Abstract
Size distributions of twelve polycyclic aromatic hydrocarbons (PAHs), elemental carbon (EC), organic carbon (OC) and major inorganic ions were measured at two receptor locations in the Pearl River Delta (PRD) Region in southern China during 2006–2008. The size distributions of three- and four-ring PAHs were characterized with three modes, a condensation mode with a mass median aerodynamic diameter (MMAD) in the range of 0.2–0.3 μm, a droplet mode (MMAD: .7–1.0 μm), and a coarse mode (MMAD: 3–5 μm). The less volatile five- to six-ring PAHs were mainly distributed in the condensation mode and droplet mode, with little presence in the coarse mode. The droplet mode was the most prominent mode for all PAHs at both sites, accounting for more than 70% of the total particle-phase five- and six-ring PAHs and more than 60% of the total particle-phase three- and four-ring PAHs. The dominant droplet mode could be attributed to a result of in-cloud processing of vehicular soot particles and biomass burning particles during the transport of source aerosols to the receptor sites. Presence of sulfate aqueous coating on the droplet-mode particles was hypothesized to make PAHs inaccessible to volatilization from particle phase and to oxidation by gaseous oxidants. Further implications of a dominant droplet-mode PAHs were discussed.
1. Introduction
Polycyclic aromatic hydrocarbons (PAHs) are known toxic pollutants to organisms in every compartment of the environment. Their presence in the environment is chiefly derived from incomplete combustion of fossil fuels and biomass. Atmospheric transport and deposition bring this class of persistent organic compounds to other compartments of the environment (i.e., soil, water, and sediment) and ecosystems far from their source areas. Size distribution data of PAHs are important for studying their fate in the environment and health effects. Both the nature of emission sources (e.g., type of fuel, burning conditions) and atmospheric processes after emissions affect abundance and size distribution characteristics of PAHs in the atmosphere.
A number of studies have reported PAH size distribution measurements in rural or suburban atmospheres around the world in recent years (Allen et al. 1996; Bi et al. Citation2005; Offenberg and Baker Citation1999; Kaupp and Mclachlan 2000; Eiguren-Fernandez et al. 2003; Miguel et al. Citation2004; Yang et al. 2006; Wu et al. 2006; Gnauk et al. 2008). In six of these studies, the less volatile PAHs (i.e., five- to seven-ring PAHs) were reported to be unimodally distributed and the modal peak position appeared in the fine size range; in three studies, the less volatile PAHs were also reported to exist in coarse mode in addition to the fine mode. All studies report that the more volatile PAHs (i.e., two- to four-ring PAHs) had both fine mode and coarse mode. The study by Offenberg and Baker (Citation1999) is an exception. They did not find volatile PAHs in coarse-mode aerosols collected at an over-water sampling station in Michigan Lake.
In this work, we focus on size distribution characteristics of PAHs at two locations downwind of urban areas in the Pearl River Delta Region (PRD) in South China. The objective is to characterize size distributions of PAHs at receptor sites and to discuss implications of the observation of a dominant droplet mode on the fate of PAHs.
2. Experimental section
2.1. Sample Collection
Size-segregated aerosol sampling was carried out at two rural/suburban sites in the PRD. The two locations on the map are shown in . Backgarden (BG, 23°29′14″N, 113°02′18″E) is located 48 km to the north of Guangzhou (GZ). Sampling at BG was conducted in July, a time when southeast monsoon placed BG in the downwind of Guangzhou urban area. The second site is on the campus of Hong Kong University of Science & Technology (HKUST, 23°19′12″N, 114°16′12″E) on the southeast coast of Hong Kong. The university is located in a relatively less populated area and the nearest commercial area is more than 5 km away. In winter, northwest monsoon affects the region and places HKUST site in the downwind of the PRD region. Typical trajectories of air masses during HKUST winter sampling days and BG summer sampling days are shown in .
FIG. 1 (a) Location maps of the two receptor sampling sites of urban areas in the Pearl River Delta region (1. Backgarden: summer downwind site. 2. HKUST: Winter downwind site). (b) 48 h back trajectories of air masses for all winter sampling days at HKUST (right) and all summer sampling days at BG (left). Back trajectories were calculated using NOAA's the hybrid single-particle Lagrangian integrated trajectories (HYSPLIT) model HYSPLIT (http://ready.arl.noaa.gov/HYSPLIT_traj.php). (Figure provided in color online.)
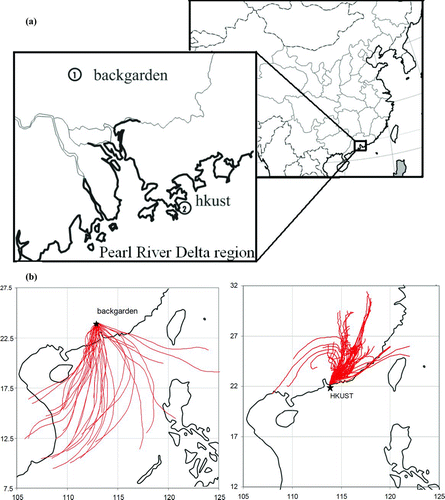
Important aerosol sources in the PRD region include vehicle exhaust, biomass burning, industrial plumes, residential cooking, and secondary formation (Cao et al. Citation2004; Yuan et al. 2006; Andreae et al. Citation2008; Duan et al. Citation2007; Hagler et al. 2006). There are no local industries or intensive vehicular traffic at the two sampling sites. The two sites are well situated to measure the outflows of the PRD urban areas in summer and winter, respectively.
Aerosols were collected using a 10-stage MOUDI (Micro Orifice Uniform Deposit Impactor, MSP Corp, Shoreview, MN) operating at a flow rate of 30 L min–1 for durations ranging from one to three days. The cutoff sizes of MOUDI are 0.056, 0.1, 0.18, 0.32, 0.56, 1.0, 1.8, 3.2, 5.6, and 10 μm. The collection substrate was prebaked quartz fiber filter. At the HKUST site, ten sets of samples were collected in January and February 2008. At the BG site, seven sets were collected in July 2006. There was no rain during sample collection. Field blank samples were collected at the beginning of each campaign.
