Abstract
We present a new in situ aerosol flow reactor for gas-phase studies during drying of aerosol droplets by small-angle X-ray scattering (SAXS). The setup consists of an outer confinement shell and an inner tube for aerosol flow that can be positioned by a remote-controlled motor to perform scanning measurements along the flow path, providing “quasi-time-resolved” information on the drying process. A controlled temperature step is provided by an insulating separation between a cooled and a heated section in the dryer. First experiments were performed on aerosols of surfactant- and silica-precursor/surfactant solutions (CTAB/ethanol, TEOS/CTAB). Measurements of the mesostructure formation during the initial steps of evaporation-induced self-assembly (EISA) were made to illustrate the working principle of aerosol drying during passage through an adjustable temperature gradient.
INTRODUCTION
Structural characterization of aerosols on the nanometer scale is important in a variety of scientific areas: for developing technical applications, like functional powders or catalytic materials (CitationKim et al. 2006; CitationMoon et al. 2009; CitationSanchez et al. 2010), medical applications (drug delivery systems, diagnostic particles, nanotoxicology; CitationTsapis et al. 2002; CitationRavichandran 2009), or for an assessment of the impact of artificial and naturally occurring aerosol particles on environment and climate (CitationWise et al. 2005). Small-angle (and wide-angle) X-ray scattering provides a fast and versatile method for characterizing nanoscale structural features (CitationSchaefer et al. 1991), and even their relation to chemical reactivity and physical properties, if a relevant in situ experiment can be designed (CitationKammler et al. 2005; CitationMarmiroli et al. 2010). Using the high brilliance available at synchrotron sources, it is possible to measure aerosols directly in the gas phase (CitationBoissiere et al. 2003; CitationSen et al. 2007; CitationShyjumon et al. 2008; CitationMitchell et al. 2010), avoiding the introduction of structural alterations by sampling, depositing, fixation, or time passing during the measurement. Time-resolved data acquisition is particularly crucial when fast synthesis processes are to be examined, e.g., in our study, structure formation in aerosols of mesostructured microparticles.
Mesostructured microparticles have applications for catalysis, coatings, optics, and drug delivery, and a large variety of morphologies and properties has been reported by applying different synthetic routes and recipes. Evaporation-induced self-assembly (EISA) by spray drying provides an attractive route for the production of such powders, because it can be run cost-efficiently, fast, and continuously, and provides good control of the product by selecting appropriate process parameters. The EISA process is driven by a progressive concentration of the nonvolatile compounds of a precursor solution during evaporation of a solvent that is usually a mixture of one or more alcohols and water (CitationBrinker et al. 1999). In situ studies of thin film structuring during the EISA process have been very useful for identifying critical parameters such as the relative humidity of the ambient atmosphere (CitationCagnol et al. 2003; CitationGibaud et al. 2003; CitationGrosso et al. 2004; CitationDourdain et al. 2005). Studies available on the mesostructuring during spray drying are, however, mainly restricted to assessing the influence of process parameters on the final product (CitationBruinsma et al. 1997; CitationLu et al. 1999; CitationRao et al. 2002; CitationBore et al. 2003; CitationAreva et al. 2004; CitationHampsey et al. 2005; CitationAlonso et al. 2007; CitationBoissiere et al. 2011), which is a drawback for understanding the mechanisms of the actual self-assembly process. The drying kinetics has previously been identified as influencing the overall particle morphology (CitationJayanthi et al. 1993; CitationTsapis et al. 2002; CitationSen et al. 2007). In the case of mesostructuring droplets, the time- and temperature-dependent shift of alcohol and water content along the radial evaporation gradient of the droplet, additionally, has consequences for mesophase self-assembly (CitationGibaud et al. 2003; CitationAlonso et al. 2007) and Si condensation (CitationBoissiere et al. 2003). The motivation for constructing the in situ aerosol dryer was to characterize the initial stages of the EISA process, by measuring the structural changes during passage of the aerosol through a temperature gradient.
