Abstract
Inorganic and organic constituents of aerosols from a major railyard and repair facility were characterized to develop a profile of emissions from railyard activities. The railyard has very consistent downslope winds blowing laterally across the railyard for about 8 hours each night, so two sampling stations were used, one just upwind of the railyard and one downwind adjacent to the railyard fence line. Aerosol samples were collected by rotating drum impactors (DRUM and Lundgren) in up to 9 size modes for 5 weeks in summer and fall of 2005 in tandem with the Roseville Railyard Aerosol Monitoring Project (RRAMP), which measured, black carbon (BC) PM2, as well as NO and NO2. The DRUM aerosol samples were analyzed for mass, optical absorption, and elemental content in 3 h time resolution to allow separation of day and night. Organic analysis was conducted on another set of time integrated size-segregated samples taken by a Lundgren impactor during nighttime hours. The ratio between the downwind versus upwind sites at night was as high as 21.9 (NO, RRAMP) and 6.4 (optical absorption, DRUM) but many species had ratios greater than 2, demonstrating which aerosols arose from railyard activities. The main emissions from the railyard consisted of very fine (0.26 > D p > 0.09 μm) and ultrafine (<0.1 μm) aerosols associated with diesel exhaust such as mass, organic matter, transition metals, and sulfur, the latter 3.3% of the mass since locomotive diesel fuel still contained sulfur. There were also coarse soil aerosols contaminated with anthropogenic metals and petroleum-derived n-alkanes. The aerosol PAH (polycyclic aromatic hydrocarbons) profile showed higher proportions of the heavier PAHs, such as benzo[a]pyrene, compared to diesel truck exhaust on a per unit mass. These aerosols were mostly in the ultrafine (<0.1 μm) size mode, enhancing lung capture. These results and those of Roseville Railyard Aerosol Monitoring Project (RRAMP) largely confirm earlier California Air Resources Board's (ARB) model estimates of health impacts downwind of the railyard based on diesel exhaust, while adding data on very fine transition metals and contaminated soils, potentially important to human health.
INTRODUCTION
The aerosol emissions from railyards are largely understudied despite a high density of large diesel engines and associated industrial activities often in close proximity to dwellings. Locally, railroads dominate the pollutant inventory for NOx (24.2%) and SO2 (63.3%) for Placer County, including emissions from the Union Pacific Rail Road's (UPRR) J. R. Davis Railyard in Roseville (), CA (ARB Citation2010, Supporting Materials 1). The 0.5 km wide by 6 km long railyard just north of Sacramento is the largest maintenance and service yard in the western United States, with over 31,000 locomotives visiting each year for repair and maintenance, with an additional 16,000 trains transiting through the yard each year (2005).
The California Air Resources Board (ARB) initiated a risk assessment study in 2000 of diesel emissions from this site. The results of this assessment study (Hand et al. Citation2004) concluded excess cancer risk levels of 500 per million in the neighborhood immediately downwind of the railyard, and the 100 per million extended well into Roseville. Through a joint agreement between UPRR and the California ARB, Placer and Sacramento Counties APCDs, and the US EPA, Region IX, a multi-year plan is in place for reduction of pollutants and measuring the improvement through the Roseville Railyard Aerosol Monitoring Project (RRAMP) (Campbell and Fujita Citation2009).
The Roseville Railyard is a good site to collect emission profiles from diesel locomotive engines for a variety of reasons. The first reason is that the summertime meteorology at the site is both stable and predictable, hence samplers can be sited both upwind and downwind of the railyard to collect paired aerosol data. The second advantage of sampling at the Roseville Railyard is that it is a service depot that conducts engine repairs and maintenance. This site does not have truck traffic that is ubiquitous at other rail depots that are primarily associated with transport of goods. Lastly, the emission profiles collected will represent a fleet of locomotives rather than a single locomotive that would be typical of an emission sample directly from the stack. The main limitation of sampling at this site is that repair activities (e.g. welding, grinding, etc.) could generate aerosols that are not emissions from locomotives.
The objective of this study was to characterize the aerosol emission from the Roseville Railyard. Size-resolved aerosol samples for elemental analysis were collected upwind and downwind of the railyard. Size-resolved aerosols were also collected downwind of the railyard for organic analysis. The inorganic and organic aerosol data were combined with the gas data (e.g., NO, NO2, etc.) collected by local agencies to compile a profile of emissions from the railyard.
METHODS AND MEASUREMENTS
RRAMP and Placer County APCD Data
Since the local wind patterns move predictably across the railyard during summertime nights, the RRAMP measurements were conducted during the summers from 2005 to 2008 (Campbell and Fujita Citation2009; Christofk Citation2009) in order to track promised reductions in railyard aerosols. RRAMP measured NO, NO2, and black carbon (BC) hourly on a southeast to northwest transect across the railyard parallel to the regular nighttime downslope winds. The () PM2.5 mass was measured at the same sites but over 24 h. Because of the time averaging, it mixed railyard and nonrailyard aerosols. The downwind Denio site was placed close to the location of the maximum excess cancer risk prediction of the 2004 ARB report (Hand et al. Citation2004). The measurement selected by RRAMP to identify railyard impacts and track reductions in railyard emissions was Elemental Carbon (EC) mass, derived from the BC measurement by Aethalometer, as a surrogate for diesel particulate matter (DPM).