We have previously evaluated the positive sampling artifacts of semivolatile species on quartz filter impactor substrates placed in a MOUDI sampler (the last filter stage excluded) and found little organic carbon due to adsorption (Huang et al. 2006a). Unlike aerosol collection using filtration, in an impactor sampler, organic vapors have little chance to contact the substrate filters. Negative sampling artifact is possible through vaporization due to the pressure drop in the impactor sampler, however, both theoretical and field investigations indicate that evaporative loss in MOUDI is moderate. Zhang and McMurry (1991) made a theoretical evaluation and concluded that for impactors with a moderate pressure drop, like the MOUDI, the volatilization losses for the adsorbed species (e.g., PAHs) are moderate (∼20% or less). Field evaluation of evaporative loss of ammonium nitrate, a semivolatile inorganic compound, found that MOUDI had a collection efficiency of 71 ± 23% for nitrate in comparison with a sampler that is free of both positive and negative sampling artifacts (Chang et al. Citation2001).
Particle bounce was expected to be negligible as a result of partial entrainment of collected particles into the fibrous mat of the quartz fiber filter collection substrate (Chang et al. Citation1999 Chang et al. Citation2001) and the high relative humidity conditions during the sampling periods (∼80% on summer sampling days and ∼70% on winter sampling days) (Stein et al. 1994).
2.2. Sample Analysis
Each quartz fiber substrate was cut into four equal quarters. One quarter was submitted to an aerosol carbon analyzer (Sunset Laboratory, Tigard, OR, USA) to determine the aerosol elemental carbon (EC) and organic carbon (OC) contents. A second quarter was extracted with pure water. The water extract was filtered and analyzed using ion chromatography for major inorganic ions including sulfate, nitrate, chloride, oxalate, Na+, K+, NH+ 4, Ca2+, and Mg2+. The details of the EC, OC and ion analytical methods can be found in the papers by Yu and Yu (Citation2009), Yu et al. (Citation2010), and Yang et al. (Citation2005).
A third quarter was used for analysis of PAHs using a method that couples in-injection port thermal desorption with GC-MS analysis. This method was developed in our lab and details were given in the paper by Ho and Yu (Citation2004). A detailed validation of the method against the traditional solvent-extraction GC-MS method was reported by Ho et al. (2008). Briefly, the quartz filter was cut to strips and inserted into GC liner, where PAHs were thermally desorbed. PAHs subsequently entered GC column for separation and quantification. Twelve PAHs including phenanthrene (PHE), anthracene (ANT), fluoranthene (FLU), pyrene (PYR), chrysene (CHR), benzo[b]fluoranthene (BbF), benzo[k]fluoranthene (BkF), benzo[e]pyrene (BeP), benzo[a]pyrene (BaP), Perylene (PER), indeno[1,2,3-cd]pyrene (IND), and benzo[ghi]perylene (BghiP) were quantified. The limits of detection (LODs) of the twelve PAHs range from 0.087 to 0.31 nanogram per sample. The LODs in air concentrations are 4–14 pg m–3, corresponding to a sampled air volume of 85 m3, i.e., sampling at 30 L min–1 for two days. The minimal concentrations detected in a single size bin ranged from 0.01 to 0.05 ng m–3 for different PAHs, and the maximal concentrations ranged from 0.5 to 14.8 ng m–3.
3. Results and Discussion
Size-segregated concentrations of PAHs, EC, OC, sulfate, and potassium in MOUDI size bins were inverted to continuous lognormal distributions. Mass median aerodynamic diameter (MMAD), geometric standard deviation (σ g ) and mass concentration (Cm ) of each size mode were retrieved by the inversion technique described by Dong et al. (Citation2004). The mean size distributions of PAHs, EC, OC, sulfate, and K+ are shown in for the BG summer samples and in for the HKUST winter samples. The sum of concentrations in all size bins from 0.056 to 18 μm of a species (e.g., individual PAHs, EC, OC, sulfate, potassium) are also listed in Figures and . tabulates the modal concentrations of the total 3-4 ring and the total 5–6 ring PAHs in individual samples. The modal concentrations for a GZ urban site are also included for comparison. More details of the GZ urban data are provided in the supplemental information document and will be reported in a separate paper (Yu and Yu, 2010).
FIG. 2 Average size distributions of PAHs, EC, OC, sulfate, and K+ in the BG summer downwind samples. (Figure provided in color online.)
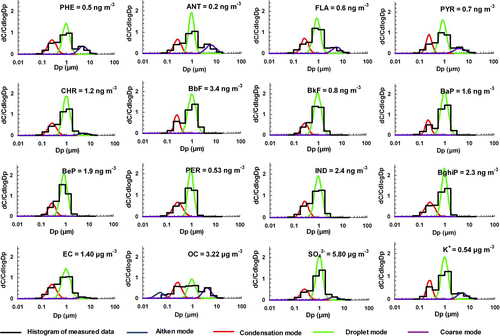
FIG. 3 Average size distributions of PAHs, EC, OC, sulfate, and K+ in the HKUST winter downwind samples. (Figure provided in color online.)
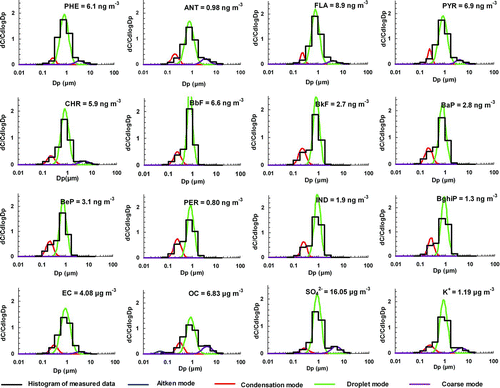
TABLE 1 Modal concentrations (ng m−3) of total 3–4 ring PAHs at a summer downwind site (BG), a winter downwind site (HKUST) in the Pearl River Delta region
3.1. PAH Sources and Distribution Profiles
PAHs are products of incomplete combustion. Important contributing sources include vehicle exhaust, biomass burning, coal combustion, and cooking. Previous studies on PAHs showed that vehicular exhaust was the major source of PAHs in the urban atmosphere of the PRD (Li et al. 2006; Tan et al. 2006), in line with the large number of vehicles in this rapidly developing economic region. Biomass burning, in the forms of open field burning of crop residues in the harvest season and biofuel burning for cooking, occurs widely in the PRD rural areas. Residential coal heating is popular in North China, but is not necessary in a subtropical region like the PRD. Picene, a tracer of coal burning, was detected in only 2 of the 12 winter samples in Hong Kong, whereas picene was detected in nearly all PM2.5 samples during four seasons in Beijing (Zheng et al. 2006). The north–south contrast in picene concentrations reflects the north–south difference in domestic coal combustion as an aerosol source. PAH emission rates from other sources like cooking and natural gas burning are much smaller than those from vehicles and from biomass burning, although they can not be excluded from the source list (Schauer et al. Citation1996).
