Abstract
The development of electrospray technique is restricted due to main drawbacks, mainly because it is restricted to electrically conducting liquids and to low throughputs. To overcome these limitations, we have developed a multi-jet electrospray technique for non-conducting fluids. To obtain stable multi-jet operation, a grooved coaxial nozzle was employed to enhance the exterior electrical field. The multi-jet coaxial electrospray mechanism is identical to a single cone-jet coaxial electrospray, and multi-jet operation for a non-conducting fluid has the same features as a single cone-jet for a conducting liquid. The droplet size can be controlled by adjusting the flow rates, and the droplets monodisperse with good stability and uniformity.
1. INTRODUCTION
Since CitationZeleny's (1914) pioneering studies, electrospray techniques have been widely used in the field of liquid atomization. Analytical descriptions were formulated by CitationTaylor (1964) and the functioning modes of electrospray were described by CitationCloupeau and Prunetfoch (1994). Electrospray can produce monodisperse liquid droplets with good spatial dispersion. Many comprehensive studies have been proposed for practical application of electrospray technique. Electrospray ionization mass spectrometry was introduced by CitationFenn et al. (1989). The use of electrospray for an aerosol generator was reported by CitationChen et al. (1995) The colloid thruster for adjustment of micro-satellite motion was introduced by Gamero-Castano et al. (2010). Furthermore, The miniaturize combustor using electrospray source was introduced by CitationDeng et al. (2007).
However, still there are obstacles to broaden their applications because it results in very low throughputs and is restricted to conducting liquids. Many modifications to the technique have been proposed to overcome these limitations. Electrospray for non-conducting liquids was reported by CitationLoscertales et al. (2002), who showed that a double-layered cone-jet formation occurs when two immiscible liquids are fed coaxially. They reported that tangential electrical stress of the inner liquid can transmit throughout the outer liquid by viscous diffusion; following from this, micro-encapsulation techniques have been developed. Many studies have been carried out with the aim of increasing throughputs. The multi-jet electrospray mode was proposed by CitationDuby et al. (2006), the multiplexed electrospray system (MES) was suggested by CitationDeng et al. (2009), and multiple electrosprays using an array of holes were developed by CitationBocanegra et al. (2005). However, there is no approach that overcomes the two drawbacks simultaneously. This study proposes an electrospray technique that is capable of large throughputs of non-conducting liquids.
The basic concept of the electrospray technique for non-conducting liquids is similar to that reported by CitationLopez-Herrera et al. (2003). The conducting liquid in the inner capillary can be extruded into jets by the stress induced by an electric field. As the electric field strength increases, the number of jets increases. At the end of the inner capillary, there is an interface between the inner and outer liquids. At this interface, there is a tangential stress of inner liquid caused by the electric field, which can penetrate into the outer liquid by viscous diffusion. Therefore, the two liquids can extrude simultaneously. We can control the multi-jet operation using a coaxial grooved nozzle electrospray system and achieve stable electrospray with large throughputs of non-conducting liquids.
2. MATERIALS AND METHODS
The experimental set-up is shown schematically in . The system consisted of three main parts: a coaxial nozzle, a liquid feeding system, and the instruments. Here we describe each of these components.
2.1. Coaxial Nozzle Assembly
In order for the electrospray system to operate with two liquids, a coaxial nozzle was used. Coaxial nozzles have been widely used in the field of micro encapsulation (CitationChen et al. 2005; CitationXie et al. 2008; CitationKim and Kim 2010), where the two liquids are separately fed into the composite nozzle. In this study, in order to achieve stable multi-jet operation, we used a grooved inner nozzle, where the electric field is enhanced at the grooves (CitationDuby et al. 2006). The geometry of stainless steel coaxial nozzle is shown in . The outer nozzle had an outer diameter of 6.350 mm and inner diameter of 4.064 mm. The inner nozzle had an outer diameter of 3.175 mm and inner diameter of 2.032 mm. The six grooves in the inner nozzle were made by wire electro discharge machining (WEDM). Each groove had a width of 0.4 mm and height of 0.5 mm. (It should be noted that, in order to ensure uniform operation and equipartitioning of the jets, the symmetry of the grooves is very important.)
