Abstract
In this study, we compare the chemical and oxidative characteristics of concentration-enriched PM2.5 samples simultaneously collected by a filter, a Nano-Micro-Orifice Uniform Deposition Impactor, and a BioSampler. Gravimetric measurements showed considerable agreement in particulate matter (PM) collection efficiency for all three samplers. Accordingly, samples from the three collectors exhibited similar chemical compositions. The mass fractions of their inorganic ions, labile and nonlabile, were comparable. Moreover, the organic carbon (OC) content of the BioSampler slurry was similar to that of the filter, while water-soluble OC levels of the filter and impactor samples were close to a 100% agreement. Lastly, linear regression analyses demonstrated that the water-soluble elements existed in similar proportions for the filter and impactor samples. Their respective total components were also in very good agreement. By contrast, the recoverable elements from the BioSampler slurry, determined by high-resolution magnetic sector inductively coupled plasma mass spectrometry, were in good agreement with the water-soluble elements of the filter and impactor samples but not their corresponding total components. In spite of the overall agreement among the samples on their chemical composition, findings from a macrophage reactive oxygen species (ROS), a dithiothreitol (DTT), and a dihydroxybenzoate (DHBA) assay revealed that the oxidative potential of aqueous extracts of the filter and impactor substrates was similar yet substantially lower than that of the BioSampler slurry. However, filtering of the BioSampler slurry, i.e., removal of insoluble PM components, attenuated its ROS activity to about the same level as that of the water extracts of the filter and impactor samples. These findings first indicate that insoluble PM species are potentially redox active, and second that particle collection by the BioSampler, which circumvents the need for PM extraction, constitutes a viable alternative for collecting concentrated particles for characterization of the oxidative properties of PM.
1. INTRODUCTION
Ambient particulate pollution has been of primary interest to epidemiological research. Relevant studies correlated increased exposure to airborne particulate matter (PM) with adverse health effects in humans (Delfino et al. Citation2005; Pope and Dockery Citation2006). However, despite the significant evidence, a clear identification of the PM properties eliciting its acute responses remains to be found (Vedal Citation1997). An emerging hypothesis is that many of the health risks derive from the ability of PM to exert oxidative stress, initiated by the formation of reactive oxygen species (ROS) within and on the surface of affected cells (Becker et al. Citation2002; Baulig et al. Citation2003; Donaldson et al. Citation2003). The production of ROS changes the redox status of the cell, thereby inducing inflammation-related disorders (Li et al. Citation2002; Schafer et al. Citation2003). In addition, it was particularly found that the toxicity of PM is linked to its chemical composition (Hetland et al. Citation2004; Jalava et al. Citation2009). Therefore, efficient PM collection and extraction for measurement of its oxidative potential are of crucial importance.
Conventional PM sampling methodologies consist of filtration and impaction. In these techniques, PM is first collected on substrates or filters and then removed into suspension for in vitro assays. However, these methods are associated with a variety of drawbacks. First, if deionized ultrapure water is used for particle removal, insoluble PM-bound species are unlikely to be extracted. These species may have a considerable toxicological potential. Alternatively, organic solvents can be used. Nonetheless, these solvents must be removed prior to the bioassay, which may remove some potentially toxic PM-bound labile species such as semivolatile organics. Moreover, if acid solvents are used, metals and elements are solely extracted. This procedure also converts these components to soluble forms, which may alter their toxicity, and tends to oxidize organics to carbon dioxide. Second, sonication and vortexing during extraction may induce physical changes and alter the biological properties of PM (Dick et al. Citation2000). Finally, sampling artifacts have been widely related to the use of filters and impactors. These include loss of labile species such as ammonium nitrate and organics (Cheng and Tsai Citation1997; Eatough et al. Citation2003), adsorption of vapor phase organics (Turpin et al. Citation1994), and reactions between particles and incoming gases (Tsapakis and Stephanou Citation2003).