The HETF/UC Davis DELTA Group Data
The HETF/DELTA Group (for information on these organizations see the Acknowledgements) measurements were designed to gain additional information on railyard emissions, including aerosols as a function of size, time, and composition, and time integrated speciated organic aerosols. Samples were collected by a pair of DELTA Group 8 stage rotating drum (DRUM) impactors in 8 size modes, 10–0.09 μm, (Cahill et al. Citation1985; Raabe et al. Citation1988), located at the Denio and Pool sites. Aerosols were impacted onto lightly greased Mylar strips, (Wesolowski et al. Citation1978; Cahill Citation1979). The 2 DRUM samplers were collocated for 4 days at the Denio site to establish equivalency, but only the very fine (0.26 > D p > 0.09 μm) stage of the Pool sampler met the ±15% equivalency criteria, due to an internal leak later discovered in one DRUM stage. Organics were collected during the July–August period with an identical DELTA 8 DRUM for 8 size modes using fired aluminum strips as the deposition foils, with a Teflon ultrafine after-filter. For the two fall periods, a high flow Lundgren 4 stage impactor plus after-filter (Lundgren Citation1967) was used, keyed to the night winds from 10 PM to 6 AM. Organic data were averaged over the entire time periods. The sampling enhancements are shown below in , with the average value of RRAMP parameters at the same site and time.
The DRUM samples were analyzed for mass by soft beta ray transmission, shown to be equivalent to Federal Reference Method (FRM) mass during a 1 year side by side study with the ARB at downtown Sacramento, 2007, (Supplemental Information 2) while elements were analyzed by synchrotron-induced x-ray fluorescence (S-XRF) on the University of California Davis microprobe beam line 10.3.1 at the Advanced Light Source, Lawrence Berkeley NL. The DRUM samples were also analyzed every 3 h for optical absorption by optical spectroscopy. (Cahill and Wakabayashi Citation1993; Bench et al. Citation2002; Miller Citation2002). All aspects of sampling and analysis are validated in DRUM Quality Assurance Protocols ver 1/09 (summarized in the Supplemental Information-2 and intact on the DELTA web site, http://delta.ucdavis.edu).
Organic samples were analyzed for selected organics, namely alkanes, PAHs, acids, and sugars, roughly 80 species in all, for each size range using GC-MS. More details on the organic methodology are presented in (Cahill Citation2010) and Supplemental Information-4.
TABLE 1 Sampling schedule and mean RRAMP monitoring data for the four time periods utilized
RESULTS
Meteorology and Trajectory Analysis
The Sacramento meteorological parameters of the study period, 8–12 July (colocated DRUMs at Denio) and from 12 July–17 August, Pool-Denio upwind downwind DRUMs, met the RRAMP design parameters very well. The mean nighttime (10 PM–6 AM) wind direction was 160°P, mean daytime wind direction 270°, WSW, SW, or SSW, with 7 exceptions (10, 16, 24, 26 July; 7, 13, 14 August) when the wind was from the south. The wind velocities were steady, and daytime maximum temperatures ranged from 26°C to 40°C (Campbell and Fujita Citation2009). A summary of the meteorological data is in the Supplemental Information 3. There were periods of stronger wind velocities and oceanic influences seen in the form of sea salt (July 22, July 25 and 26, August 4 and 13) which can overcome the nighttime cross-railyard profile (Supplemental Information 3).
RRAMP Results
The RRAMP results for summer, 2005, confirmed the soundness of the project design in that it clearly separated railyard diesel emissions from upwind sources such as nearby Interstate 80. (Campbell and Fujita Citation2009) ( a and b). Since the Pool site is downwind of the railyard for roughly 16 h each day, the extreme ratio of NO, for example, Denio/Pool, requires some discussion. The low Pool daytime concentrations are caused both by the better ventilation of the stronger daytime winds and the fact that the typical daytime winds from the southwest cross a less active portion of the railyard.
During RRAMP, EC was measured using Aethalometer (BC) data and supplemented by EC from 24 h filters by Sunset Labs. The Aethalometer produces real-time data with high time resolution, but the measurements drift over time as observed by comparing units placed in close proximity to each other (Campbell and Fujita Citation2009). Both the Aethalometer and filter based techniques have the further limitation that there is no separation of the particles by size making it difficult to identify the processes emitting the carbon.
Calculating the downwind–upwind ratios for the July–August RRAMP study period, it is clear that nitric oxide (NO) has the best discrimination between railyard and nonrailyard sources in summer, (×22.1) () with NO2 close behind (×7.2). The BC (×2.3) is significantly worse, while 24 h average PM2.5 (×1.5) is not very specific to the railyard, since the 24 h samples integrate both upwind and downwind times.
HETF/UC Davis DELTA Group Results
Identification of addition railyard emissions above and beyond RRAMP's limited measurements can be obtained by two methods: (1) direct upwind–downwind comparisons, and (2) utilization of the extreme day–night variations at the Denio site, allowed by the ability of the DRUM sampler to gather data on aerosols versus size, time, and composition.
The aerosols collected in the Denio 8 DRUM for July and August are shown below in .
FIG. 3 Photograph of DRUM strips, Stages 1 (top) to Stage 8 (bottom) from the Denio site, 8 July–17 August 2005. There is fiducial marker at the end and slight tear in the end of Stage 8. The black lines are soot each evening.