SETUP AND EXPERIMENTAL DETAILS
Aerosol Flow Reactor
shows a schematic drawing of the working principle, and shows a sketch of the aerosol flow reactor. The dryer setup consists of two tubes, an outer confinement shell with fixed position and a vertical slit, and an inner tube with axial slits mounted on a stable aluminum support that can be moved inside the shell by an externally mounted motor stage. The vertical slit also allows wide-angle X-ray measurements up to 2θ ∼ 30°. The slits are not covered with X-ray transparent foils to avoid background scattering from windows contaminated with eventually depositing aerosol. It is important to avoid these deposits, because they undergo continued mesostructuration upon drying during the measurements; therefore, the background signal changes and cannot be used for data correction. This “open construction” demands a reliable exhaust at both the vertical measurement slit and the outlet of the tube to avoid potential contamination of the hutch air with aerosol particles.
FIG. 1 (a) Schematic drawing of the working principle of the flow reactor. a: Cooled part of the dryer, b: insulating separation, c: heated part. The position of d: confinement shell and e: window is fixed, the inner dryer tube with f: axial slit is moved inside by a motor stage to change the measurement position within the aerosol flow path. A PT100 is mounted at the end of the f: slit to control the heating according to the maximum aerosol temperature. g: Ring-shaped outlet for confinement air into the shell. (b) Sketch of the aerosol dryer. a: Cooled part of the dryer, b: insulating separation, c: heated part, d: confinement shell with e: X-ray window, and f: axial slit in the inner tube. Distances are given in mm. (c) Inlet piece with tubes for aerosol, cooling water, and confinement air. (d) Aerosol dryer mounted at the SAXS beamline at ELETTRA.
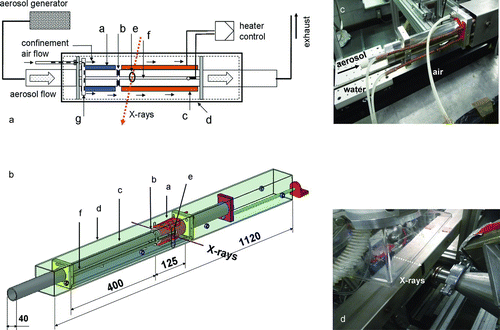
The inlet piece () has an opening for aerosol flow into the inner tube, with a slideable tube inset to allow an optional additional path of maximum ∼200 mm inside the flow reactor before the first measurement position (i.e., at the beginning of the axial slits). Additional openings in the inlet piece allow the flow of air into the outer confinement shell and circulation of cooling water. shows the aerosol dryer mounted on the experimental station at ELETTRA. The inner tube consists of a thermostat-controlled water-cooled (Huber Unistat CC, Offenburg, Germany) part and a heated part that are separated by a thermal insulator to provide a steep and adjustable temperature gradient. Heater tapes (Omegalux, Stamford, USA, 1040 W) were used as heating devices for the presented drying experiments; the effective power density at the inner tube was approximately 2.5 W/cm2. To improve the efficiency and thermal stability, a thermal insulation blanket (ITM Fibermax, Schupp, Aachen, Germany) was attached to the outside of the heater tube. For achieving even higher temperatures, e.g., to observe calcination of aerosols, the heater tapes can be replaced by heating cartridges. A PT100 resistance thermometer was placed at the end of the axial slit (i.e., at the end of measurement range), in the center of the tube, approximately at the same height (with respect to the tube diameter) as the beam position during X-ray measurements (). This configuration was chosen in order to control the heater with respect to the actual aerosol temperature at the final measurement position. shows examples of temperature profiles measured with and without the flow of reference aerosols. The temperature profile at the separation is influenced not only by the solvent but also by the temperature and relative humidity of the gas or air used as confinement. For the presented experiments, the starting temperature of the cooled part was kept at approximately room temperature (20°C–27°C); the maximum applied (air or aerosol) temperature in the scanning range was ∼160°C. The maximum achieved temperature was 300°C (without aerosol). The measurements were taken at steps of 20–40 mm along the dryer tube that corresponded to time steps during aerosol flow of about 200–240 ms. The setup provides a total residence time of the aerosol particles of ∼1500–2000 ms in the scanning range, with a possible temporal resolution of ∼50 ms, depending on the X-ray beam dimensions. These numbers are, however, a lower estimate, because the aerosol flow rate depends not only on the settings of the aerosol generator (for the presented experiments 250–300 l/h); a slight increase of the flow velocity is expected due to the influence of the exhaust system of the experimental hutch and the flow rate of the confinement air. These parameters have to be adjusted to optimize the particle density of the aerosol (determining X-ray signal quality and exposure time) and for confining the flow in the inner tube. For the presented experiments with ethanol-based (EtOH) based precursor solution, the aerosol was generated with a commercial aerosol generator (TSI 3079 Atomizer, Topas GmbH, Dresden, Germany) functioning as a two-stream injection nozzle.