To overcome some of the limitations of filtration and impaction, novel approaches employ a liquid impinger PM collector (BioSampler, SKC West, Inc., Fullerton, CA) in tandem with a particle concentrator (Versatile Aerosol Concentration Enrichment System [VACES]; Kim et al. 2001a, Citation2001b). In this method, concentration-enriched enlarged particles are injected into a swirling flow and then collected by a combination of impaction and centrifugal forces. Using a PM concentrator is necessary because of the characteristics of the BioSampler. At its nominal flow rate of 12.5 Lpm, the BioSampler has a collection efficiency close to 100% for particles larger than 1.5 μm. But when operated in conjunction with a PM concentrator, where even ultrafine particles are grown to supermicrometer droplets, a BioSampler effectively collects any size range particles (Kim et al. Citation2001b). Particle concentration also provides enrichment factors that can be as high as 40 without significantly affecting PM properties, such as size (Misra et al. Citation2004), bulk chemistry (Kim et al. Citation2001a; Khlystov et al. Citation2005), or single particle chemistry (Zhao et al. Citation2005) and morphology (Kim et al. Citation2001a). This direct collection of a highly concentrated liquid suspension eliminates uncertainties related to PM extraction and is particularly useful for oxidative assays. Additionally, the inertia-based PM collection mechanism of the BioSampler resembles particle deposition in the human respiratory tract, thereby rendering this method representative of “real-world” exposure to atmospheric particles. Given the substantial sampling and extraction discrepancies in the aforementioned techniques, bioassays for PM collected by these methods may yield dissimilar results. For instance, outcomes could be similar if the bioactivity is derived from water-soluble PM species, but different if obtained from nonwater-soluble compounds. To the best of the authors’ knowledge, no studies were found in the literature that systematically evaluated the properties of PM collected by these various sampling methods. In this study, we compare the sampling techniques of filtration, impaction, and concentrator-BioSampler tandem. This was accomplished by concurrently collecting ambient PM2.5 (aerodynamic diameter <2.5 μm) samples via these three methods. The chemical and oxidative properties of the PM samples were then examined by conducting the chemical speciation and oxidative assays. The connection between the redox activity and the water-soluble as well as insoluble PM content of the samples was also investigated in order to assess their role in the particle oxidative activity. This is pivotal as these species have different cellular targets and pathways in the respiratory tract during “real-life” exposure. Results from this study provide additional insights into PM collection by filters, impactors, and concentrator-BioSamplers for chemical and oxidative assays. Characterization of these systems and their limitations will ultimately aid in elucidating PM-induced health risks.
2. METHODS
2.1. Sample Collection
For the purpose of comparing filtration, impaction, and concentrator-BioSampler tandem sampling methods, concentrated ambient PM2.5 samples were simultaneously collected by using a filter, a Nano-Micro-Orifice Uniform Deposition Impactor (Nano-MOUDI, MSP Corp., Minneapolis, MN), and a BioSampler. Concentrating these fine-mode particles was necessary to enable their collection by the BioSampler, as aforementioned, with the additional advantage of reducing the sampling time required to collect PM loadings sufficient for chemical and oxidative analyses. The collection of samples was conducted at the Particle Instrumentation Unit (PIU) of Southern California Particle Center (SCPC) near the main campus of the University of Southern California, Los Angeles, CA. The site is located about 130 m to the NE of I-110 freeway in Los Angeles (Ning et al. Citation2007). Sampling lasted for 5 h a day and was carried out during weekdays in the period of January to March 2010. To ensure reproducibility and reliability of the results, three replicates of the experiment were conducted. Accordingly, three sets each of filter, impactor, and BioSampler samples were collected in total.