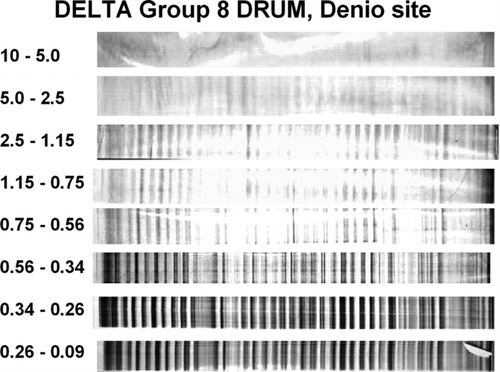
The dramatic day–night differences seen on the DRUM strips echo the results of RRAMP for NO and NO2, but are more regular than the RRAMP BC data (). Note that for the BC, the deposit in the 0.34–0.26 μm size mode is slightly darker than the 0.26–0.09 μm mode, but the pattern is seen for all size modes up to 5.0 μm, above which the width of the DRUM slot averages over day and night. This pattern is largely absent from the Pool site. Thus, with the support of the meteorological analysis, the RRAMP data gas and particulate data, and the qualitative but compelling patterns DRUM samples of , we can evaluate emission from the railyard via the upwind–downwind differences and ratios using nighttime hours, circa 10 PM–6 AM.
Railyard Emissions Via Upwind–Downwind Ratios at Night
In order to quantify the average impact of the railyard, the upwind–downwind ratios at night allow a clean separation of the influence of upwind sources. The failure of the coarser DRUM stages at the Pool site to meet quality assurance (QA) criteria in a side by side pretest only allowed comparisons of the very fine (0.26 > D p > 0.09 μm) size mode. However, most diesel exhaust occurs in size ranges below 0.26 μm (Abu-Allaban et al. Citation2002; Gertler et al. Citation2003; Abu-Allaban et al. Citation2004) with about half in this size mode (Zielinska et al. 2004; Riddle et al. Citation2007).
In order to analyze the very fine mode DRUM samples in 3 h increments, the 3 major analytical techniques used in our study; mass (by soft beta ray transmission), optical analysis versus wavelength (by optical spectroscopy), and elemental concentrations (by synchrotron-induced x-ray fluorescence, S-XRF). These are described in Supplemental Information 2.
Analysis of Mass
Mass values were processed with a time resolution of 1½h. Mass can be measured by transmission of beta rays through foils and filters (Chueinta and Hopke Citation2001). We have developed a soft beta ray (Ni63) source matched in energy to the thin Mylar (480 μg/cm2) deposition foils and stretched Teflon filters and capable of analysis in 0.5 mm steps. Precision of the beta gauge for very fine particles at the Denio site are included in the Supporting Materials, as are the results of a recent intercomparison, DRUM to FRM, with the California Air Resources Board in Sacramento, 2007. In , we show mass measurements at the Pool site (upwind at night) and the Denio site (downwind at night) for the very fine (0.26 > D p > 0.09 μm) aerosols for the July–August campaign.
FIG. 4 Mass Comparison, Pool versus Denio, for very fine (0.26 > D p > 0.09 μm) mass. Colocated mass comparisons occur for 12 July (in boxed area).
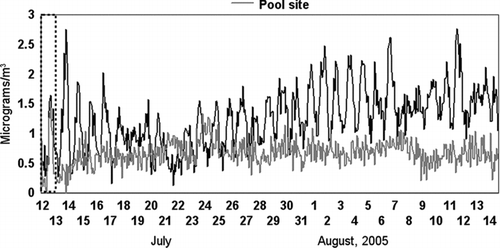
Except for the periods of strong marine influence starting on 22 July, a strong upwind–downwind difference is seen each night. The extreme day–night pattern seen in the RRAMP data, especially the NO results, is mirrored in the mass data that showed the same sort of pattern. The average downwind minus upwind value at night was about 1.5 μg/m3, but recall that these data omit both the important ultrafine component and all mass above 0.26 μm. The RRAMP mean PM2.5 24 h mass difference for this period was 4.6 μg/m3.
Optical Analysis
The Mylar strips from the DRUM samplers offer a new approach for quantifying the light absorption pattern seen in . Greased Mylar is between 65% and 80% transmission for light from 350 nm to >800 nm (Miller Citation2002). Below 310 nm Mylar is effectively 100% absorptive. Mylar can therefore be used effectively as a substrate from transmission spectroscopy in the ultraviolet A through the near infrared regions of the electromagnetic spectrum. Later, we show the optical attenuation for the summer intensive.
It can be seen that there is good agreement between the measured absorption coefficient at the Denio and Pool strips when the DRUMs were colocated (). The impacts of the marine incursions from 22 July to 27 July and 12 August are also clearly in evidence.
Elemental Analysis
Aerosols of special interest are the very fine phosphorus, zinc and sulfur, all tracers of truck diesel emissions (Zielinska et al. 2004; Brandenberger et al. Citation2005). Elemental analyses were performed on all DRUM strips with 3 h resolution. The results for sulfur from the Pool site and the Denio site are shown in , but other elements also showed this pattern. Note that the first 4 days, the 2 DRUMs were located side by side at the Denio site to validate equivalency.
FIG. 6 Sulfur in the very fine (0.26 < D p < 0.09 μm), mode at the Pool site and the Denio site. 9–12 July the samplers were colocated at the Denio site (in the dashed area).
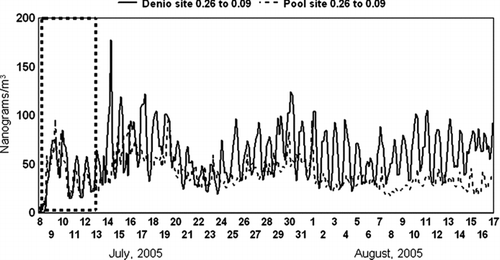
These results and those from the rest of the S-XRF analyses for the very fine (0.26 > D p > 0.09 μm) size mode are summarized in , along with RRAMP results for the same period, 12 July–15 August 2005.
