a press-on force of 22.5 × 10–4N, the drug loading increased to 1042.76 ± 77.98 μg·cm–2 at 30 min. In the presence of beads, drug adhesion is increased due to additional press-on forces resulting in higher drug loading. FIG. 5 Effect of beads on the drug loading onto polyolefin film at various times. The times investigated are 1, 10, and 30 min. (Figure provided in color online.) ![]() Using high-density stainless steel beads imparting an external press-on force of 35.5 × 10–4N, we can achieve high drug loadings at lower mixing times. The drug loading is further enhanced to 1462.28 ± 235.3 mcg·cm–2. This is due to the capability of high-density stainless steel beads to impart higher press-on adhesion energy. However, the maximum drug loading occurs at 10 min (1700.1 ± 138.66 mcg·cm–2), after which it reaches a plateau. With the entire standardized surface covered with a monolayer of drug particle, the continued mixing with high-density stainless steel beads may result in multi layers of drug pressed on to the existing monolayer. With increased residence time of mixing, the continued collisions of stainless steel beads on this multilayer may also result in removing some of the drug particles. Thus the drug loading is both a function of drug loading time as well as external press-on forces (beads). |
Drug Adhesion
Drug adhesion studies were performed using the centrifugation method. The rotational centrifugal force used in this study is 715·g (4000 rpm). shows the percentage of budesonide remaining on the film surface as a function of the drug loading time. It is clear that as the loading time increases, so does the % budesonide remaining on the film. This is a clear indication of increasing press-on forces with drug loading time. It is also seen that % budesonide remaining on the film is higher when steel beads are used compared to plastic beads and no beads. This is because the heavier stainless steel beads impart higher press-on forces as compared to plastic beads. From our previous experience, it was clear that as increasing mixing energy increases external press-on forces and drug adhesion to the film surface is enhanced (Selvam and Smyth 2010). It was also shown that by increasing mixing time or energy, budesonide particles bound to the cavities on carrier surface are redistributed over the carrier surface where they come in reach of press-on forces (Dickhoff 2006).
Drug Dispersion
The TSI was used to determine the aerosol characteristics of the prototype DPI. shows the aerosol characteristics of the drug dispersion as a function of mixing times as the drug is loaded onto the standardized surface using plastic beads. From , it is clear that as drug loading time increases, higher percentage of drug is retained in the film. This is due to increased drug adhesion (higher press-on forces). The FPF is a maximum at 1 min at 70.07 ± 4.71%. Comparing this to the commercial budesonide turbuhaler DPI, the FPF is reported to be 44% (Ball et al. 2002; Bisgaard et al. Citation1998; Thorsson et al. Citation1994; Borgstrom et al. Citation1994). While FPF is almost similar at 10 and 30 min drug loading, at 55.91 ± 6.81% and 55.97 ± 5.97%, respectively. However the fine particle dose (FPD) increases with time. This is due to the higher drug loading onto the film as mixing time increases. Similar studies are performed with high-density stainless steel beads as well as a scenario wherein no beads were used.
FIG. 7 Effect of mixing times on aerosol performance using TSI. Drug loading was performed using plastic beads. (Figure provided in color online.)
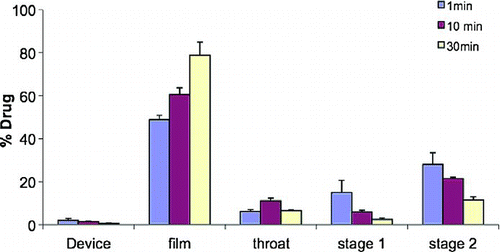
shows the aerosol characteristics determined using TSI in the presence and absence of beads at a drug loading time of 10 and 30 min. The film retention is the lowest when plastic beads are used. The film retention and RF decreases as the time of mixing increases. In the absence of beads, lower RF and higher film retention was observed. This is due to the formation of multilayer aggregates of highly cohesive budesonide particles and the presence of beads help to decrease the cohesive nature of budesonide particles and deaggregate the particles over time. When high density stainless steel beads are used for drug loading, RF of the resulting drug dispersions is lower (<10%) compared to the low density plastic beads. This correlates to the higher press-on adhesion energy imparted by the stainless steel beads compared to the plastic beads. In traditional DPI formulations, the intensity and the time of mixing drug and a carrier particle will also affect drug dispersion during inhalation. It was shown that at lower mixing intensity of 20 rpm (Turbulamixer) resulted in a higher budesonide deposition in the later stages of a multi stage liquid impinger as compared to a higher mixing intensity of 90 rpm (Dickhoff et al. Citation2003). Similar to the plastic beads, as the drug loading time increases, the FPF decreases to as low as 12% for steel beads. In the case when no beads are used the FPF obtained is 46–49%. There is no significant change (p < 0.05) in FPF with drug loading time. Thus it could be seen that the forces of drug loading on to the film surface affect the aerosol characteristics of the drug dispersions. These forces are responsible for drug aggregation on the lactose carrier surface (Kulvanich and Stewart Citation1987; de Boer et al. Citation2004a). This aggregation increases the magnitude of the removal forces acting on such particles during inhalation (Louey and Stewart Citation2002) and the degree of aggregation might affect the FPF (de Boer et al. Citation2004a, Citation2004b; Podczeck 1998b). If drug aggregation is strong enough to withstand the forces that need to break them up, the fraction detached may be high, but FPF will be unsatisfactory (Podczeck 1998b). Such aggregations would be broken up by the frictional and inertial press-on forces during mixing, provided sufficient mixing time (de Boer et al. Citation2004a). The type of mixer used, the batch size and the mixing time determine the magnitude of these forces.