To optimize the interaction of the two liquids and minimize the interference of the liquid meniscus, the inner nozzle was extended 0.5 mm out from end of the outer nozzle. If the two nozzles are terminated at the same level, the menisci are easily submerged by the outer liquid, and operation becomes intermittent. If the inner nozzle extends too far, it becomes difficult to obtain multi-jet formation.
2.2. Liquids and Feeding System
To obtain electrospray with a non-conducting liquid, two liquids must be used; the inner one must be conducting, while the outer one is the non-conducting liquid. We used ethanol (MERCK, Germany) as the inner liquid and olive oil (Junsei Chemical Co., Ltd., Japan) as the outer liquid. Ethanol is widely used in the field of electrospray due to its favorable physical properties. The physical properties of the two liquids are summarized in .
TABLE 1 Physical properties of the fluids
Two syringe pumps were used to control flow rates of the two liquids with high accuracy (KDS-100, KD-Scientific, USA). To prevent the formation of micro bubbles, the liquids were sonicated for a few minutes before loading into the syringes. The syringe needles were connected to the nozzles using Teflon-coated tubing, and the flow rates of the two liquids were controlled using the syringe pumps. The flow rates of ethanol were 4 ml/h and 6 ml/h, and the flow rate of olive oil was varied through (ethanol flow rate of 4 ml/h) 8, 12, 16, and 20 ml/h, and (ethanol flow rate of 6 ml/h) 12, 18, 24, and 30 ml/h. These flow rates are comparable to the high-throughput flow rates used in previous studies (CitationDuby et al. 2006; CitationDeng et al. 2009; CitationBocanegra et al. 2005).
2.3. Electrospray System Set-up and Operation
A high voltage source (Korea Switching, Inc., Korea) was connected to the top of the coaxial nozzle, and a stainless steel mesh ground plate was placed 10 mm below the tip of the nozzle to create a large electric field at the tip of the nozzle. When a droplet reached the ground plate, it passed through the mesh due to gravity, minimizing clogging.
The onset-voltage of the functioning mode depends on the physical liquid properties and flow rates of the liquids. In order to achieve large flow rates, we used the multi-jet mode. To identify multi-jet formation, we observed an exterior feature of jet formation, and measurements were made when the jet became stable.
2.4. Measurements
Measurement of the electrospray system was carried using three methods. Visualization of the spray pattern was conducted using a conventional digital camera (Olympus, Japan) with a high-resolution lens (Nikon, Japan) and light source. Visualization of interior structure of the liquid meniscus was obtained using a charge-coupled device (CCD) array camera (Marlin F-145C2, AVT, Germany) with a high magnification lens. The droplet current was measured using an electrometer (Model 6514, Keithley Instruments, USA), and the real-time size distribution was determined using a phase Doppler particle analyzer (PDPA, TSI Inc., USA).
To obtain a clear image of the structure of the two liquids, a digital camera and a CCD array camera with a high-resolution lens were used. The liquid column was quite thin, making it difficult to observe using ambient light, and so a light source was used and scattered light from the liquid was detected using the cameras. To distinguish the interface of two liquids, the pigment Rhodamine B (Junsei Chemical Co., Ltd., Japan) was added to the ethanol. (There is a slight increase of electrical conductivity adding Rhodamine B. σw/ Rhodamine B = 30.2 μS/m.) We observed the formation of a stable multi-jet spray pattern.
Each droplet had an electrical charge with the same polarity, and so when the droplets reached the ground plate, the charge was transferred. From measurement of this current, we can infer the number of multi-jet formed at the certain flow rates of inner liquids.