The particle-phase PAH profile collected at the summer downwind site (BG) was found to be very similar to that at urban GZ (). At both BG and urban GZ locations, the total PAH mass was dominated by five- and six-ring PAHs (called PAH5-6 hereafter). PAH5-6 accounted for 88% of the total PAHs in urban GZ and 81% at the BG site.
FIG. 4 Comparison of PAH profiles at the urban Guangzhou, summer downwind site (BG) and winter downwind site (HKUST).
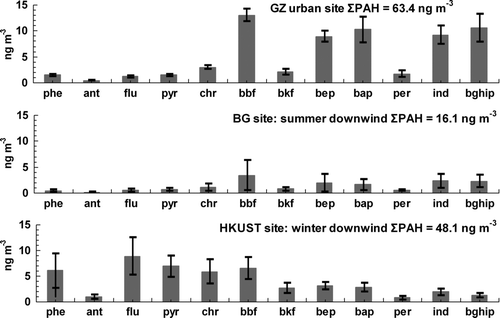
In an effort to identify the possible sources of PAHs at our sampling sites, the correlations of individual PAHs with the measured major aerosol constituents including EC, OC, and ionic species in fine particles (i.e., in particles in the size range of 0.056–1.8 μm) were examined. Among the major constituents we have analyzed, EC indicates contributions of combustion sources; OC has major contributions from both primary emissions from combustion sources and secondary formation contribution; K+ is a biomass burning tracer; sulfate, nitrate, oxalate, and ammonium are secondary aerosol constituents; and Ca2+ are linked with dust particles. The correlation coefficients of PAHs with K+, EC, OC, and sulfate are shown in . The correlations of PAHs with nitrate, oxalate, and NH+ 4 are poor (not shown), similar to the correlations with sulfate. The correlations with Ca2+ are also poor (not shown). At 99% confidence level, all PAHs are correlated with EC and OC in the combined data sets of GZ urban and BG summer samples. This implies that PAHs had common sources with EC and OC in urban GZ and its downwind site in summer.
TABLE 2 Summary of correlation coefficients of various PAHs with EC, OC, K+, and sulfate on fine particles (0.056–1.8 μm)
In general, EC can be emitted from all combustion sources and therefore is not a unique tracer for vehicular emissions; however, previous field measurement studies and source apportionment analysis in this region have indicated that vehicular exhaust is the dominant source of EC in urban areas of this region (e.g., Yu et al. Citation2004; Yuan et al. 2006; Hagler et al. 2006). Yuan et al. (2006) conducted source apportionment using positive matrix factorization analysis of PM10 chemical composition data and found that EC in Hong Kong was mainly from vehicular emissions. The spatial and temporal variation patterns observed in field measurements in Hong Kong also support this conclusion (e.g., Yu et al. Citation2004; Hagler et al. 2006). Contributions from other combustion sources (e.g., biomass burning, coal combustion, cooking) are minor to ambient EC loadings in the urban areas in the region. Considering that EC could be regarded as a tracer for vehicle emissions in this region (Hagler et al 2006), we speculate that vehicle exhaust transported from the upwind urban areas was the major PAH source in the summer downwind site. The PAH concentration contrast between urban GZ (ΣPAH: 63.4 ng m–3) and the rural site BG (ΣPAH: 16.1 ng m–3) also supports the source–receptor relationship between the two sites.
The PAH distribution profile in the winter downwind samples (HKUST) was very different from those in the urban GZ and BG summer samples (). The most prominent difference is the much higher relative abundance of the more volatile PAHs (i.e., three- and four-ring PAHs, called PAH3–4 hereafter) in the HKUST winter samples. PAH3–4 accounted for 60% in the HKUST winter samples while the percentage was 19% in the BG summer samples and 12% in the GZ summer samples. Contrary to the GZ and BG summer samples, PAHs have better correlations with K+ than with EC, OC, and sulfate in the HKUST winter samples (). Potassium is a known tracer of biomass burning emissions (Chow and Watson 2007). Crop residue burning in the field and as cooking fuels is estimated to be the main form biomass burning in the PRD (Streets et al. 2003; Lin et al. Citation2010). The positive correlations of PAHs with K+ suggest that PAHs came from the same source region as K+. In another words, biomass burning emissions and other regional combustion sources are dominant sources for PAHs at the winter downwind site.
The difference in relative abundance of PAH3-4 and PAH5-6 between the two receptor sites is possibly a combined result of different PAH sources impacting the two sites and difference in PAH3−4 partitioning between gas phase and aerosol phase due to temperature change in summer and winter. A number of studies have reported ambient PAH levels on biomass burning days or PAHs emission factors from various agricultural fuels. Some found volatile PAHs dominated the PAH profiles in the source aerosols (Venkataraman et al. 2002; Hays et al. Citation2005; Conde et al. Citation2005; Yang et al. 2006; Keshtkar and Ashbaugh Citation2007), whereas others found nonvolatile PAHs were more abundant (Dhammapala et al. Citation2007; Anttila et al. 2008; Kleeman et al. Citation2008; Wang et al. 2008). Most likely, PAH profiles in biomass burning emissions depend on biomass fuel types and combustion conditions. Our lab collected rice straw and sugarcane burning smoke particles at a rural site of the PRD (Lin et al. Citation2010). Chemical analysis showed that the aerosols produced during rice straw smoldering contain little EC, but high levels of OC, K+, and 3–4 ring PAHs. In comparison, the aerosols produced during the flaming burning of sugarcane contain high EC, but low OC, K+, and 3–4 ring PAHs. This difference can be explained by fuel-dependent conversion efficiency from OC to EC during combustion processes. Because rice straw is the most abundant and the most used biomass fuel in the PRD region, high fraction of PAH3–4 in the winter downwind samples could be partly attributed to rice straw burning emissions.