TABLE 2 Aerosol characteristics of the dispersion as a function of beads and drug loading time
Conclusion
In this study, we have found that conditions of drug loading have profound impact on the drug adhesion forces and the resultant drug dispersion using a prototype DPI. As the drug loading time increases, so does the drug adhesion and hence the amount of drug loaded on to the standardized film surface. With the help of additional press-on forces, in our case beads, we can further increase the amount of drug loaded onto the standardized surface. Drug adhesion increased further with the employment of beads as determined by centrifugation methods. The aerosol dispersion studies using our prototype DPI indicated that as the drug loading time increases and increasing external press-on forces, the drug retained on the standardized surface increases resulting in decreasing FPF.
REFERENCES
- Ball , D. J. , Hirst , P. H. , Newman , S. P. , Sonet , B. , Streel , B. and Vanderbist , F. 2002 . Deposition and Pharmacokinetics of Budesonide from the Miat Monodose Inhaler, a Simple Dry Powder Device . Int. J. Pharm. , 245 : 123 – 132 .
- Begat , P. , Morton , D. A. V. , Staniforth , J. N. and Price , R. 2004a . The Cohesive–Adhesive Balances in Dry Powder Inhaler Formulations II: Influence on Fine Particle Delivery Characteristics . Pharm. Res. , 21 : 1826 – 1833 .
- Begat , P. , Morton , D. A. V. , Staniforth , J. N. and Price , R. 2004b . The Cohesive–Adhesive Balances in Dry Powder Inhaler Formulations I: Direct Quantification by Atomic Force Microscopy . Pharm. Res. , 21 : 1591 – 1597 .
- Bell , J. H. , Hartley , P. S. and Cox , J. S. G. 1971 . Dry Powder Aerosols I: a New Powder Inhalation Device . J. Pharm. Sci. , 60 : 1559 – 1564 .
- Bisgaard , H. , Klug , B. , Sumby , B. S. and Burnell , P. K. 1998 . Fine Particle Mass from the Diskus Inhaler and Turbuhaler Inhaler in Children with Asthma . Eur. Respir. J. Eur. Soc. Clin. Respir. Physiology. , 11 : 1111 – 1115 .
- de Boer , A. H. , Dickhoff , B. H. J. , Hagedoorn , P. , Gjaltema , D. , Goede , J. , Lambregts , D. and Frijlink , H. W. 2005 . a Critical Evaluation of the Relevant Parameters for Drug Redispersion from Adhesive Mixtures During Inhalation . Int. J. Pharm. , 294 : 173 – 184 .
- de Boer , A. H. , Hagedoorn , P. , Gjaltema , D. , Lambregts , D. , Irngartinger , M. and Frijlink , H. W. 2004a . The Mode of Drug Particle Detachment from Carrier Crystals in an Air Classifier–Based Inhaler . Pharm. Res. , 21 : 2167 – 2174 .
- de Boer , A. H. , Hagedoorn , P. , Gjaltema , D. , Lambregts , D. , Irngartinger , M. and Frijlink , H. W. 2004b . The Rate of Drug Particle Detachment from Carrier Crystals in an Air Classifier–Based Inhaler . Pharm. Res. , 21 : 2158 – 2166 .