FIG. 2 (a) Temperature steps achieved at the maximum set temperatures of 100°C (squares), 120°C (dots), 150°C (triangles up), and 190°C (triangles down) without aerosol flow. (b) Axial temperature profiles with aerosol of water (squares) and 70% EtOH (dots) and without aerosol flow (triangles) with 150°C as the maximum set temperature at the heated end. The insulation between heated and cooled part is located at 80 mm. The cooled part was kept at approximately room temperature.
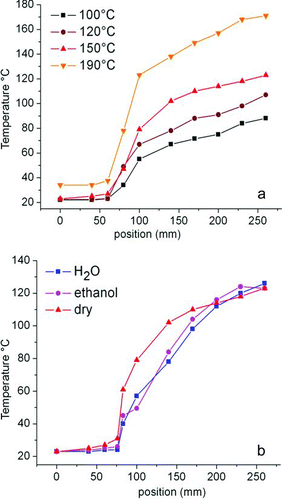
Sample Preparation
Aerosols were produced from an EtOH-rich precursor solution of tetraethylorthosilicate (TEOS)/cetyltrimethylammonium bromide (CTAB)/HCl/H2O/EtOH at molar ratios of 1(TEOS):0.15(CTAB):0.004(HCl):5(H2O):22(EtOH) (CitationLu et al. 1999) and solutions with equivalent molar ratios of CTAB/ EtOH and CTAB/EtOH/H2O. Deionized H2O was used in Millipore quality. TEOS and CTAB were purchased from Sigma Aldrich (Vienna, Austria) and EtOH and HCl from Bartelt (Graz, Austria). The precursor solutions were used after about 6 up to maximum 12 h.
X-Ray Measurements and Data Analysis
In situ gas-phase small-angle X-ray scattering (SAXS) measurements were performed at the Austrian SAXS beamline at ELETTRA, Trieste, Italy, at an energy of 8 keV (CitationAmenitsch et al. 1998). The scattering patterns were recorded with a 2D image plate detector (Mar300, MarResearch, Norderstedt, Germany) using collection times of 200–250 s. The scattering vector (difference between the vector of the incoming and the scattered wave) is related to the scattering angle 2θ via |
| = 4πsin(θ)/λ (λ is the wavelength) and is inversely related to the repeat distance (d spacing) of the sample mesostructure. The gas-phase measurements were restricted to the strongest low-order reflection due to the low scattering intensity of the aerosol, mainly limited by the low particle concentration. Before analysis, the background signal measured at the corresponding positions was subtracted from the patterns, and the resulting images were radially integrated using the software FIT2D (CitationHammersley 1998). The peak parameters were evaluated using the software package IGOR (WaveMetrics, Inc.). Peak position and area were fitted using the function I(q) = A/(1+((q−q
0)/fwhm)2) + a + b q + c q
2, combining a second-order polynomial as residual background with a Lorentz function, where A is the peak height, fwhm is the full width at half maximum, and q
0 is the peak position.