3.2. Size Distributions of PAHs
The size distributions of individual PAHs at the two receptor sites are shown in Figures and . Although the samples were collected at different sites and in different seasons, the size distribution patterns of aerosol components, including individual PAHs, EC, OC, sulfate, and K+, show little difference between the two sites. However, a significant difference was observed between the size distributions of PAH5–6 and PAH3–4. The PAH5−6 group was mainly distributed in the condensation mode (MMAD: 0.2–0.3 μm, σ g : 1.2–1.6) and droplet mode (MMAD: 0.7–1.0 μm, σ g : 1.2–1.6), with little presence in the coarse mode. On average, the droplet mode accounted for 77% and 71% of PAH5−6 in the winter downwind samples at HKUST and in the summer downwind samples at BG, respectively (). The PAH3−4 group had three significant modes: condensation mode, droplet mode, and coarse mode (MMAD: 3–5 μm, σ g : 1.4–1.8). The coarse-mode size range in this study is 3–10 μm. While the droplet mode was still the most prominent mode (83% of total PAHs in the winter downwind samples and 61% in the summer downwind samples), a significant presence was observed in the coarse mode (6% in the winter downwind samples and 13% in the summer downwind samples).
In comparison with PAH size distributions at the urban GZ site, four major differences are identified at the receptor sites. First, the dominant size mode shifted from ∼0.4 μm condensation mode at the urban site to 0.7–1.0 μm droplet mode at the two receptor sites. Second, 3–5% of PAH5–6 was observed in the coarse mode in GZ urban samples while PAH5–6 was not detected in the coarse mode at the receptor sites. Third, the fraction of PAH3–4 in the coarse mode decreased from ∼20% in GZ urban samples to 6% and 13% at the receptor sites.
In other parts of the world, Allen et al. (1996), Miguel et al. (Citation2004), Eiguren-Fernandez et al. (2003), and Offenberg and Baker (Citation1999) compared PAH size distributions between rural sites and the nearby urban sites. The study in the downwind Los Angeles by Miguel and coworkers (Eiguren-Fernandez et al. 2003; Miguel et al. Citation2004) and the study in the downwind of Boston by Allen et al. (1996) found that both volatile and non-volatile PAHs were associated with the coarse particles to a greater extent than at their respective upwind urban sites. Opposite observation is reported by Offenberg and Baker (Citation1999). In their study, both high and low molecular weight PAHs were more evenly distributed to fine and coarse particles in urban Chicago, while at a downwind overwater station in Michigan Lake, PAHs resided mostly in the droplet mode size range (0.45–1.4 μm size) and no coarse-mode PAHs were detected.
3.2.1. PAHs in the Droplet Mode
It takes about 2–3 h for an air parcel to move from the PRD source areas to the downwind sites (Zhang and Zhang 2001). In our observations of the summer downwind samples, 73% of fine mode K+ resided in the droplet mode, while 66% of EC resided in the droplet mode. In winter downwind samples, both K+ and EC have a mass fraction of 85% in the droplet mode.
A theoretical calculation by Huang et al. (Citation2006b) has indicated that freshly emitted EC particles at ∼0.4 μm are effective cloud condensation nuclei (CCN) at atmospherically relevant critical supersaturation values of less than 1%. Once soot particles act as CCN in a cloud event to form cloud droplets, sulfate and secondary organic materials are subsequently produced as a result of cloud aqueous chemistry. After water evaporation from the cloud droplets, new sulfate particles with soot or K+ core are formed and the resulting particles are in the droplet-mode size. This mechanism of droplet-mode aerosol formation is demonstrated in a number of studies in the literature (e.g., Meng and Seinfeld 1994; Kerminen and Wexler Citation1995; Huang et al Citation2006b; Huang and Yu Citation2008). Meng and Seinfeld (1994) showed through a numerical analysis that in-cloud formation was the only possible mechanism for the growth of condensation mode sulfate to droplet-mode sulfate. Huang and Yu (Citation2008) performed a similar theoretical analysis of growth processes of EC particles and concluded that in-cloud processing was the only plausible mechanism to grow EC particles to the 0.56–1.0 μm size bin (i.e., the droplet mode size) in the atmosphere of Shenzhen, a mega-city in the PRD. Other mechanisms like coagulation, H2SO4 condensation, and hygroscopic growth are too slow to account for the significant growth of EC particles to this size.
Cloud events are common in our study region. The average cloud cover in this coastal region is about 70% in summer and 78% in winter (www.hko.gov.hk). The commonly observed low clouds in this region include strato and stratocumulus clouds. The typical height of low stratus clouds is from 250 to 500 m, which is generally lower than the mixing height (Meng and Seinfeld 1994).
PAHs are precursors and intermediates in soot formation (Dobbins et al. 1998). Particle-phase PAHs co-emit with vehicle soot particles or biomass-burning soot particles by adsorbing onto particle surface. Naturally, PAHs reside in the same size as soot particles when they are freshly emitted (Venkataraman and Friedlander Citation1994; Yu and Yu 2010). The PAHs retained on the soot particle phase undergo the same atmospheric processing (e.g., cloud processing) as their parent soot particles. This is supported by the same size range of droplet mode that PAHs exhibit as EC and sulfate. Following the analysis of the origin of the droplet mode EC, we speculate that the dominant mode of PAH5–6 and PAH3–4 in the size mode of MMAD at 0.7–1.0 μm at the two receptor sites could be attributed to in-cloud processing of soot particles or biomass burning particles. Dominance of a droplet-mode in size distributions of PAHs was also observed in winter samples in Seoul, South Korea, during which air pollution transported from North Korea and China impacted Seoul (Lee et al. 2008).