Two side-by-side VACES were used to provide concentration-enriched ambient PM2.5 to the three collectors as illustrated in . Development and evaluation of the VACES are described in detail in Kim et al. (2001a, Citation2001b). Briefly, each VACES consists of three parallel sampling lines where ambient PM2.5 is first pulled through a saturation-condensation system and then separately drawn in each line at a flow rate of 110 Lpm. As a result of supersaturation, the sampled aerosol particles were grown to 2.5–3 μm droplets. The individual streams of droplets were then concentrated by passing them through their respective virtual impactors (VIs) of cutpoint 1.5 μm at the intake flow rate of 110 Lpm. Concentrated droplets then exited their corresponding VI at the minor flow rate of 5 Lpm. Output minor flows from the two VACES were subsequently combined to one 30 Lpm flow. Next, the 30 Lpm output flow was split into three parallel streams each of 10 Lpm flow rate. Two of these sampling lines were then separately passed through two diffusion dryers (TSI Model 3062, TSI, Inc., St. Paul, MN) to dehydrate the grown particles to their original size. One of these diffusion dried streams was hereafter split into two equal halves of 5 Lpm, each directed to a filter holder consisting of either a 37-mm Teflon filter (Teflo, Pall Corp., Life Sciences, 2-μm pore, Ann Arbor, MI) or a 37-mm prebaked quartz filter (Whatman International Ltd., Maidstone, England) to collect PM2.5 by filtration. The other 10 Lpm diffusion dried stream was pulled into a Nano-MOUDI operated with three impaction stages loaded with 47-mm Teflon filters (Teflo, Pall Corp., Life Sciences, 2-μm pore, Ann Arbor, MI) and having cutpoints of 2.5, 0.312, and 0.018 μm. Therefore, only substrates of the second and third stages were analyzed for collection by impaction of the ambient PM2.5 samples. It should however be noted that gravimetric analysis showed that the mass collected on the first stage was negligible, confirming that only PM2.5 was drawn through the sampling inlet. On the other hand, the remaining 10 Lpm stream was split and directly pulled through two identical preautoclaved BioSamplers each operating at 5 Lpm rather than their standard flow rate of 12.5 Lpm. The decrease in flow rate was essential for reducing the pressure drop across the BioSampler from 36.1 to 4.2 kPa (Kim et al. Citation2001b). Hence, evaporation of PM after its condensational growth can be minimized (Kim et al. Citation2001b). Moreover, despite the decrease in flow rate, which would increase the cutpoint of the BioSampler, its collection efficiency remains close to 100% for particles larger than 2 μm (Kim et al. Citation2001b). Therefore, the supermicrometer-enlarged and concentration-enriched fine particles were effectively collected by the BioSamplers. Furthermore, for validation purposes, the collection efficiency of the BioSamplers was determined by placing a 37-mm Teflon “after-filter” downstream of each one of them.
2.2. Gravimetric Analysis
For the measurement of particle mass concentration, the Teflon filters from filtration and impaction were preweighed and postweighed after equilibration by using a Mettler 5 Microbalance (Mettler Toledo, Inc., Highstown, NJ) in a room with controlled temperature of 21°C–24°C and relative humidity of 40%–50%. Particle mass concentrations of the filter and Nano-MOUDI samples were then evaluated as the ratio of their total mass loading to the volume sampled. The particle mass concentration of the BioSampler suspension was determined as the difference in the mass gains of the 37-mm Teflon filter and the BioSampler “after-filter.”
2.3. Chemical Analyses
For each set of samples, the Teflon filters of the filtration and impaction methods were cut into several sections, and the BioSampler slurry was divided into multiple subslurries, with separate filters sections and subslurries used for the chemical and oxidative assays. The quartz filter of the filter-based method was cut into two sections for total and water-soluble organic carbon (WSOC) analyses. The PM2.5 samples were subsequently analyzed for inorganic ions, organic carbon (OC) (except for impaction samples), and total elements. Furthermore, WSOC and water-soluble elements on the filters and impactor substrates were determined. To conduct these chemical analyses, water extractions of the cut filters sections were performed for the water-soluble ions, water-soluble elements, and WSOC, whereas acid extraction was conducted for the total elemental analysis.