RESULTS AND DISCUSSION
Characterization of the Experimental Setup
Physical parameters during drying have previously been shown to influence the mesostructure formation by their impact on solvent composition and drying speed, influencing Si condensation and degree of order (CitationBoissiere et al. 2003; CitationBore et al. 2003; CitationHampsey et al. 2005). shows the temperature gradients measured at different maximum set temperatures without aerosol flow, with the heater setup used for the presented experiments. The temperature at the dryer entrance was kept at approximately room temperature; the temperature gradient was raised by setting the maximum temperature in the heated part to 100°C, 120°C, 150°C, and 190°C.
The flow velocity and heat capacity of the aerosol have a direct influence on the temperature increase of the aerosol during drying and the radial temperature gradient in the dryer tube (which is changing with axial position and aerosol temperature). To assess the influence of solvent composition on the temperature step, temperature profiles of aerosol of deionized water and 70% EtOH were measured as reference. shows profiles of these aerosols with the maximum dryer temperature set to 150°C, compared with the profile without aerosol flow. The influence of the aerosols and their different heat capacity and evaporation temperature (corresponding to different positions in the flow reactor) is clearly visible immediately after the separation; with progressive drying, it is compensated. This is also valid for aerosols containing nonvolatile solution components (surfactant and Si precursor), the temperature gradients of these systems are also dominated by heater control and solvent evaporation.
X-Ray Experiments
CTAB solutions
shows SAXS measurements of aerosol of CTAB/EtOH solution during spray drying. A clear increase of the peak height was observed in the measurements during initial mesostructure formation and subsequent drying of the structure (). Crystallization of CTAB from a highly concentrated, ethanolic solution is expected to result in a lamellar crystalline mesostructure (CitationCampanelli and Scaramuzza 1986). In bulk solution, EtOH is known to enrich between head and tail groups of the surfactant (CitationDoshi et al. 2003), therefore suppressing curvature induction of the surfactant and disturbing micelle formation (CitationZana 1995). The formation of a lamellar phase parallel to interfaces (interfaces with solid substrate or air) during EISA is also a typical feature of confined systems such as aerosol droplets (CitationFan et al. 2008). The maximum observed at q ∼ 2.1 nm−1 corresponds to a lattice spacing of ∼3 nm that is larger than the length of the stretched CTAB tail group of ∼2.2 nm (CitationVenkataraman and Vasudevan 2001), possibly relating to a strongly tilted surfactant bilayer. The observed slight changes of peak position () could be tentatively explained based on the findings for thin films (CitationDoshi et al. 2003): Initial evaporation of the interlamellar EtOH results in a slight decrease of lattice spacing; subsequent evaporation of EtOH intercalated in the surfactant layer results in a slight increase of the characteristic repeat distance at the final drying stage. The opposite behavior of the H2O-containing solution can be explained by a steric effect of H2O molecules associated with the surfactant head groups during initial faster evaporation of EtOH, before water finally evaporates with a time delay at the final stage.
FIG. 3 (a) SAXS patterns of aerosol of CTAB/EtOH solution during drying at the maximum set temperature of 150°C. The curves are offset for better visibility, the numbers represent the approximate temperature according to Figure 4b. (b) Peak height (full circles/squares) and d spacing (open circles/squares) during drying of CTAB/EtOH and CTAB/EtOH/(H2O) solutions.
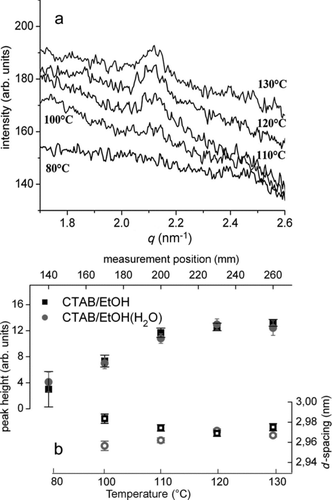
The purpose of these first measurements was to show the feasibility of following the mesophase formation in the gas phase with the presented setup. The results show a potential for also characterizing chemically more complex systems that might show interesting behavior in 3D confinement. It should be pointed out that shows the original curves after background subtraction and a clear signal could be obtained although the scattering contrast (electron density contrast) of CTAB is lower than that of condensing silica.