One important result of in-cloud processing is the formation of secondary organic aerosol (SOA) and sulfate coating in the droplet mode. The absorptive ability of organic matter (OM) not only depends on OM abundance (i.e., f om, see Equation (Equation1) below), but also is sensitive to the nature of OM (i.e., activity coefficient γ om , Equation (Equation1)). Under high relative humidity condition, the droplet-mode OM likely exists in two phases: aqueous phase and organic phase. The activity coefficient of PAHs in water is about 106 times that in OM (Harner and Bidleman 1998). In another words, aqueous phase virtually has no absorbing ability for PAHs. On the other hand, it is likely that sulfate coating on the droplet-mode particles traps PAHs inside the particle. Little new gas-phase PAHs would partition to the particle cores, and PAHs originally sorbed on the particle surface are prevented from volatilizing to the gas phase and are inaccessible to oxidation by gaseous oxidants.
We did not measure the gas-phase concentrations of PAHs. As a result, a direct assessment of the gas-particle partitioning equilibrium state is not possible. However, how further PAHs in the droplet size mode deviate from the equilibrium state in comparison with PAHs in the condensation mode can be inferred from the ratios of PAH concentrations in the two size modes, since PAHs on particles in different mode sizes equilibrate with a common gas-phase. The size-specific gas-particle partitioning coefficients of PAHs can be estimated based on EC adsorption and OM absorption using Pankow's model (Pankow 1987; Pankow 1994). The concentration of a PAH in size mode i (Ci p ) is therefore expressed by Equation (Equation1) given below:
An estimation of the relative abundance of PAH3−4 in the droplet mode and the condensation mode at equilibrium can be made using Equation (Equation1). This equation shows that the relative abundance of PAH3−4 in two size modes under equilibrium state should be proportional to the sorption ability of particles in the respective modes. EC and OM concentrations (Ci EC and Ci OM ) were measured in this study. EC surface area density can be estimated assuming that the condensation-mode EC particles are spherical particles of diameter 0.26 μm and the droplet-mode EC originates from spherical EC particles of diameter 0.42 μm, on the basis of observations in the PRD urban areas, followed by in-cloud processing and cloud evaporation (Yu and Yu Citation2009; Huang et al. Citation2006b). Ns is taken to be 4 × 10−10 mol cm−2 (Pankow 1987); the difference between desorption enthalpy and vaporization enthalpy Q L -Q v ≈ 3 kcal mol−1 is extracted from Pankow (1987) and experimentally confirmed by Aubin and Abbatt (Citation2006) and Guilloteau et al. (Citation2010). The mean MW om is set to 200 g/mol (Pankow 1994). The γ om values are approximated using activity coefficients in octanol. The calculated results show that PAH equilibrium concentrations in the droplet mode should be ∼3.9 times (winter samples) and ∼1.2 times (summer samples) of those in the condensation mode. The observed concentration ratios between the two modes, however, are 7.8 in the winter and 2.2 in the summer for PHE, ANT, FLU, PYR, and CHY. These volatile PAH3-4 compounds in the condensation mode particles (without an aqueous sulfate coating) can partition freely from particle phase to gas phase when the gas phase concentration decreases due to dilution or photo-oxidation degradation. Thus, it is a reasonable approximation that the PAH3-4 compounds in the condensation mode are in equilibrium with their gas-phase. The excessive PAH3-4 in the droplet mode, relative to their expected equilibrium concentrations, thus indicates that the PAHs in the droplet mode have not achieved equilibrium with gas phase.
The non-equilibrium between the droplet mode and the gas phase can be regarded that the sulfate aqueous coating in effect preserves the PAHs. It in turns implies that the atmospheric life time of droplet-mode PAHs would be longer and more of them could be transported to downwind receptor sites than PAHs residing on other particle size modes. Another implication of the aqueous barrier for PAHs is that more PAHs would be found in the particle phase than predicted by the gas-particle equilibrium theory. More gas-particle partitioning data is needed to support the speculations arising from the size distribution observations in this study.
3.2.2. PAHs in the Coarse Mode
Because coarse particles deposit faster than fine particles, coarse-mode PAHs are believed to result from partitioning from gas phase to locally generated coarse particles. Allen et al. (1996), Miguel et al. (Citation2004), and Eiguren-Fernandez et al. (2003) attributed the increase of coarse mode PAHs in downwind sites to that all PAHs distributed more closely toward equilibrium on time scales relevant to regional transport. But obviously the equilibrium partitioning alone could not explain the decrease of coarse-mode PAH3–4 and absence of coarse-mode PAH5–6 at the two receptor sites of the PRD region.
We argue that there are possibly two reasons to explain the observations on coarse-mode PAHs in this study and in the study by Offenberg and Baker (Citation1999). First, gas-to-particle partitioning depends on not only equilibrium time, but also the abundance of PAH sorbents on coarse particles. In GZ urban atmosphere, the presence of coarse-mode PAH3−4 can be explained by adsorption by EC materials on soil or dust particles, where 20% of EC mass resided (Yu and Yu 2010). In comparison, less EC sorbent was available on the coarse particles in the rural atmospheres. Only 4% of EC mass resided in the coarse mode in the receptor site samples. Accordingly, less PAHs could be retained on the coarse particles. Lack of EC sorbent on the coarse particles could also explain the absence of coarse-mode PAHs in the atmosphere above Michigan Lake where the water surface precluded local sources of EC-containing soil and dust (Offenberg and Baker Citation1999).
The second contributing reason is that coarse-mode PAHs are more susceptible to oxidation degradation and volatilization than droplet-mode PAHs. During the transport from source regions to the receptor sites, volatile PAHs can partition onto particles when temperature falls, and they can also partition away from particles when their gas phase concentrations drop due to dilution and degradation. The aqueous coating prevents PAHs sorbed onto EC and OM from partitioning freely between the droplet-mode particles and the gas phase. In addition, the aqueous coating acts as a good barrier to make oxidant species (OH and NO3) less accessible to PAHs in soot cores. In comparison, the coarse-mode PAHs, without such an aqueous coating, are expected to be depleted faster than the PAHs trapped in the droplet mode particles.
4. Conclusions
The size distributions of twelve PAHs, together with EC, OC, sulfate, and potassium, were measured in the period of 2006–2008 at one rural and one suburban locations in the Pearl River Delta, China. At the time the samples were collected, these two locations are receptor sites of urban centers in the region, providing us opportunities to study the characteristics of particle aging processes when air mass moves away from the source regions of the PRD. Correlation analyses of PAHs with source tracers indicate that PAHs at the summer downwind site were mainly derived from vehicular emissions in urban areas while PAHs at the winter downwind location was strongly influenced by regional sources such as biomass burning.