TABLE 2 Summary of nighttime enhancements of very fine aerosols, Pool (upwind) versus Denio (downwind)
shows that the very fine optical absorption, dominated by BC, was highly enhanced downwind of the railyard, by a ratio of 6.3, while a number of species known to occur in diesel engines were typically enhanced by about a factor of 2. However, we note that the zinc to phosphorus ratio is an order of magnitude lower than that seen in diesel trucks (Zielinska et al. Citation2003).
Time Dependent Analyses of Aerosols
Information can also be gained by examining in detail the time dependency of the aerosol data as a function of size and composition. The 3 contributions to the attenuation coefficient are reflection, scattering, and absorption. For the particles in the smallest 3 size modes, this is the most likely dominated by absorption. This is supported by up to a twenty fold increase in the specific absorption (absorption per unit mass) from the larger size modes to the smaller size modes. This is also consistent with the visual observation of very black deposits on the lower stages, even though there is little mass, and the expectation that the combusted carbon will appear in the smaller particles near its source. This allows time dependent analysis of soot versus RRAMP NO data.
The striking day–night pattern seen in NO and other parameters is also evident in these data. The comparison of NO to 530 nm () absorption echo the results shown by RRAMP in a, and show that the NO and BC sources are not always correlated. One possible reason for this may be impact of idling diesel engines versus those under full power, but we do not have enough information to identify the causes.
FIG. 7 Nitric oxide and attenuation coefficient at 530 nm in the 0.26 > D p > 0.09 μm size mode measured at the Denio site.
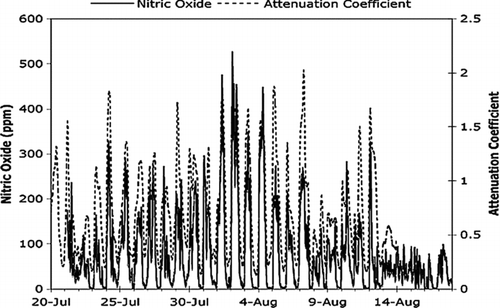
The sulfur of , represents about 3.3% of the mass in this size mode. Typically anthropogenic metals in the very fine mode such as zinc and copper showed occasional spikes of short duration and modest concentrations (a few ng/m3) at the Denio site absent from the Pool site. One persistent difference was seen in calcium, which in this size mode is not derived from soil (). It is, however, commonly used as an antacid in lubricating oil for sulfur-containing fuels and observed in this size mode in diesel tests (Zielinska et al. Citation2003; Zielinska et al. 2004).
FIG. 8 Denio versus Pool comparison for very fine (0.26 > D p > 0.09 μm) calcium. Note the good agreement for the colocated samplers, 8–12 July, and the interference with the pattern during the period of marine incursions, 22–24 July.
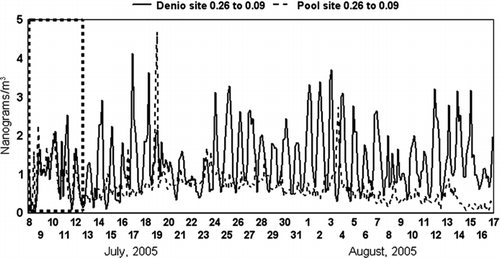
While the very fine aerosols are normally about ½ the total mass of DPM (Zielinska et al. Citation2003), the diesel aerosols downwind of the Roseville Railyard are coarser, extending well into the accumulation mode, as seen in and in the nighttime railyard sulfur size distribution extended in size up to 2.5 μm ().
Significant correlated sulfur mass extended in size up to 2.5 μm. This pattern supports ARB 2004's estimate of the importance of the idling diesel engines in railyard emissions.
The data on sulfur can also be compared to crustal elementals seen at the Denio site ().
Looking in detail at the mass to elemental profiles in time, it is clear from the data (, below) that the coarser soils dominated Roseville's air during the day when wind speeds were high whereas the fine, very fine, and ultrafine particles dominated at night.
Size Distributions of Elements
The nighttime size distributions at the Denio site () show both the presence of soils that are resuspended on the slow nighttime winds, with considerable admixtures of elements that far exceed the expected earth crustal ratios (Weast Citation1989) and thus must be considered anthropogenic. Note that the silicon/iron ratio approaches 1:1 for submicron particles, when Enrichment Factor (EF) from earth crustal ratios would be about 5:1. This clearly indicates excess iron in the soil with a likely anthropogenic source. Many transition metals had high EFs such as 33:1 (zinc) and 43:1 (copper). The presence of fine potassium is unexplained since the normal submicron source, residential wood burning, is unlikely in temperatures that reached 40°C during the study. The most probable cause is burning of agricultural waste and/or small forest fires in the Sierra Nevada.
Based on the size distribution of the potentially toxic very fine copper, we can anticipate even high levels in the unmeasured ultrafine (<0.09 μm) mode. Veryfine and ultrafine copper aerosols are emitted from brake pads in trucks and cars.