FIG. 2 Spray visualization. (a) Qethanol = 4ml/h, Qolive oil = 12ml/h; (b) Qethanol = 4ml/h, Qolive oil = 16 ml/h; (c) Qethanol = 4ml/h, Qolive oil = 20ml/h; (d) Qethanol = 6ml/h, Qolive oil = 18ml/h; (e) Qethanol = 6ml/h, Qolive oil = 24ml/h; (f) Qethanol = 6ml/h, Qolive oil = 30ml/h.
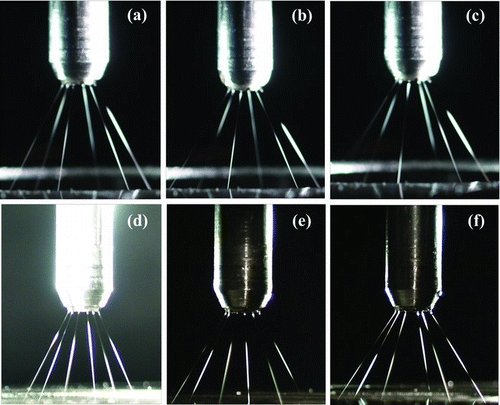
The size distribution of the droplets was measured using the PDPA. When stable multi-jets formed, we measured the size distribution of the droplets 10 mm from the end of the nozzle. To ensure reliable results, each measurement was conducted for 5000 droplets and all working jets.
3. RESULTS AND DISCUSSION
3.1. Multi-Jet Formation and Spray Visualization
Multi-jet formation appeared at larger flow rates than the cone-jet mode. The overall procedure of multi-jet formation for two liquids was identical to multi-jet operation for a single conducting liquid. When a cone-jet formed, further increasing the applied voltage resulted in the single liquid column becoming unstable, and additional liquid columns appeared. With a smooth nozzle, the jet formation is unstable, and the origins of the jets move along the circumference of the nozzle. This makes it hard to control multi-jet operation. However, when a grooved nozzle was used, each jet was anchored at a groove, and stable operation was achieved. The jets were electrically balanced with each other, and six stable jets were observed. Images showing multi-jet operation are presented in .
3.2. Visualization of the Liquid Meniscus
A CCD array camera with a high-magnification lens was used to investigate the structure of the jets. It is important that the two immiscible fluids have an interface along the liquid column.
FIG. 3 The meniscus of the Taylor cone consisted of two liquid layers: (a) Qethanol = 4ml/h, Qolive oil = 12ml/h; (b) Qethanol = 6ml/h, Qolive oil = 18ml/h.
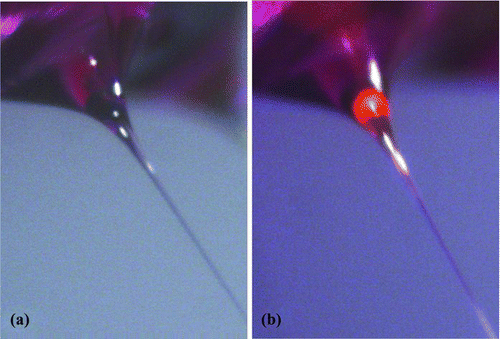
FIG. 5 Current versus voltage for multi-jet operation. (a) Qethanol = 4ml/h, Qolive oil = 12ml/h; (b) Qethanol = 6ml/h, Qolive oil = 18ml/h.
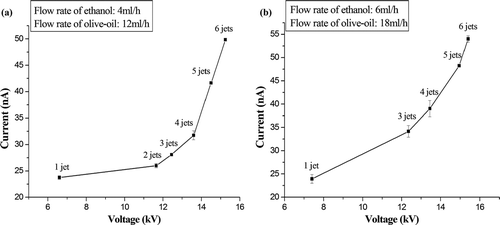
shows images of individual jets, and Taylor cones can clearly be seen at the nozzle. The jets consisted of a double layer of the two liquids along the column. This result supports the electrospray mechanism of the non-conducting fluid. The interface between the two liquids extends a sufficiently long distance that they have enough time to relieve the electrically induced stress of the conducting liquid through the non-conducting liquid by viscous shear forces. This phenomenon was proposed by CitationLopez-Herrera et al. (2003); however, their results were limited to the cone-jet regime. We obtained the same result in the multi-jet regime.