FIG. 4 Peak position of TEOS/CTAB/HCl/H2O/EtOH during drying at the maximum set temperature of 150°C. A slight decrease of d 10 spacing of the hexagonal phase is visible at the last three positions at the heated end. In contrast to the solutions without TEOS, a peak was observed for this solution already at the first measured position.
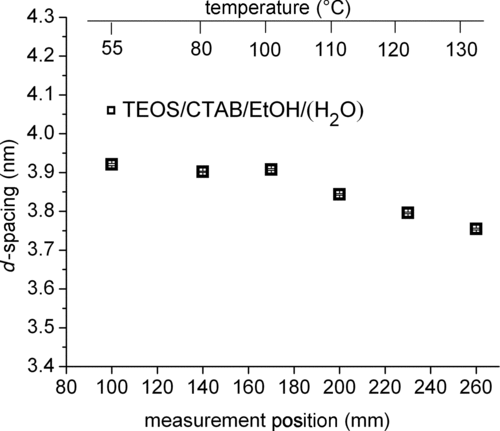
EISA of Si-precursor solution
Solvent composition and content of the precursor solution was previously shown to have an impact on the lattice spacing of the formed mesostructure. EtOH influences the size of micelles in bulk solution or thin films (CitationFontell et al. 1991; CitationDoshi et al. 2003) and inhibits Si condensation (CitationBoissiere et al. 2003). H2O has a geometrical role and aids Si condensation in the precursor solution. In thin films, a structural effect was observed when humidity from the ambient atmosphere was adsorbed to the drying system (CitationCagnol et al. 2003; CitationDoshi et al. 2003). We examined the influence of Si condensation in the precursor solution by measuring aerosols produced from the same batch with intervals of several hours (keeping the remaining solution in a closed vessel on a stirring plate to avoid EtOH evaporation), but we found no significant impact of this factor on the characteristic repeat distance, for the investigated chemical composition and aging times. An influence of decreasing solution EtOH content was, however, clearly visible as an increase of lattice spacing already at the dryer entrance, before the aerosol drying (data not shown). The influence of H2O adsorbed from humidified carrier gas and confinement air was assessed with a previous prototype of the setup. An effect on the repeat distance was visible in the first stages of drying, but was compensated at the end of the drying process. An improvement of the degree of order in the dry powder was observed by applying low (80°C) dryer temperatures (CitationBore et al. 2003; CitationShyjumon et al. submitted).
Measurements of aerosol of TEOS/CTAB/HCl/H2O/EtOH solution showed a signal at q ∼ 1.6 nm−1 corresponding to the (10) reflection of a 2D hexagonal phase, consistent with previous studies reporting such a structure for comparable systems (CitationBoissiere et al. 2003; CitationBore et al. 2003). This system showed a slight decrease of the first-order lattice spacing of about 0.2 nm at the end of the dryer (), at temperatures above 100°C. A decrease of about 0.2 nm was also observed by CitationBoissiere et al. (2003) for measurements after the dryer exit while increasing the heater temperature. During the beginning of the drying process (50°C–100°C), the initial repeat distance was preserved, indicating that EtOH evaporation during spray drying is too fast to allow any significant mesophase transitions in the course, as were observed for thin films (CitationGrosso et al. 2004). The decrease of lattice spacing above 100°C can also be attributed to the evaporation of water from the hydration shell around the CTAB core, causing changes in the core radius (CitationShyjumon et al. submitted).
Powder deposit was collected at the dryer exit during the presented experiments and was measured immediately and 3 days later (see the online supplemental information). The lattice spacing remained the same, indicating that the drying was complete after passage of the dryer tube.