Analysis of Organic Components
The analysis of organic components as a function of particulate size required different sample collect techniques than for mass, optics, and elements. Samples were integrated over time, but used nighttime hours identified by the RRAMP and DRUM data. The particulate samples collected were analyzed for four classes of chemicals, namely PAHs (16), alkanes (18), organic acids (22), and sugars (11). Details of the analytical methods and the full data set are in the Supporting Materials 4, and in references (Cahill Citation2010). A major component of the quality assurance is the recovery of isotopically-labeled internal standards applied directly on the aerosol samples prior to sample extraction. The recoveries of the isotopically-labeled internal standard were calculated relative to an injection standard (pyrene-d 10) added to the sample extract just prior to instrumental analysis. These recoveries averaged 77% and are shown in detail in the supplemental information (Supplemental Information 4).
Based on the recovery data of the labeled surrogates from all the sample matrices (aluminum foil and quartz filters), it appears that the analytical method was effective for the heavier PAHs, n-alkanes, and some of the organic acids. Since the data are keyed to the isotopically labeled standards, there is no need to scale the results using the above recovery data. The good recovery numbers merely confirm the analytical procedures are efficient. The minimum detection limit (MDL) for this study was defined as the mean field blank signal plus 3 standard deviations (SD) of the field blanks (mean + 3 SD). Therefore, the data reported herein is well above the background contamination of the field blanks.
The relative fraction of the different identified organic constituents shows that the identified alkanes represent the greatest proportion of the identified mass, and using the consumer price index (CPI), most of these are petroleum derived. Sugars represented 18–25% of the identified mass and organic acids were 21–31% of the mass. The PAHs, which are considered one of the more toxic components of the aerosols, were 1.1–2.0% of the identified organic matter. These chemical profiles, supported by size distribution data, show that a considerable fraction of the organic matter probably originated from sites other than the Roseville Railyard. In particular, the sugars and the long-chain alkanoic acids are likely biological and/or cooking in origin.
Examining the PAHs in more detail due to their importance as a toxic aerosols, the sampling was done using 2 distinct instruments, both slotted impactors were: (1) the DELTA 8 DRUM at 16 L/min, and (2) The classic Lundgren 4 DRUM at 150 L/min. Both had after-filters. The 8 DRUM integrated over 7 weeks, 5 August–27 September, 24 h/day, and the two Lundgren runs were 10 days each, 27 September–7 October, 7 October–17 October, and nighttime only. The results are shown in .
TABLE 3 Concentrations (pg/m3) of particulate PAHs observed at the Roseville Railyard. The scaled 8 DRUM data, ×2.6, represents the assumption that there was negligible PAH contamination during daylight hours, based on ambient PAH sampling at a background site, June 2005 (Cahill et al. Citation2007)
The Lundgren PAH concentrations were approximately 4 times higher than the 8-stage DRUM. We propose that this appears to be the result of the 8-stage DRUM samples being “diluted” by the daytime sampling that lacked any railyard influence ( a, x 2). Note that the median benzo[a]pyrene results seen by the ARB at the nearby Roseville sampling site next to I-80 was only 25 pg/m3 for the years 2002–2004, the latest data available (ARB Citation2010).
One advantage of the 8 DRUM over the Lundgren was additional size information. shows two 3 PAHs, one light, and two heavy versus particles size for summer, 2005. The presence of PAHs in the ultrafine size mode shows a finer aerosol size that may have health consequences (Kilburn et al. Citation2000; Parent et al. Citation2007) as indicated by data on PAHs from diesel trucks (Zielinska et al. 2004).
FIG. 13 Pyrene, benzo[a]pyrene, and Indo[1,2,3-cd]pyrene concentrations (pg/m3) in the different size fractions of the 8-stage DRUM sampler and the after-filter.
![FIG. 13 Pyrene, benzo[a]pyrene, and Indo[1,2,3-cd]pyrene concentrations (pg/m3) in the different size fractions of the 8-stage DRUM sampler and the after-filter.](/cms/asset/58a09fd8-8656-4b92-bef4-285eadf985e4/uast_a_580796_o_f0013g.gif)
The PAH results from the Lundgren sampler also support these observations. This sampler was only operated during the nighttime hours when the local meteorology brings a weak down-slope breeze across the railyard towards the sampling site. Therefore, the higher concentrations observed by this sampler were expected and support the assertion that they are coming from the site.
The other class of organic chemicals of importance to railyard emissions was the alkanes and related hydrocarbons. Unlike the PAHs, there were appreciable alkanes in the field blanks but the concentrations were generally low enough not to interfere with the quantification of the aerosol alkanes. Both the 8-stage DRUM and the Lundgren samples showed that the C29 n-alkane was the most abundant chemical observed with concentrations between 9 and 30 ng/m3. Unlike the PAH data, the concentrations of alkanes were different between the early and the late Lundgren samples with the early sample having concentrations that were almost twice as high as the late sample. The 8-stage and early Lundgren samples had no clear pattern of odd–even carbon number species, suggesting a petroleum source of the hydrocarbons.
DISCUSSION
The RRAMP data (Campbell and Jujita 2009) provide measurements of DPM, roughly 7 μg/m3 DPM from the Denio-Pool comparison roughly confirms the ARB's 2004 estimate of the impact of the railyard on Roseville, using the conversion 300 excess cancer deaths per 1 μg/m3 diesel particulate mass. However, one must take into account the different distances involved and an estimated annual average correction in comparing RRAMP the ARB estimate. The additional data generated by the current studies has added additional information on the nature of the emissions from railyards (Sawant et al. Citation2007; Sheesley et al. Citation2008; Umezawa et al. Citation2008).