The size distributions of three- and four-ring PAHs are characterized by significant presence in three modes: condensation mode (MMAD: 0.2–0.3 μm), droplet mode (MMAD: 0.7–1.0 μm) and coarse mode (MMAD: 3–5 μm). The size distributions of five- and six-ring PAHs consist of the first two modes, with the coarse mode undetected. The droplet mode is the most prominent mode for both PAH3–4 and PAH5–6. We have deduced that PAHs in the droplet-mode size did not achieve equilibrium state because the sulfate coating has a trapping effect on PAHs inside the droplet mode particles. This aqueous coating could prevent PAHs from partitioning freely from droplet mode particles to gas phase and make PAHs less susceptible to oxidation degradation. Further implications include: (Equation1) the lifetime of PAHs in the droplet-mode particles could be longer than those in the gas phase or on particles in the condensation mode and coarse mode; and (2) more PAHs would be found in the particle phase than predicted by gas-particle equilibrium theory. Future studies are suggested to investigate partitioning behaviors of PAHs between gas-phase and the droplet-mode particle-phase to further our understanding of PAHs in the atmosphere.
Supplemental Files.zip
Download Zip (59.7 KB)Acknowledgments
This work was partially supported by the Research Grant Council of Hong Kong, China (621405). We thank Peking University for organizing the PRIDE-PRD2006 campaign, which was mainly sponsored by China National Basic Research and Development Program-2002CB410801 and 2002CB211605.
REFERENCES
- Allen , J. O. , Dookeran , N. M. , Smith , K. A. , Sarofim , A. F. , Taghizadeh , K. and Lafleur , A. L. 1996 . Measurement of Polycyclic Aromatic Hydrocarbons Associated with Size-Segregated Atmospheric Aerosols in Massachusetts . Environ. Sci. Technol. , 30 ( 3 ) : 1023 – 1031 .
- Andreae , M. O. , Schmid , O. , Yang , H. , Chand , D. , Yu , J. Z. , Zeng , L. M. and Zhang , Y. H. 2008 . Optical Properties and Chemical Composition of the Atmospheric Aerosol in Urban Guangzhou, China . Atmos. Environ. , 42 : 6335 – 6350 .
- Anttila , P. , Makkonen , U. , Hellen , H. , Kyllonen , K. , Leppanen , S. , Saari , H. and Hakola , H. 2008 . Impact of the Open Biomass Fires in Spring and Summer of 2006 on the Chemical Composition of Background Air in South-Eastern Finland . Atmos. Environ. , 42 : 6472 – 6486 .
- Aubin , D. G. and Abbatt , J. P. 2006 . Laboratory Measurements of Thermodynamics of Adsorption of Small Aromatic Gases to n-Hexane Soot Surfaces . Environ. Sci. Technol. , 40 : 179 – 187 .
- Bi , X. , Sheng , G. , Peng , P. , Chen , Y. and Fu , J. 2005 . Size Distribution of N-Alkanes and Polycyclic Aromatic Hydrocarbons (PAHs) in Urban and Rural Atmospheres of Guangzhou, China . Atmos. Environ. , 39 : 477 – 487 .
- Cao , J. J. , Lee , S. C. , Ho , K. F. , Zou , S. C. , Fung , K. , Li , Y. , Watson , J. G. and Chow , J. C. 2004 . Spatial and Seasonal Variations of Atmospheric Organic Carbon and Elemental Carbon in Pearl River Delta Region, China . Atmos. Environ. , 38 : 4447 – 4456 .
- Chang , M. , Kim , S. and Sioutas , C. 1999 . Experimental Studies on Particle Impaction and Bounce: Effects of Substrate Design and Material . Atmos. Environ. , 15 : 2313 – 2323 .
- Chang , M. C. , Sioutas , C. , Fokkens , P. B. and Cassee , F. R. 2001 . Field Evaluation of a Mobile High-Capacity Particle Size Classifier (HCPSC) for Separate Collection of Coarse, Fine, and Ultrafine Particles . J. Aerosol Sci. , 32 : 139 – 156 .
- Chow , J. C. and Watson , J. G. 2007 . Review of Measurement Methods and Compositions for Ultrafine Particles . Aerosol Air Qual. Res. , 7 ( 2 ) : 121 – 173 .
- Conde , F. J. , Ayala , J. H. , Afonso , A. M. and Gonzalez , V. 2005 . Emissions of Polycyclic Aromatic Hydrocarbons from Combustion of Agricultural and Sylvicultural Debris . Atmos. Environ. , 39 : 6654 – 6663 .
- Dhammapala , R. , Claiborn , C. , Jimenez , J. , Corkill , J. , Gullett , B. , Simpson , C. and Paulsen , M. 2007 . Emission Factors of PAHs, Methoxyphenols, Levoglucosan, Elemental Carbon, and Organic Carbon from Simulated Wheat and Kentucky Bluegrass Stubble Burns . Atmos. Environ. , 41 : 2660 – 2669 .
- Dobbins , R. A. , Fletcher , R. A. and Chang , H. C. 1998 . The Evolution of Soot Precursor Particles in a Diffusion Flame . Combust. Flame , 115 ( 3 ) : 285 – 298 .
- Dong , Y. J. , Hays , M. D. , Smith , N. D. and Kinsey , J. S. 2004 . Inverting Cascade Impactor Data for Size-Resolved Characterization of Fine Particulate Source Emissions . J. Aerosol. Sci. , 35 : 1497 – 1512 .
- Duan , J. , Tan , J. , Cheng , D. , Bi , X. , Deng , W. , Sheng , G. , Fu , J. and Wong , M. H. 2007 . Sources and Characteristics of Carbonaceous Aerosol in (of the?) Two Largest Cities in Pearl River Delta Region, China . Atmos. Environ. , 41 : 2895 – 2903 .
- Eiguren-Fernandez , A. , Miguel , A. H. , Jaques , P. A. and Sioutas , C. 2003 . Evaluation of a Denuder-MOUDI-PUF Sampling System to Measure the Size Distribution of Semi-Volatile Polycyclic Aromatic Hydrocarbons in the Atmosphere . Aerosol Sci. Technol. , 37 ( 3 ) : 201 – 209 . doi: 10.1080/02786820300943
- Gnauk , T. , Müller , K. , Van Pinxteren , D. , He , L. Y. , Niu , Y. , Hu , M. and Herrmann , H. 2008 . Size-Segregated Particulate Chemical Composition in Xinken, Pearl River Delta, China:OC/EC and Organic Compounds . Atmos. Environ. , 42 ( 25 ) : 6296 – 6309 .