CONCLUSION
We present a new in situ aerosol flow reactor that allows us to follow the structural changes in mesostructured aerosols during solvent evaporation/Si condensation along an adjustable temperature gradient. Controlling the drying process and flow conditions was possible by applying different temperature profiles and by adapting the flow rate of confinement air and exhaust. First experiments were performed on EISA of CTAB/EtOH solutions. It was possible to gain quasi-time-resolved gas-phase measurements of the initial mesophase formation during EISA in aerosol droplets. A clear increase of peak height was observed in the measurements, allowing us to follow the mesostructure formation of CTAB/EtOH solutions and changes during subsequent drying of the structure. In situ measurements of EISA of the Si-precursor solution showed that the lattice spacings did not change considerably in the course of mesostructure formation, but that they were mostly determined by the precursor solution. The lattice spacing also remained stable in the collected powder. Therefore, at least for EtOH-based systems, spray drying seems to offer a route that allows one to control the final unit cell dimensions directly via the solution composition, probably because drying and stabilization happen too fast to allow the additional processes observed for thin films. In the future, this setup will be applied for the direct mesostructural characterization of aerosols, for even more complex processes like self-assembly of hierarchical porous structures, cocrystallization of nanoparticles in mesoporous materials, or the role of 3D confinement on self-assembly and crystallization. We also plan to integrate complementary methods for droplet and particle characterization, such as static light scattering for size measurements.
uast_a_564680_sup_17896109.zip
Download Zip (165.1 KB)Acknowledgments
We thank Christian Morello for his support with the setup. The study was financed by the project SAXIER contract no. RIDS 011934.
[Supplementary materials are available for this article. Go to the publisher's online edition of Aerosol Science and Technology to view the free supplementary files.]
REFERENCES
- Alonso , B. , Véron , E. , Durand , D. , Massiot , D. and Clinard , C. 2007 . New Insights into the Formation of Textures through Spray-Drying and Self-Assembly . Microporous Mesoporous Mater. , 106 : 76 – 94 .
- Amenitsch , H. , Rappolt , M. , Kriechbaum , M. , Mio , H. , Laggner , P. and Bernstorff , S. 1998 . First Performance Assessment of the Small-Angle X-Ray Scattering Beamline at ELETTRA . J. Synchrotron Radiat. , 5 : 506 – 508 .
- Areva , S. , Boissiere , C. , Grosso , D. , Asakawa , T. , Sanchez , C. and Linden , M. 2004 . One-Pot Aerosol Synthesis of Ordered Hierarchical Mesoporous Core-Shell Silica Nanoparticles . Chem. Comm. , 10 : 1630 – 1631 .
- Boissiere , C. , Grosso , D. , Amenitsch , H. , Gibaud , A. , Coupé , A. , Baccile , N. and Sanchez , C. 2003 . First In-Situ SAXS Studies of the Mesostructuration of Spherical Silica and Titania Particles during Spray-Drying Process . Chem. Comm. , 9 : 2798 – 2799 .
- Boissiere , C. , Grosso , D. , Chaumonnot , A. , Nicole , L. and Sanchez , C. 2011 . Aerosol Route to Functional Nanostructured Inorganic and Hybrid Porous Materials . Adv. Mater. , 23 : 599 – 623 .
- Bore , M. T. , Rathod , S. B. , Ward , T. L. and Datye , A. K. 2003 . Hexagonal Mesostructure in Powders Produced by Evaporation-Induced Self-Assembly of Aerosols from Aqueous Tetraethoxysilane Solutions . Langmuir , 19 : 256 – 264 .
- Brinker , C. J. , Lu , Y. , Sellinger , A. and Fan , H. 1999 . Evaporation-Induced Self-Assembly: Nanostructures Made Easy . Adv. Mater. , 11 : 579 – 585 .
- Bruinsma , P. J. , Kim , A. Y. , Liu , J. and Baskaran , S. 1997 . Mesoporous Silica Synthesized by Solvent Evaporation: Spun Fibers and Spray-Dried Hollow Spheres . Chem. Mater. , 9 : 2507 – 2512 .
- Cagnol , F. , Grosso , D. , Soler-Illia , G. J. D. A. , Crepaldi , E. L. , Babonneau , F. , Amenitsch , H. and Sanchez , C. 2003 . Humidity-Controlled Mesostructuration in CTAB-Templated Silica Thin Film Processing. The Existence of a Modulable Steady State . J. Mater. Chem. , 13 : 61 – 66 .