First, the presence of the very fine metals from the railyard pose an additional health threat. The concern is that many metals, including nickel, lead, and copper are increasing concentrations as the sizes become smaller (). We can anticipate that these concentrations could well be even higher in the important ultrafine mode with very high lung capture efficiencies. Recent studies in the Central Valley of California have tied these very fine metallic aerosols to ischemic heart disease (Cahill et al. Forthcoming)
Second, the presence of the contaminated soil from the railyard in the coarse modes can pose a particle deposition problem in local residences.
Finally, the PAHs from the locomotive diesel engines showed a very different trend in terms of the PAH profile compared to diesel engines used in trucks and other on-road applications (). The PAH profile from the locomotives had considerably higher concentrations of the heavy PAHs with molecular weights greater than 252 amu compared to the on-road diesel trucks and the NIST standard reference materials. This trend was observed with all 3 samples collected, namely the 8-stage DRUM and both of the Lundgren samples.
The most likely explanation for most of the size distribution is that our work was the first to our knowledge that used artifact minimizing stretched Teflon after-filter for organic speciation. The standard filter is fired quartz, and is roughly twenty times thicker in the areal density in μg/cm2 than the stretched Teflon filter that was identical to that used by IMPROVE, FRM, and many other networks. Fired quartz has a known and well documented positive artifact effect when volatile materials are present (Finalyson-Pitts and Pitts Citation2000; Watson et al. Citation2009). From mass 228 amu and below, an increasing proportion of the PAHs occur in the vapor phase, and thus depending on conditions could be collected as artifact by quartz filters. If this was the collection method for the NIST SRM, it would share the effect. Other effects may be contributory. Since both our data and the ARB 2004 analysis showed that a major fraction of the emissions came from idling diesel engines, this could be a factor in the molecular mass differences. It is also unclear if the locomotive emissions have a higher proportion of heavier PAHs arising from the large-bore engine lubricants or the locomotive fuel, which is different from the diesel fuel used in trucks. Finally, one possible explanation for different PAH profiles is that the lighter PAHs could “blow-off” of the sample during the long sample collection periods. We do not believe this to be a significant effect. First, an analysis of the alkanes shows negligible concentrations of heavy alkanes on the after-filter. Second, if “blow-off” was significant, then we would expect the samples exposed to the air stream for a longer period of time to have lower proportions of the lighter (178 and 202 amu) PAHs. This was not observed. The 8-stage DRUM sampler collected material for 6 weeks, in high temperature conditions, and its profile is very similar to the Lundgren samples that were only 1.5 weeks in duration and only operated at night. Therefore, the PAH profile does not appear to be affected by “blow-off” during sample collection.
The second important point is the size distribution of the PAHs. The Kelvin Effect demands that ultrafine PAHs have to be non-volatile to survive significant times in the atmosphere, so even if the original PAH emission mix looked like standard diesel emissions, but in the ultrafine mode, the lighter and more volatile PAHs would evaporate more readily. However, it is unclear if this process is rapid enough to cause the observed difference since the samplers were within 100 m of many of the sources. Whatever the reason for the difference, the presence of so much toxic PAHs in the ultrafine mode with highly efficient lung capture increases potential health impacts.
It is also necessary to determine the mass of PAHs in the aerosols, and/or the ratio as a fraction of total aerosol mass, fine mass, and/or EC, to allow estimates of aerosol impacts. The most direct way is to form the ratio of PAHs to the major diesel aerosol component, EC. This is not available from the NIST diesel SRM 1650a and SRM 2975, but these data are available from the Desert Research Institute Report to the National Renewable Energy Laboratory, 2004. (Zielenska et al. 2004). An additional source is from the work of Gertler et al. (Citation2003) in a report to the Health Effects Institute and publications (Gertler et al. Citation2003). For the Roseville data, EC was measured at the Denio and Pool sites through October 2005. These were calculated using both mass and EC, since the results were compatible when the results were combined.
A raw average of the fifteen values gives a ratio of the RRAMP benzo[a]pyrene to diesel trucks of 7.7 ± 3.5. However, we conclude that the Denio–Pool difference in EC is not a good match to the Denio-only PAHs. Removing these data, and weighing the averages by errors, we derive 5.5 ± 0.7, where the latter value is the Standard Error of the mean. This means that there was about 5.5 times more benzo[a]pyrene from the railyard aerosols than from diesel truck aerosols, per unit very fine/ultrafine mass and per unit EC. Details are in the Supplemental Information 5.
Mitigation of emissions sources and transport out of the railyard would have health benefits, and four classes of mitigation are proposed (Cahill et al. Citation2007); source reduction (new engines, better fuels, limitations on idling, … the focus of current mitigation), source to right of way fence (paving interior roads and using ground covers to reduce dust, vegetative barriers), right of way fence to receptors (distance, vegetative barriers), and at the receptors (filters for including indoor air mitigation). These could lower the still-elevated diesel cancer risk, reduce very fine inhalable metals, and lower coarse metal laden dusts.