Prior to the ionic and water-soluble elemental analysis, the Teflon filters sections were immersed in screw-cap polypropylene tubes containing 15 mL of Milli-Q water. The tubes were placed in a shaker table for 6 h at 25°C, in the dark, with continuous agitation. Samples were then filtered using 0.45-μm polypropylene syringe filters and aliquots dispensed for the measurement of water-soluble ions and elements. The inorganic ion composition was determined by ion chromatography (IC). Measured ions included chloride, sodium, nitrate, ammonium, and sulfate. The water-soluble inorganic element concentrations of the PM2.5 samples were measured using high-resolution magnetic sector inductively coupled plasma mass spectrometry (SF-ICP-MS, Thermo-Finnigan Element 2; Zhang et al. Citation2008). A total of forty-nine elements were quantified. A separate water extraction was performed for the quantification of WSOC. Sections of the quartz filters and Teflon impaction substrates were extracted in 12 mL of Milli-Q water, for 16 h, with shaking, in ashed glass vials. Extracts were then filtered with a glass fiber (GF) syringe filter (0.7 μm) and polypropylene syringe barrel, and subsequently measured using a Sievers 900 Total Organic Carbon Analyzer (Stone et al. Citation2009). For the determination of total elements in PM collected on the Teflon filters and impaction substrates, a microwave-aided Teflon bomb digestion protocol using a mixture of 1 mL of 16 M nitric acid, 0.250 mL of 12 M hydrochloric acid, and 0.10 mL of hydrofluoric acid was used. Total OC levels were only quantified from the quartz filter sections by the National Institute for Occupational Safety and Health (NIOSH) Thermal Optical Transmission (TOT) method (Birch and Cary Citation1996). The Teflon filters from the impactors were not suitable for TOT analysis.
The BioSampler subslurries were directly analyzed, without filtration, for their ionic and OC content, using IC and the Sievers 900 Total Organic Carbon Analyzer, respectively. Their elemental composition was determined after acidification with nitric acid (5% v/v) and then direct analysis by SF-ICP-MS without further digestion.
2.4. Oxidative Assays
The oxidative capacity of the PM2.5 samples was assessed by means of three independent methods: a macrophage-based ROS assay and two chemical approaches, the dithiothreitol (DTT) and the dihydroxybenzoate (DHBA) assays, as described in the following sections.
2.4.1. ROS Assay
Prior to the ROS assay, sections of the same Teflon filters and impaction substrates used in the chemical analyses were extracted with 1.0 mL of Milli-Q water for 16 h, in the dark on a shaker table, and filtered through 0.22-μm filters. To investigate the contribution of insoluble PM species to the redox activity, the macrophage ROS assay was applied to the BioSampler subslurries, which were allocated for this assay, directly (without filtration) and after their filtration. The filtration process was performed using a 13-mm Whatman Puradisc syringe filter (0.22-μm polypropylene). Prior to use, the syringe was washed with dilute hydrochloric acid and then rinsed with Milli-Q water. Depending on the overall slurry volume, aliquots of 1, 3, or 5 mL were filtered, discarding the first ∼100 μL of the filtrate, with the rest collected for the ROS analysis. It should also be noted that no attempt was made to directly evaluate the ROS activity of the insoluble PM components. In that event, the unfiltered particles would be resuspended and analyzed for their activity, while bypassing any uncertainties or difficulties associated with PM removal from filters and substrates. However, in this study, comparisons are only established between filtered and unfiltered slurry samples.
The macrophage ROS assay is a fluorogenic cell-based method that assesses the ROS production by PM. Experiments were performed with the rat alveolar cell line, NR8383, obtained from the American Type Culture Collection (ATCC). This highly responsive alveolar macrophage cell line exhibits all the characteristics of normal primary macrophage cells, responding to appropriate microbial, particulate, or soluble stimuli with phagocytosis and killing. NR8383 cells were maintained in Hams F12 medium containing 2 mM l-glutamine supplemented with 1.176 g/L sodium bicarbonate and 15% heat-inactivated fetal bovine serum. Cells were cultured at 37°C in a humidified 5% CO2 incubator and maintained by transferring nonadherent cells to new flasks weekly. Cultures were set up to contain a floating cell concentration of approximately 4 × 105 cells/mL of media. Cultured nonadherent macrophage cells were harvested and gently concentrated by centrifugation at 750 rpm for 5 min; the culture media were removed and replaced with salts glucose medium (SGM) to produce a cell suspension of 1000 cells/μL. Macrophage cells (100 μL = 100,000 cells) were dispensed into each well of a ninety-six-well plate and incubated for 2 h in a 37°C incubator under a 6% CO2 atmosphere. Approximately 15 min before the end of the incubation period, 2′,7′-dichlorodihydroflourescein diacetate (DCFH-DA) was added to each prepared sample to achieve a final concentration of 45 μM. After the incubation period, during which time more than 98% of the cells have settled and adhered to the well bottom, the SGM was pipetted off and immediately replaced with 100 μL of the SGM-buffered sample extract or control sample. The plate was returned to the 37°C incubator for 2.5 h after which time the fluorescence intensity of each well was determined at 504-nm excitation and 529-nm emission (515-nm cutoff) by using a m5e microplate reader (Molecular Devices, Inc., Sunnyvale, CA). Further details can be found in Landreman et al. Citation(2008).