- Campanelli , A. R. and Scaramuzza , L. 1986 . Hexadecyltrimethylammonium Bromide . Acta Cryst. C , 42 : 1380 – 1383 .
- Doshi , D. A. , Gibaud , A. , Liu , N. , Sturmayr , D. , Malanoski , A. P. , Dunphy , D. R. , Chen , H. , Narayanan , S. , MacPhee , A. , Wang , J. , Reed , S. T. , Hurd , A. J. , Van Swol , F. and Brinker , C. J. 2003 . In-Situ X-Ray Scattering Study of Continuous Silica—Surfactant Self-Assembly during Steady-State Dip Coating . J. Phys. Chem. B , 107 : 7683 – 7688 .
- Dourdain , S. , Rezaire , A. , Mehdi , A. , Ocko , B. M. and Gibaud , A. 2005 . Real Time GISAXS Study of Micelle Hydration in CTAB Templated Silica Thin Films . Physica B: Condensed Matter , 357 : 180 – 184 .
- Fan , J. , Boettcher , S. W. , Tsung , C.-K. , Shi , Q. , Schierhorn , M. and Stucky , G. D. 2008 . Field-Directed and Confined Molecular Assembly of Mesostructured Materials: Basic Principles and New Opportunities . Chem. Mater , 20 : 909 – 921 .
- Fontell , K. , Khan , A. , Lindström , B. , Maciejewska , D. and Puang-Ngern , S. 1991 . Phase Equilibria and Structures in Ternary Systems of a Cationic Surfactant (C16TABr or (C16TA)2SO4), Alcohol, and Water . Colloid Polym Sci. , 269 : 727 – 742 .
- Gibaud , A. , Grosso , D. , Smarsly , B. , Baptiste , A. , Bardeau , J. F. , Babonneau , F. , Doshi , D. A. , Chen , Z. , Brinker , J. C. and Sanchez , C. 2003 . Evaporation-Controlled Self-Assembly of Silica Surfactant Mesophases . J. Phys. Chem. B , 107 : 6114 – 6118 .
- Grosso , D. , Cagnol , F. , Soler-Illia , G. J. D. A. , Crepaldi , E. L. , Amenitsch , H. , Brunet-Bruneau , A. , Bourgeois , A. and Sanchez , C. 2004 . Fundamentals of Mesostructuring through Evaporation-Induced Self-Assembly . Adv. Func. Mater. , 14 : 309 – 322 .
- Hammersley , A. P. 1998 . FIT2D V9.129 Reference Manual, V3.1, ESRF Internal Report ESRF98HA01T ESRF, Grenoble. Available online at: http://www.esrf.eu/computing/scientific/FIT2D
- Hampsey , E. J. , Arsenault , S. , Hu , Q. and Lu , Y. 2005 . One-Step Synthesis of Mesoporous Metal-SiO2 Particles by an Aerosol-Assisted Self-Assembly Process . Chem. Mater. , 17 : 2475 – 2480 .
- Jayanthi , G. V. , Zhang , S. C. and Messing , G. L. 1993 . Modeling of Solid Particle Formation during Solution Aerosol Thermolysis: The Evaporation Stage . Aerosol Sci. Technol. , 19 : 478 – 490 .
- Kammler , H. K. , Beaucage , G. , Kohls , D. J. , Agashe , N. and Ilavsky , J. 2005 . Monitoring Simultaneously the Growth of Nanoparticles and Aggregates by In Situ Ultra-Small-Angle X-Ray Scattering . J. Appl. Phys. , 97 : 054309
- Kim , H.-S. , Lee , K.-H. and Kim , S.-G. 2006 . Growth of Monodisperse Silver Nanoparticles in Polymer Matrix by Spray Pyrolysis . Aerosol Sci. Technol. , 40 : 536 – 544 .
- Lu , Y. , Fan , H. , Stump , A. , Ward , T. L. , Rieker , T. and Brinker , C. J. 1999 . Aerosol-Assisted Self-Assembly of Mesostructured Spherical Nanoparticles . Nature , 398 : 223 – 226 .