- Guilloteau , A. , Bedjanian , Y. , Nguyen , M. L. and Tomas , A. 2010 . Desorption of Polycyclic Aromatic Hydrocarbons from a Soot Surface: Three- to Five-Ring PAHs . J. Phys. Chem. A , 114 : 942 – 948 .
- Hagler , G. S. W. , Bergin , M. H. , Salmon , L. G. , Yu , J. Z. , Wan , E. C. H. , Zheng , M. , Zeng , L. M. , Kiang , C. S. , Zhang , Y. H. , Lau , A. K. H. and Schauer , J. J. 2006 . Source Areas and Chemical Composition of Fine Particulate Matter in the Pearl River Delta Region of China . Atmos. Environ. , 40 ( 20 ) : 3802 – 3815 .
- Harner , T. and Bidleman , T. F. 1998 . Octanol-Air Partition Coefficient for Describing Particle/Gas Partitioning of Aromatic Compounds in Urban Air . Environ. Sci. Technol. , 32 ( 10 ) : 1494 – 1502 .
- Hays , M. , Fine , P. M. , Geron , C. D. , Kleeman , M. and Gullett , B. K. 2005 . Open Burning of Agricultural Biomass: Physical and Chemical Properties of Particle-Phase Emissions . Atmos. Environ. , 39 : 6747 – 6764 .
- Ho , S. S. H. and Yu , J. Z. 2004 . In-Injection Port Thermal Desorption and Subsequent Gas Chromatography-Mass Spectrometric Analysis of Polycyclic Aromatic Hydrocarbons and N-Alkanes in Atmospheric Aerosol Samples . J. Chromatogr. , 1059 : 121 – 129 .
- Ho , S. S. H. , Yu , J. Z. , Chow , J. C. , Watson , J. G. , Zielinska , B. , Sit , E. H. L. and Schauer , J. J. 2008 . Evaluation of an In-Injection Port Thermal Desorption GC-MS Method for Analysis of Non-Polar Organic Compounds in Ambient Aerosol Samples . J. Chromatogr. , 1200 ( 2 ) : 217 – 227 .
- Huang , X. F. , Yu , J. Z. , He , L. Y. and Yuan , Z. 2006a . Water-Soluble Organic Carbon and Oxalate in Aerosols at a Coastal Urban Site in China: Size Distribution Characteristics, Sources, and Formation Mechanisms . J. Geophys. Res. , 111 : D22212 doi: 10.1029/2006JD007408
- Huang , X. F. , Yu , J. Z. , He , L. Y. and Hu , M. 2006b . Size Distribution Characteristics of Elemental Carbon Emitted from Chinese Vehicles: Results of a Tunnel Study and Atmospheric Implications . Environ. Sci. Technol. , 40 : 5355 – 5360 .
- Huang , X. F. and Yu , J. Z. 2008 . Size Distributions of Elemental Carbon in the Atmosphere of a Coastal Urban Area in South China:Characteristics, Evolution Processes, and Implications for the Mixing State . Atmos. Chem. Phys. , 8 : 5843 – 5853 .
- Kaupp , H. and Mclachlan , M. S. 2000 . Distribution of Polychlorinated Dibenzo-p-Dioxins and Dibenzofurans (PCDD/Fs) and Polycyclic Aromatic Hydrocarbons (PAHs) within the Full Size Range of Atmospheric Particles . Atmos. Environ. , 34 ( 1 ) : 73 – 83 .
- Kerminen , V.-M. and Wexler , A. S. 1995 . Growth Laws for Atmospheric Aerosol Particles: An Examination of the Bimodality of the Accumulation Mode . Atmos. Environ. , 29 : 3263 – 3275 .
- Keshtkar , H. and Ashbaugh , L. L. 2007 . Size Distribution of Polycyclic Aromatic Hydrocarbon Particulate Emission Factors from Agricultural Burning . Atmos. Environ. , 41 : 2729 – 2739 .
- Kleeman , M. J. , Michael , A. R. , Riddle , S. G. , Fine , P. M. , Hays , M. D. , Schauer , J. J. and Hannigan , M. P. 2008 . Size Distribution of Trace Organic Species Emitted from Biomass Combustion and Meat Charbroiling . Atmos. Environ. , 42 : 3059 – 3075 .
- Lee , J. Y. , Shin , H. J. , Bae , S. Y. , Kim , Y. P. and Kang , C. H. 2008 . Seasonal Variation of Particle Size Distributions of PAHs at Seoul, Korea . Air Qual Atmos Health , 1 : 57 – 68 . doi: 10.1007/S11869-008-0002-2
- Li , J. , Zhang , G. , Li , X. D. , Qi , S. H. , Liu , G. Q. and Peng , X. Z. 2006 . Source Seasonality of Polycyclic Aromatic Hydrocarbons (PAHs) in a Subtropical City, Guangzhou, South China . Sci. Total Environ. , 355 ( 1-3 ) : 145 – 155 .
- Lin , P. , Engling , G. and Yu , J. Z. 2010 . Humic-Like Substance in Fresh Emissions of Rice Straw Burning and in Ambient Aerosols in the Pearl River Delta Region, China . Atmos. Chem. Phys. , 10 : 6487 – 6500 .
- Meng , Z. Y. and Seinfeld , J. H. 1994 . On the Source of the Submicrometer Droplet Mode of Urban and Regional Aerosols . Aerosol Sci. Technol. , 20 ( 3 ) : 253 – 265 .
- Miguel , A. H. , Eiguren-Fernandez , A. , Jaques , P. A. , Froines , J. R. , Grant , B. L. , Mayo , P. R. and Sioutas , C. 2004 . Seasonal Variation of the Particle Size Distribution of Polycyclic Aromatic Hydrocarbons and of Major Aerosol Species in Claremont, California . Atmos. Environ. , 38 : 3241 – 3251 .