2.4.2. DTT Assay
The DTT assay was conducted on ultrapure water extracts of the remaining Teflon sections of the filtration and impaction samples. The samples were agitated for 10 min in a vortex mixer, then subjected to 20 min of sonication, and then followed by a second 10-min agitation in the vortex mixer. On the other hand, this assay was directly applied, without filtration, to the BioSampler subslurries dispensed for this assay.
This chemical assay measures the redox activity of a sample based on its ability to transfer electrons from DTT to oxygen (Cho et al. Citation2005; Ayres et al. Citation2008). This process generates the superoxide radical that subsequently comproportionates to hydrogen peroxide and oxygen. The rate of this reaction is monitored by the consumption of DTT under standardized conditions and is proportional to the concentration of the redox-active species in the PM sample (Cho et al. Citation2005). Compounds capable of this reaction have generally been limited to organic species, such as polycyclic aromatic hydrocarbons (PAHs) and quinones (Cho et al. Citation2005; Ayres et al. Citation2008), but high concentrations of transition metal ions can also oxidize DTT (Netto and Stadtman Citation1996; Kachur et al. Citation1997).
2.4.3. DHBA Assay
The DHBA assay was performed on aliquots of the same filter and impactor water-extracted samples that were used for the DTT assay. It was also directly applied, without filtration, to the remaining BioSampler subslurries.
This analytical procedure quantifies the metal-based redox activity by measuring the ability of transition metals, such as iron and copper, associated with PM to catalyze the Fenton reaction in which hydrogen peroxide is converted to the hydroxyl radical. Ascorbic acid is added to reduce oxidized metals and to generate hydrogen peroxide by the reduction of oxygen. Hydroxyl radical, formed from the conversion of hydrogen peroxide, will then react rapidly with salicylic acid to form 2,3- and 2,5-DHBA. Redox-active organic compounds such as quinones consume ascorbate but do not generate DHBA. Therefore, as the production of DHBA is metal dependent, organic redox species will not interfere with this assay (Ayres et al. Citation2008; DiStefano et al. Citation2009).
3. RESULTS AND DISCUSSION
3.1. Gravimetric Composition
The PM2.5 mass concentrations of the three collected sets of filter, Nano-MOUDI substrate, and unfiltered BioSampler slurry are shown in . As can be inferred from the data, the samples from all the collectors exhibited set-to-set variability driven by changes in ambient levels of PM2.5. However, for each set, the mass concentrations of the samples were similar, indicating very comparable PM2.5 collection by all three methods. This quite remarkable agreement among devices can be first attributed to the effect of concentration enrichment on the collected PM. Filters and impactors are commonly associated with volatilization losses of labile species (Cheng and Tsai Citation1997; Eatough et al. Citation2003). But the use of particle concentrators has been shown to significantly reduce volatilization artifacts of their collected PM. In particular, losses of semivolatile nitrate, a major component of ambient fine PM in Los Angeles (Lawson Citation1990; Hering et al. Citation1997), can be reduced to less than 10% (Chang et al. Citation2000). Moreover, the concentration-enriched slurry and the low-pressure drop (4.2 kPa) of the BioSampler minimized losses from its particle suspension (Kim et al. Citation2001b). Second, the similarity in total PM collection among the samplers can also be related to the efficient trapping of PM2.5 by the BioSampler. Although the BioSampler was operated at a reduced flow rate, which is expected to increase its cutpoint, “after-filter” gravimetric measurements indicated that over 95% of PM2.5 by mass is captured by the sampler.