- Marmiroli , B. , Grenci , G. , Cacho-Nerin , F. , Sartori , B. , Laggner , P. , Businaro , L. and Amenitsch , H. 2010 . Experimental Set-Up for Time Resolved Small Angle X-Ray Scattering Studies of Nanoparticles Formation Using a Free-Jet Micromixer . Nucl. Instrum. Methods Phys. Res. B , 268 : 329 – 333 .
- Mitchell , J. B. A. , di Stasio , S. , LeGarrec , J. L. and Florescu-Mitchell , A. I. 2010 . Synchrotron Radiation Studies of Additives in Combustion. I: Water . Nucl. Instrum. Methods Phys. Res. B , 268 : 1486 – 1491 .
- Moon , Y. K. , Lee , J. , Lee , J. K. , Kim , T. K. and Kim , S. H. 2009 . Synthesis of Length-Controlled Aerosol Carbon Nanotubes and Their Dispersion Stability in Aqueous Solution . Langmuir , 25 : 1739 – 1743 .
- Rao , R. G. V. , Lopez , G. P. , Bravo , J. , Pham , H. , Datye , A. K. , Xu , H. F. and Ward , T. L. 2002 . Monodisperse Mesoporous Silica Microspheres Formed by Evaporation-Induced Self-Assembly of Surfactant Templates in Aerosols . Adv. Mater. , 14 : 1301 – 1304 .
- Ravichandran , R. 2009 . Nanotechnology-Based Drug Delivery Systems . Nanobiotechnol , 5 : 17 – 33 .
- Sanchez , C. , Rozes , L. , Ribot , F. , Laberty-Robert , C. , Grosso , D. , Sassoye , C. , Boissiere , C. and Nicole , L. 2010 . “Chimie Douce”: A Land of Opportunities for the Designed Construction of Functional Inorganic and Hybrid Organic–Inorganic Nanomaterials . C. R. Chimie , 13 : 3 – 39 .
- Schaefer , D. W. , Olivier , B. J. , Hurd , A. J. , Beaucage , G. B. , Ivie , J. J. and Herd , C. R. 1991 . Structure of Combustion Aerosols . J. Aerosol Sci. , 22 : 447 – 450 .
- Sen , D. , Spalla , O. , Taché , O. , Haltebourg , P. and Thill , A. 2007 . Slow Drying of a Spray of Nanoparticles Dispersion: In Situ SAXS Investigation . Langmuir , 23 : 4296 – 4302 .
- Shyjumon , I. , Rappolt , M. , Sartori , B. , Amenitsch , H. and Laggner , P. 2008 . Novel In Situ Setup to Study the Formation of Nanoparticles in the Gas Phase by Small Angle X-Ray Scattering . Rev. Sci. Instrum. , 79 : 043905
- Shyjumon , I. , Rappolt , M. , Sartori , B. , Cacho-Nerin , F. , Grenci , G. , Amenitsch , H. and Laggner , P. Mesostructured Si-Particles Studied by Comparison of Gas Phase and Powder Deposit X-Ray Diffraction . Submitted
- Tsapis , N. , Bennett , D. , Jackson , B. , Weitz , D. A. and Edwards , D. A. 2002 . Trojan Particles: Large Porous Carriers of Nanoparticles for Drug Delivery . Proc. Nat. Acad. Sci. U. S. A. , 99 : 12001 – 12005 .
- Venkataraman , N. V. and Vasudevan , S. 2001 . Hydrocarbon Chain Conformation in an Intercalated Surfactant Monolayer and Bilayer . Proc. Indian Acad. Sci. (Chem. Sci.) , 113 : 539 – 558 .
- Wise , M. E. , Biskos , G. , Martin , S. T. , Russell , L. M. and Buseck , P. R. 2005 . Phase Transitions of Single Salt Particles Studied Using a Transmission Electron Microscope with an Environmental Cell . Aerosol Sci. Technol. , 39 : 849 – 856 .
- Zana , R. 1995 . Aqueous Surfactant–Alcohol Systems: A Review . Adv. Coll. Interf. Sci. , 57 : l – 64 .