CONCLUSIONS
The results of this study basically confirm the ARB's estimates of the impacts of the Roseville Railyard on the surrounding community while providing additional information on coarse and fine aerosols from the railyard on the community. The upwind–downwind and day–night study design effectively differentiated ambient aerosols from those emanating from the Roseville Railyard. Elevated NO ratios strongly suggested the diesel emissions from the railyard were the source for toxic aerosols present in the downwind samples. Coarse soils were contaminated with anthropogenic metals and petroleum-based n-alkane organic matter, and very fine (0.26 > D p > 0.09 μm) elements phosphorus, zinc, and sulfur, tracers for diesel emissions, were observed from the railyard. Regretfully, we do not have data on ultrafine metals. Benzo[a]pyrene, which is labeled as one of the most toxic PAHs, was detected at levels 5.5 ± 0.7 times as concentrated as in diesel truck exhaust per unit mass. In addition, the heavy PAHs were almost entirely in the ultrafine mode, enhancing lung capture.
uast_a_580796_sup_18686253.zip
Download Zip (462.4 KB)Acknowledgments
The authors wish to acknowledge the continual support, encouragement, and expert scientific guidance of the Health Effects Task Force (HETF) of Breathe California of Sacramento-Emigrant Trails, its Chair, Jan Sharpless, and its Consultant, Betty Turner. HETF is a long term (1994) standing committee of volunteer experts from state and local agencies, universities and medical organizations. It provides high level technical expertise to Breath California of Sacramento Emigrant Trails, a local NGO that has focused on protecting lungs from disease, smoking, and air pollution since 1917. (http://www.sacbreathe.org).The Detection and Evaluation of Long-range Transport of Aerosols (DELTA) Group is a primarily global climate research association headquartered at UC Davis (http://delta.ucdavis.edu) that lends its samplers, analytical capabilities, and expertise to problems of human health. The staff of the Placer County APCD, especially Yushuo Chang, Kurt Schreiber, and Mike Sims, who were exemplary in their support of our field analyses and their provision of meteorology and data from RRAMP. We would like to gratefully acknowledge EPA Region IX for funding the organic analysis (Meredith Kurpius, project manager).The authors also gratefully acknowledge the NOAA Air Resources Laboratory (ARL) for the provision of the HYSPLIT transport and dispersion model and/or READY website (http://www.arl.noaa.gov/ready.html) used in this publication. This report has benefited greatly from the comments and suggestions of the RRAMP Technical Advisory Committee, but the opinions expressed herein are solely those of the authors.
[Supplementary materials are available for this article. Go to the publisher's online edition of Aerosol Science and Technology to view the free supplementary files.]
Notes
aUnable to quantify compound due to analytical problem, namely excessive enrichment of chrysene-d 12 that saturated the ion trap mass spectrometer.
REFERENCES
- Abu-Allaban , M. , Coulomb , W. , Gertler , A. W. , Gillies , J. , Pierson , W. R. , Rogers , C. F. , Sagebiel , J. C. and Tarnay , L. 2002 . Exhaust Particle Size Distribution Measurements at the Tuscarora Mountain Tunnel . Aerosol. Sci. Tech. , 36 : 771 – 789 .
- Abu-Allaban , M. , Rogers , C. F. and Gertler , A. W. 2004 . A Quantitative Description of Vehicle Exhaust Particle Size Distributions in a Highway Tunnel . J. Air Waste Manage. Assoc. , 54 : 360 – 366 .
- ARB . 2010 . HTU . http://www.arb.ca.gov (in the section on Data and Statistics)
- Bench , G. , Grant , P. G. , Ueda , D. , Cliff , S. S. , Perry , K. D. and Cahill , T. A. 2002 . The Use of Stim and Pesa to Measure Profiles of Aerosol Mass and Hydrogen Content, Respectively, across Mylar Rotating Drums Impactor Samples . Aerosol. Sci. Tech. , 36 : 642 – 651 .
- Brandenberger , S. , Mohr , M. , Grob , K. and Neukomb , H. P. 2005 . Contribution of Unburned Lubricating Oil and Diesel Fuel to Particulate Emission from Passenger Cars . Atmos. Environ. , 39 : 6985 – 6994 .
- Cahill , T. A. 1979 . Comments on Surface Coatings for Lundgren-Type Impactors . Aerosol Meas. , : 131 – 134 .
- Cahill , T. M. 2010 . Size-Resolved Organic Speciation of Wintertime Aerosols in California's Central Valley . Environ. Sci. Technol. , 44 : 2315 – 2321 .
- Cahill , T. A , Barnes , D. E. , Spada , N. J. , Lawton , J. A. and Cahill , T. M. 2011 . Very Fine and Ultra-Fine Metals and Ischemic Heart Disease in the California Central Valley 1: 2003–2007 . Aerosol. Sci. Tech. , 45 : 1124 – 1134 .
- Cahill , T. A. , Cahill , T. M. , Barnes , D. E. , Spada , N. J. , Cliff , S. S. , Perry , K. D. and Fujii , E. 2007 . “ Mass, Organic, and Elemental Aerosols by size, time, and composition at the Union Pacific Rail Road's Roseville Railyard ” . In Final Report to the Health Effects Task Force (HETF), Breathe California of Sacramento-Emigrant Trails Sacramento, CA
- Cahill , T. A. , Goodart , C. , Nelson , J. W. , Eldred , R. A. , Nasstrom , J. S. and Feeney , P. J. 1985 . “ Design and Evaluation of the Drum Impactor ” . In Proceedings of International Symposium on Particulate and Multi-phase Processes Edited by: Ariman , T. and Veziroglu , T. N. 319 – 325 . Washington, D.C.
- Cahill , T. A. and Wakabayashi , P. 1993 . “ Compositional Analysis of Size-Segregated Aerosol Samples ” . In Measurement Challenges in Atmospheric Chemistry Edited by: Newman , L. 211 – 228 . American Chemical Society,Washington, , DC
- Campbell , D. and Fujita , E. M. 2009 . “ Roseville Rail Yard Air Monitoring Project (RRAMP) ” . In Final Report Summary of Data QA and Trend Analysis , Reno, NV : Desert Research Institute .