3.2. Chemical Composition
3.2.1. Inorganic Ions
The samples were analyzed for five major inorganic ions: nitrate, ammonium, sulfate, sodium, and chloride. shows the average mass fractions of the measured ions for the filter, impactor substrate, and unfiltered BioSampler slurry. The mass fraction is defined as the mass ratio of a given species to that of PM2.5. Error bars represent their associated standard errors. Results show that the mass fractions of individual ions are about the same for all samplers. These findings then indicate efficient extraction and, most importantly, collection of all the ionic species, even labile ammonium nitrate, by the three methods. The levels of ammonium and nitrate for the filter were 14%–22% lower than those for the impactor substrate and BioSampler slurry. Considering uncertainties in the analyses and measurements, this can be considered as a reasonably good agreement. This agreement can be associated with the PM concentration enrichment process, which, as noted earlier, diminishes volatilization losses of particulate nitrate and ammonium from the filter and impactor samples from about 50%–70% to less than 10% (Chang et al. Citation2000). Similarly, at the reduced flow rate of 5 Lpm, the highly concentrated liquid suspension of the BioSampler and its small pressure drop of 4.2 kPa minimize volatilization losses of these species (Kim et al. Citation2001b). These results confirm the findings of Chang et al. Citation(2000) who showed that when used in combination with a particle concentrator, the samplers are reliable collectors of labile ionic species in PM. In addition, all three samplers effectively collected the nonvolatile sodium and sulfate ions. The average mass fractions of these species were in fairly good agreement among the concomitant samplers in spite of their low values.
3.2.2. Organic Carbon
To investigate the adequacy of the collectors for bulk organic matter sampling, the OC or water-soluble OC content of the filter, Nano-MOUDI substrate, and unfiltered BioSampler slurry was determined as illustrated in . Plotted for each of the samplers are the average mass fractions of WSOC or OC and their respective standard errors. Results reveal a very good agreement of WSOC between the filter and Nano-MOUDI substrates. This agreement can, again, be attributed to a reduction in evaporative losses by means of particle concentration enrichment (Chang et al. Citation2000) as well as the similarity in sample treatment and extraction. Both the filtration and impaction substrates were water extracted using comparable protocols prior to their WSOC measurement. On the other hand, interestingly, the OC content of the BioSampler slurry was quite comparable (i.e., within 15%) to the total OC level of the filter, but greater than its WSOC content. Therefore, both WSOC and water-insoluble OC (WIOC) were retained in the BioSampler liquid suspension. These results indicate that the concentrator-BioSampler tandem method constitutes an effective and direct technique for collecting and recovering both WSOC and WIOC.
FIG. 4 Average mass fractions of OC and WSOC in PM2.5 samples from the filter, Nano-MOUDI, and BioSampler (unfiltered slurry). Error bars represent one standard error.
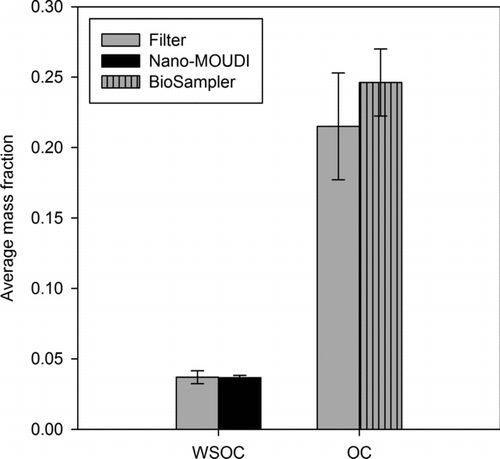
FIG. 5 (a) Linear regression analysis between the average mass fractions of water-soluble elements in PM2.5 samples from the filter and Nano-MOUDI. Standard errors are shown in parentheses. Plot is presented on a log-log scale. (b) Linear regression analyses between the average mass fractions of water-soluble and recoverable elements in PM2.5 samples from the filter–BioSampler (unfiltered slurry) and Nano-MOUDI–BioSampler (unfiltered slurry). Standard errors are shown in parentheses. Plots are presented on a log-log scale.