3.3. Functioning Domains of the Multi-jet Spray
The multi-jet mode appeared only under certain applied voltages. As the voltage was increased significantly beyond the onset voltage required for the six stable jets to be formed, the jets became extremely unstable.
shows the voltage required for the onset of the multi-jet mode and for the onset of the unstable regime as a function of the flow rates of the two liquids. The range of voltages at which stable multi-jet operation was observed was approximately 1 kV, and the onset voltage was proportional to the flow rate of the inner liquid (the conducting liquid). As the flow rate of the outer liquid increased, the onset voltage increased slightly, but there was no strong relationship between the onset voltage and the flow rate of the non-conducting liquid.
3.4. Current of Individual Droplets
shows the current as a function of the applied voltage. Each current and voltage point corresponded to the onset of an additional jet. There was a super-linear relationship between the current and voltage because not only did the number of jets increase with voltage, but the flow rate in each individual jet also increased.
shows the current as a function of the flow rate of the non-conducting liquid. As there was no charge on this liquid, there was no strong correlation. This supports the mechanism of electrospray, as the non-conducting fluid is not extruded by the electric field, but by viscous forces applied by the conducting liquid at the interface between the two fluids.
TABLE 2 Geometric mean diameter and geometric standard deviation of the droplets
3.5. Size Distribution of the Droplets
The real-time size distribution of the droplets is shown in . We were able to control the diameter of the droplets by adjusting the flow rates of the two liquids. When the flow rate of the inner liquid was fixed, the diameter of the droplets increased with the flow rate of the outer liquid. As the total flow rate of the two liquids increased, the diameter of droplets also increased. To characterize the uniformity of the droplets, we used the geometric standard deviation (GSD), defined as

3.6. Droplet Formation
Droplet formation in electrospray occurs when a liquid column is extruded by the electrical stress, and once it exceeds a certain length, the column breaks down into droplets due to instability (CitationCloupeau and Prunetfoch 1994). These droplets then break down again to finer particles due to the excessive charge held on the individual droplets. However, in the case of electrospray with a coaxial nozzle, the mechanism of droplet formation is not well established. Some attempts have been made to describe the droplet formation within the field of micro encapsulation (CitationLoscertales et al. 2002; CitationMei and Chen 2007). However, we hypothesize a new mechanism of a droplet formation. In this mechanism, the inner (conducting) liquid undergoes the same procedure as with a single liquid. When the inner liquid disintegrates to form the finer particles, there are strong Coulombic repulsions between droplets because the individual conducting liquid droplets have the same polarity. The outer liquid column then disintegrates because of the instability of the jets, and the inner liquid droplets emerge from the inner region and spread out due to Coulombic repulsion. The outer liquid then covers the inner liquid droplets due to the viscous interaction between the two liquids. CitationMei and Chen (2007) conducted many experiments with coaxial electrospray, with varying feed conditions. When the inner liquid (ethanol) droplets break through the outer liquid (olive oil), electrospray was not observed. This means that ethanol simply undergoes electrospray by itself, and these results in little interaction between the two liquids. This explanation still requires further development, however, as it doesn't describe the instability in the electrospray technique.
4. Conclusion
We used a grooved coaxial nozzle to generate stable multi-jet operation using a non-conducting fluid. We achieved a large throughput, which was comparable to that achieved by an array of conventional nozzles, and were able to achieve monodisperse droplets. Experimental investigations revealed that stable and reproducible multi-jet operation could be achieved over a wide range of flow rates. The jets consisted of two layers—an inner conducting fluid and the outer non-conducting fluid—and the size of the droplets could be controlled by adjusting the flow rates of the two liquids. We expect that this technique can be extended to numerous non-conducting liquids, and will facilitate practical applications of electrospray such as the production of microfibers by electrospinning without the requirement for a conductivity enhancer. This technique would then allow further development of the synthesis of micro filters and artificial tissue.