- Christofk , T. 2009 . Final Update Pertaining to the Mitigation Measures and Monitoring Activities for the Union Pacific Roseville Railyard . http://www.placer.ca.gov/apcd
- Chueinta , W. and Hopke , P. K. 2001 . Beta Gauge for Aerosol Mass Measurement . Aerosol. Sci. Tech. , 35 : 840 – 843 .
- DELTA Group . 2010 . http://delta.ucdavis.edu
- Finalyson-Pitts , B. and Pitts , J. 2000 . Chemistry of the Upper and Lower Atmosphere , San Diego, CA : Academic Press . (Chapter 10, Figure 10.3)
- Gertler , A. , Abu-Allaban , M. , Coulombe , W. , Gillies , J. A. , Pierson , W. R. , Rogers , F. C. , Sagebiel , J. C. , Tarnay , L. and Cahill , T. A. 2003 . Measurements of Mobile Source Particulate Emissions in a Highway Tunnel . Int. J. Vehicle Des. , 27 : 86 – 93 .
- Hand , R. , Di , P. , Servin , A. , Hunsaker , L. and Suer , C. 2004 . Roseville Rail Yard Study , Sacramento, CA : California Air Resources Board .
- Kilburn , K. H. 2000 . Effects of Diesel Exhaust on Neurobehavioral and Pulmonary Functions . Arch. Environ. Health , 55 : 11 – 17 .
- Lundgren , D. A. 1967 . An aerosol sampler for determination of particulate concentration as a function of size and time . J. Air Pollut. Control Ass. , 17 : 225 – 229 .
- Miller , R. 2002 . Ultraviolet Extinction in Boundary Layer Aerosols: Chromatic Variations in Size and Time Resolved Particulate Matter Collected on Teflon Filters and Greased Mylar Strips . PhD diss, University of California, Davis
- Parent , M. E. , Rousseau , M. C. , Boffetta , Cohen , P. A. and Siemiatycki , J. 2007 . Exposure to Diesel and Gasoline Engine Emissions and the Risk of Lung Cancer . Am. J. Epidemiol. , 165 : 53 – 62 .
- Raabe , O. G. , Braaten , D. A. , Axelbaum , R. L. , Teague , S. V. and Cahill , T. A. 1988 . Calibration Studies of the Drum Impactor . J. Aerosol Sci. , 19 : 183 – 195 .
- Riddle , S. G. , Robert , M. A. , Jakober , C. A. , Hannigan , M. P. and Kleeman , M. J. 2007 . Size Distribution of Trace Organic Species Emitted from Heavy-Duty Diesel Vehicles . Environ. Sci. Technol. , 42 : 974 – 976 .
- Rolph , G. D. 2003 . Real-time Environmental Applications and Display System (READY) , Silver Spring, MD : NOAA Air Resources Laboratory . http://www.arl.noaa.gov/ready/hysplit4.html
- Sawant , A. A. , Nigam , A. , Miller , J. W. , Johnson , K. C. and Cocker , D. R. 2007 . Regulated and Non-Regulated Emissions from in-Use Diesel-Electric Switching Locomotives . Environ. Sci. Technol. , 41 : 6074 – 6083 .
- Sheesley , R. J. , Schauer , J. J. , Smith , T. J. , Garshick , E. , Laden , F. , Marr , L. C. and Molina , L. T. 2008 . Assessment of Diesel Particulate Matter Exposure in the Workplace: Freight Terminals . J. Environ. Monitor. , 10 : 305 – 314 .
- Umezawa , M. , Sakata , C. , Tabata , M. , Tanaka , N. , Kudo , S. , Takeda , K. , Ihara , T. and Sugamata , M. 2008 . Diesel Exhaust Exposure Enhances the Persistence of Endometriosis Model in Rats . J. Health Sci. , 54 : 503 – 507 .
- Watson , J. G. , Chow , J. C. , Chen , L. W. A. and Frank , N. H. 2009 . Methods to Assess Carbonaceous Aerosol Sampling Artifacts for Improve and Other Long-Term Networks . J. Air Waste Manage. , 59 : 898 – 911 .
- Weast , R. C. 1989 . “ 70th ed ” . In CRC Handbook of Chemistry and Physics: A Ready-Reference Book of Chemical and Physical Data , Boca Raton, FL : CRC Press . (Table F-143)
- Wesolowski , J. J. , John , W. , Devor , W. , Cahill , T. A. , Feeney , P. J. , Wolfe , G. and Flocchini , R. 1978 . “ Collection Surfaces of Cascade Impactors ” . In X-Ray Fluorescence Analysis of Environmental Samples , Edited by: Dzubay , T. 121 – 130 . Ann Arbor Science Publishers .
- Zielinska , B. , Goliff , W. , McDaniel , M. , Cahill , T. , Kittelson , D. and Watts , W. 2003 . Chemical Analyses of Collected Diesel Particulate Matter Samples in the E-43 Project , Ann Arbor, MI : National Renewable Energy Laboratory .
- Zielinska , B. , Sagebiel , J. , McDonald , J. D. , Whitney , K. and Lawson , D. R. 2004 . Emission Rates and Comparative Chemical Composition from Selected in-Use Diesel and Gasoline-Fueled Vehicles . J. Air Waste Manage Assoc. , 54 : 1138 – 1150 .