- Borgstrom , L. , Bondesson , E. , Moren , F. , Trofast , E. and Newman , S. P. 1994 . Lung Deposition of Budesonide Inhaled Via Turbuhaler: A Comparison with Terbutaline Sulphate in Normal Subjects . Eur. Respir. J. , 7 : 69 – 73 .
- Crowder , T. and Hickey , A. J. 2006 . Powder Specific Active Dispersion for Generation of Pharmaceutical Aerosols . Int. J. Pharm. , 327 : 65 – 72 .
- Dickhoff , B. H. J. 2006 . Adhesive Mixtures for Powder Inhalation: The Effect of Carrier (Surface and Bulk) Properties, Carrier Payload and Mixing Conditions on the Performance of Adhesive Mixtures for Inhalation. , Netherlands : University of Groningen .
- Dickhoff , B. H. J. , de Boer , A. H. , Lambregts , D. and Frijlink , H. W. 2005 . The Interaction Between Carrier Rugosity and Carrier Payload, and Its Effect on Drug Particle Redispersion from Adhesive Mixtures During Inhalation . Eur. J. Pharm. Biopharm. , 59 : 197 – 205 .
- Dickhoff , B. H. J. , de Boer , A. H. , Lambregts , D. and Frijlink , H. W. 2003 . The Effect of Carrier Surface and Bulk Properties on Drug Particle Detachment from Crystalline Lactose Carrier Particles During Inhalation, as Function of Carrier Payload and Mixing Time . Eur. J. Pharm. Biopharm. , 56 : 291 – 302 .
- Donovan , M. , Selvam , P. , Singh , S. , McNair , D. , Truman , R. C. and Smyth , H. D. C. 2009 . Tunable Dry Powder Inhalers: Future or Folly? . Res. Drug. Del. , 1 : 189 – 200 .
- Gallegos , M. 2008 . Flow Induced Flutter of Thin Elastic Films. M.S. , Albuquerque, NM : University of New Mexico .
- Hersey , J. A. 1975 . Powder Mixing: Theory and Practice in Pharmacy . Powder Tech. , 15 : 149 – 153 .
- Hickey , A. J. , Concession , N. M. , Van Oort , M. and Platz , M. 1994 . Factors Influencing the Dispersion of Dry Powders as Aerosols . Pharm. Tech. , 8 : 58 – 82 .
- Hickey , A. J. , Monsour , H. M. , Telko , M. J. , Zeng , X. , Smyth , H. D. C. , Mulder , T. , Mclean , R. , Langridge , J. and Papadopoulos , D. 2007 . Physical Characterization of Component Particles Included in Dry Powder Inhalers. II. Dynamic Characteristics . J. Pharm. Sci , 96 : 1302 – 1319 .
- Jones , M. D. , Harris , H. , Hooton , J. C. , Shur , J. , King , G. S. , Mathoulin , C. A. and Nichol , K. 2008 . An Investigation into the Relationship between Carrier-Based Dry Powder Inhalation Performance and Formulation Cohesive-Adhesive Force Balances . Eur. J. Pharm. Biopharm. , 69 : 496 – 507 .
- Krupp , H. 1937 . Particle Adhesion, Theory and Experiment . Adv. Colloids. Interface Sci. , 1 : 11 – 259 .
- Krycer , I. and Hersey , J. A. 1980 . Fine Powder Mixing in a Vibratory Ball Mill . Int. J. Pharm. , 6 : 119 – 129 .
- Kulvanich , P. and Stewart , P. J. 1987 . The Effect of Particle Size and Concentration on the Adhesive Characteristics of a Model Drug-Carrier Interactive System . J. Pharm. Pharmacol. , 39 : 673 – 678 .
- Lida , K. , Leuenberger , H. , Fueg , L. M. , Muller-Walz , R. , Okamoto , H. and Danjo , K. 2000 . Effect of Mixing of Fine Carrier Particles on Dry Powder Inhalation Property of Salbutamol Sulfate (SS) . J. Pharm. Soc. Japan , 120 : 113 – 119 .
- Lohrmann , M. , Kappl , M. , Butt , H.-J. , Urbanetz , N. A. and Lippold , B. C. 2007 . Adhesion Forces in Interactive Mixtures for Dry Powder Inhalers—Evaluation of a New Measuring Method . Eur. J. Pharm. Biopharm. , 67 : 579 – 586 .
- Louey , M. D. , Razia , S. and Stewart , P. J. 2003 . Influence of Physico-Chemical Carrier Properties on the In Vitro Aerosol Deposition from Interactive Mixtures . Int. J. Pharm. , 252 : 87 – 98 .