Acknowledgments
This work was supported by the BK21 program of the South Korea Ministry of Education, Science, and Technology.
Notes
aValues measured at 20°C.
REFERENCES
- Bocanegra , R. , Galan , D. , Marquez , M. , Loscertales , I. G. and Barrero , A. 2005 . Multiple Electrosprays Emitted from an Array of Holes . J. Aerosol Sci. , 36 : 1387 – 1399 .
- Chen , D. , Pui , D. Y. H. and Kaufman , S. L. 1995 . Electrospraying of Conducting Liquids for Monodisperse Aerosol Generation in the 4nm to 1.8 μm Diameter Range . J. Aerosol Sci. , 26 : 963 – 977 .
- Chen , X. , Jia , L. , Yin , X. , Cheng , J. and Lu , J. 2005 . Spraying Modes in Coaxial Jet Electrospray with Outer Driving Liquid . J. Phys. Fluids. , 17 : 032101
- Cloupeau , M. and Prunetfoch , B. 1994 . Electrohydrodynamic Spraying Functioning Modes: A Critical Review . J. Aerosol Sci. , 25 : 1021 – 1036 .
- Deng , W. , Klemic , J. F. , Li , X. , Reed , M. A. and Gomez , A. 2007 . Liquid Fuel Microcombustor Using Microfabricated Multiplexed Electrospray Sources . Proc. Combust. Inst. , 31 : 2239 – 46 .
- Deng , W. , Waits , C. M. , Morgan , B. and Gomez , A. 2009 . Compact Multiplexing of Monodisperse Electrosprays . J. Aerosol Sci. , 40 : 907 – 918 .
- Duby , M.-H. , Deng , W. , Kim , K. , Gomez , T. and Gomez , A. 2006 . Stabilization of Monodisperse Electrosprays in the Multi-Jet Mode via Electric Field Enhancement . J. Aerosol Sci. , 37 : 306 – 322 .
- Fenn , J. B. , Mann , M. , Meng , C. K. , Wong , S. K. and Whitehouse , C. 1989 . Electrospray Ionization for Mass Spectrometry of Large Biomolecules . Science. , 246 : 64 – 71 .
- Gamero-Castano , M. and Hruby , V. 2001 . Electrospray as a Source of Nanoparticles for Efficient Colloid Thrusters . J. Propul. Power. , 17 : 977 – 987 .
- Kim , W. and Kim , S. S. 2010 . Multi-Shell Encapsulation Using a Triple Coaxial Electrospray System . Anal.Chem. , 82 : 4644 – 4647 .
- Lopez-Herrera , J. M. , Barrero , A. , Lopez , A. , Loscertales , I. G. and Marquez , M. 2003 . Coaxial Jets Generated from Electrified Taylor Cones: Scaling Laws . J. Aerosol Sci. , 34 : 535 – 552 .
- Loscertales , I. G. , Barrero , A. , Guerrero , I. , Cortijo , R. , Marquez , M. and Gañàn-Calvo , A. M. 2002 . Micro/Nano Encapsulation via Electrified Coaxial Liquid Jets . Science. , 295 : 1695 – 1698 .
- Mei , F. and Chen , D. R. 2007 . Investigation of Compound Jet Electrospray: Particle Encapsulation . Phys. Fluids. , 19 : 103303
- Taylor , G. 1964 . Disintegration of Water Drops in an Electric Field . A Math. , 280 : 383 – 397 .
- Xie , J. , Ng , W. J. , Lee , L. Y. and Wang , C. H. 2008 . Encapsulation of Protein Drugs in Biodegradable Microparticles by Co-Axial Electrospray . J. Colloid Interface Sci. , 317 : 469 – 476 .
- Zeleny , J. 1914 . The Electrical Discharge from Liquid Points and a Hydrostatic Method of Measuring the Electric Intensity at Their Surfaces . Phys. Rev. , 3 : 69 – 91 .