- Offenberg , J. H. and Baker , J. E. 1999 . Aerosol Size Distributions of Polycyclic Aromatic Hydrocarbons in Urban and Over-Water Atmospheres . Environ. Sci. Technol. , 33 : 3324 – 3331 .
- Pankow , J. F. 1987 . Review and Comparative Analysis of the Theories on Partitioning between the Gas and Aerosol Particulate Phases in the Atmosphere . Atmos. Environ. , 21 ( 11 ) : 2275 – 2283 .
- Pankow , J. F. 1994 . An Absorption Model of Gas/Particle Partitioning of Organic Compounds in the Atmosphere . Atmos. Environ. , 28 ( 2 ) : 185 – 188 .
- Schauer , J. J. , Rogge , W. F. , Hildemann , L. , Mazurek , M. A. and Simoneit , B. R. T. 1996 . Source Apportionment of Airborne Particulate Matter Using Organic Compounds as Tracers . Atmos. Environ. , 30 : 3837 – 3855 .
- Stein , S. W. , Turpin , B. J. , Cai , X. , Huang , P. F. and Mcmurry , P. H. 1994 . Measurements of Relative Humidity-Dependent Bounce and Density for Atmospheric Particles Using the DMA-Impactor Technique . Atmos. Environ. , 28 : 1739 – 1746 .
- Streets , D. G. , Yarber , K. F. , Woo , J. H. and Carmichael , G. R. 2003 . Biomass Burning in Asia: Annual and Seasonal Estimates and Atmospheric Emissions . Global Biogeochem. Cy. , 17 : 1099 doi: 10.1029/2003GB002040
- Tan , J. H. , Bi , X. H. , Duan , J. C. , Rahn , K. A. , Sheng , G. Y. and Fu , J. M. 2006 . Seasonal Variation of Particulate Polycyclic Aromatic Hydrocarbons Associated with PM10 in Guangzhou, China . Atmos. Res. , 80 ( 4 ) : 250 – 262 .
- Venkataraman , C. and Friedlander , S. K. 1994 . Size Distributions of Polycyclic Aromatic Hydrocarbons and Elemental Carbon: Part 2: Ambient Measurements and Effects of Atmospheric Processes . Environ. Sci. Technol. , 28 : 563 – 572 .
- Venkataraman , C. , Negi , G. , Sarda , S. B. and Rastogi , R. 2002 . Size Distributions of Polycyclic Aromatic Hydrocarbons in Aerosol Emissions from Biofuel Combustion . J. Aerosol. Sci. , 33 ( 3 ) : 503 – 518 .
- Wang , H. L. , Zhuang , Y. H. , Hao , Z. P. , Cao , M. Q. , Zhong , J. X. , Wang , X. K. and Nguyen , T. K. O. 2008 . Polycyclic Aromatic Hydrocarbons from Rural Household Biomass Burning in a Typical Chinese Village . Science In China Series D: Earth Sciences. , 51 ( 7 ) : 1013 – 1020 .
- Wu , S. P. , Tao , S. and Liu , W. X. 2006 . Particle Size Distributions of Polycyclic Aromatic Hydrocarbons in Rural and Urban Atmosphere of Tianjin, China . Chemosphere. , 62 ( 3 ) : 357 – 367 .
- Yang , H. H. , Tsai , C. H. , Chao , M. R. , Su , Y. L. and Chien , S. M. 2006 . Source Identification and Size Distribution of Atmospheric Polycyclic Aromatic Hydrocarbons During Rice Straw Burning Period . Atmos. Environ. , 40 ( 7 ) : 1266 – 1274 .
- Yang , H. , Yu , J. Z. , Ho , S. S. H. , Xu , J. , Wu , W. S. , Wan , C. H. , Wang , X. and Wang , L. 2005 . The Chemical Composition of Inorganic and Carbonaceous Materials in PM2.5 in Nanjing, China . Atmos. Environ. , 39 : 3735 – 3749 .
- Yu , H. and Yu , J. Z. 2009 . Modal Characteristics of Elemental and Organic Carbon in an Urban Location in Guangzhou, China . Aerosol Sci. Technol. , 43 : 1108 – 1118 .
- Yu , H. , Wu , C. , Wu , D. and Yu , J. Z. 2010 . Size Distributions of Elemental Carbon and its Contribution to Light Extinction in Urban and Rural Locations in the Pearl River Delta Region, China . Atmos. Chem. Phys. , 10 : 5107 – 5119 .
- Yu , H. and Yu , J. Z. 2010 . Polycyclic Aromatic Hydrocarbons in Urban Atmosphere of Guangzhou, China:Size Distribution Characteristics and Size-Resolved Gas-particle Partitioning in preparation
- Yu , J. Z. , Tung , J. W. T. , Wu , A. W. M. , Lau , A. K. H. , Louie , P. K. K. and Fung , J. C. H. 2004 . Abundance and Seasonal Characteristics of Elemental and Organic Carbon in Hong Kong PM10 . Atmos. Environ. , 38 : 1511 – 1521 .
- Yuan , Z. B. , Lau , A. K. H. , Zhang , H. Y. , Yu , J. Z. , Louie , P. K. K. and Fung , J. C. H. 2006 . Identification and Spatiotemporal Variations of Dominant Sources of PM10 in Hong Kong . Atmos. Environ. , 40 : 1803 – 1815 .
- Zhang , Q. Y. and Zhang , Y. 2001 . “ Research on Atmospheric Boundary Layer and Pollution of Chinese Cities ” . In Research Progress on Urban Environmental Meteorology in China and Overseas. issue 2
- Zhang , X. Q. and McMurry , P. H. 1991 . Theoretical Analysis of Evaporative Losses of Adsorbed or Absorbed Species during Atmospheric Aerosol Sampling . Environ. Sci. Technol. , 25 ( 3 ) : 456 – 459 .
- Zheng , M. , Hagler , G. S. W. , Ke , L. , Bergin , M. H. , Wang , F. , Louie , P. K. K. , Salmon , L. , Sin , D. W. M. , Yu , J. Z. and Schauer , J. J. 2006 . Composition and Sources of Carbonaceous Aerosols at Three Contrasting Sites in Hong Kong . J. Geophy. Res. , 111 : D20313 doi: 10.1029/2006JD007074