- Louey , M. D. and Stewart , P. J. 2002 . Particle Interactions Involved in Aerosol Dispersion of Ternary Interactive Mixtures . Pharm. Res. , 19 : 1524 – 1531 .
- Podczeck , F. 1998a . Evaluation of the Adhesion Properties of Salbutamol Sulphate to Inhaler Materials . Pharm. Res. , 15 ( 5 ) : 806 – 808 .
- Podczeck , F. 1998b . The relationship Between Physical Properties of Lactose Monohydrate and The Aerodynamic Behaviour of Adhered Drug Particles . Int. J. Pharm. , 160 ( 1 ) : 119 – 130 .
- Podczeck , F. , Newton , J. M. and James , M. B. 1996 . The Adhesion Force of Micronized Salmeterol Xinafoate Particles To Pharmaceutically Relevant Surface Materials . J. Phys. D:Appl. Phys. , 29 : 1878 – 1884 .
- Saleem , I. , Smyth , H. D. C. and Telko , M. 2008 . Prediction of Dry Powder Inhaler Formulation Performance from Surface Energetics and Blending Dynamics . Drug Dev. Ind. Pharm. , 34 : 1002
- Selvam , P. and Smyth , H. D. C. 2010 . Effect of Press-on Forces on Drug Adhesion in Dry Powder Inhaler Formulations . J. Adhesion Sci. Tech. , submitted.
- Shur , J. , Harris , H. , Jones , M. , Kaerger , J. and Price , R. 2008 . The Role of Fines in the Modification of the Fluidization and Dispersion Mechanism within Dry Powder Inhaler Formulations . Pharm. Res. , 25 : 1631 – 1640 .
- Smith , I. J. and Parry-Billings , M. 2003 . The Inhalers of the Future? A Review of Dry Powder Devices on the Market Today . Pulmonary Pharmacol. Ther. , 16 : 79 – 95 .
- Smyth , H. D. C. , Gallegos , M. and Truman , R. C. 2008 . A Novel Mechanism of Dry Powder Inhaler Dispersion: Optimizing Flow Induced Flutter . Res. Drug Delivery , 3 : 877 – 880 .
- Smyth , H. D. C. and Truman , R. C. 2008 . Dry Powder Inhaler with Aeroelastic Dispersion Mechanism Albuquerque, NM US Patent App. 12/246, 116
- Smyth , H. D. C. and Hickey , A. J. 2005 . Carriers in Drug Powder Delivery: Implications for Inhalation System Design . Am. J. Drug Delivery , 3 : 117 – 132 .
- Srichana , T. , Martin , G. P. and Marriott , C. 1998 . Dry Powder Inhalers: The Influence of Device Resistance and Powder Formulation on Drug and Lactose Deposition In Vitro . Eur. J. Pharm. Sci. , 7 : 73 – 80 .
- Staniforth , J. N. , Rees , J. E. , Lai , F. K. and Hersey , J. A. 1985 . Interparticle Forces in Binary and Ternary Ordered Powder Mixes . J. Pharm. Pharmacol. , 34 : 141 – 145 .
- Telko , M. J and Hickey , A. J. 2005 . Dry Powder Inhaler Formulation . Respir. Care , 50 : 1209 – 1227 .
- Thorsson , L. , Edsbacker , S. and Conradson , T.-B. 1994 . Lung Deposition of Budesonide from Turbuhaler is Twice that from a Pressurized Metered-Dose Inhaler P-MDI . Eur. Respir. J. , 7 : 1839 – 1844 .
- Young , P. M. , Edge , S. , Traini , D. , Jones , M. D. , Price , R. , El-Sabawi , D. , Urry , C. and Smith , C. 2005 . The Influence of Dose on the Performance of Dry Powder Inhalation Systems . Int. J. Pharm. , 296 : 26 – 33 .
- Zeng , X. M. , Martin , G. P. , Marriott , C. and Pritchard , J. 2000a . The Influence of Carrier Morphology on Drug Delivery by Dry Powder Inhalers . Int. J. Pharm. , 200 ( 1 ) : 93 – 106 .
- Zeng , X. M. , Pandhal , K. H. and Martin , G. P. 2000b . The Influence of Lactose Carrier on the Content Homogeneity and Dispersibility of Beclomethasone Dipropionate from Dry Powder Aerosols . Int. J. Pharm. , 197 ( 1 ) : 41 – 52 .