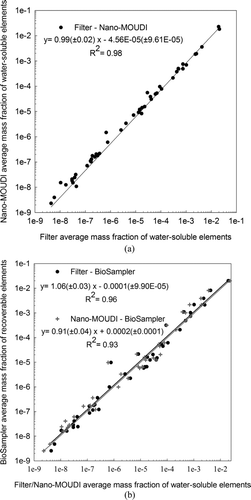
3.2.3. Metals and Trace Elements
displays a correlation plot between the average mass fractions of water-soluble elements from the filter and Nano-MOUDI substrate measurements. Linear regression analysis showed an excellent agreement between the two collectors as demonstrated by the slope of 0.99 and the regression coefficient (R 2) of 0.98. This agreement also extends over a myriad of elements (a total of 49) and spans 8 orders of magnitude, with similar average (± standard error) total elemental mass fraction between the filter and impactor substrates (53.26 ± 17.31 and 47.82 ± 11.84 μg/mg, respectively). This agreement indicates comparable collection efficiency of water-soluble elements by the filtration and impaction methods as well as robust PM digestion and SF-ICP-MS analysis methods. The levels of water-soluble elements from both the filter and Nano-MOUDI substrates and the compositions of elements measured in the unfiltered BioSampler slurry also agree very well, as shown by their correlation plots in . Linear regression yielded near-unity slopes of 1.06 and 0.91 between the average mass fractions of the BioSampler and filter as well as the BioSampler and Nano-MOUDI samples, respectively. High regression coefficients of 0.96 and 0.93 were obtained in both cases as well. The average total elemental mass fraction and associated standard error were also comparable between the filter and BioSampler (53.26 ± 17.31 and 51.12 ± 11.94 μg/mg, respectively) in addition to the Nano-MOUDI and BioSampler (47.82 ± 11.84 and 51.12 ± 11.94 μg/mg, respectively) samples. This high similarity between the recoverable elements from the BioSampler slurry and the water-soluble elements from the filter and Nano-MOUDI substrates implies an incomplete extraction of all elements from the BioSampler suspension.
The average mass fractions of total elements measured from the filter and Nano-MOUDI substrates as well as elements recovered from the unfiltered BioSampler slurry are plotted in . Also shown is the average water-solubility fraction of elements as evaluated from the mass ratio of water-soluble to total elements obtained from the filter analyses. The overlapping profiles of the filter and Nano-MOUDI data indicate an excellent agreement between the filtration and impaction sampling methods for total elements, similar in agreement to that observed for their respective water-soluble components. However, these profiles do not match the ones obtained from SF-ICP-MS analysis on the BioSampler slurry. The mass fractions of elements recovered from the BioSampler suspension fall below those from the filter and impactor substrates, with generally greater divergence observed for elements of low water solubility. These results further indicate an incomplete extraction of elements from the BioSampler slurry. This can be attributed to the incomplete solubilization of the slurry PM with the method used to prepare the slurry for SF-ICP-MS analysis. Acidification to 5% nitric acid, while appropriate for metals associated with labile phases (e.g., carbonates, OC), is not sufficiently rigorous to completely solubilize more resistant phases. Moreover, particles greater than 0.2 μm are not quantitatively atomized in the plasma, and therefore, associated elements will likely exhibit poor recovery. Lastly, particle losses during sample storage, freezing, and thawing of the slurries might have also contributed to the lower elemental levels observed for the BioSampler slurry. Follow-up experiments are needed to identify the nature of the elemental losses from the BioSampler slurry.
3.3. Redox Activity
ROS are typically formed through PM-catalyzed electron transfers from cellular reductants, such as nicotinamide-adenine dinucleotide phosphate, to molecular oxygen. Initially, the formation of cellular ROS primarily consists of the superoxide radical produced in the mitochondria that subsequently comproportionates to hydrogen peroxide (Xia et al. Citation2006; Ayres et al. Citation2008). Although not analyzed in this study, organic PM components, such as quinones and PAHs, are capable of this catalytic reduction (Brunmark and Cadenas Citation1989; Squadrito et al. Citation2001). Hydrogen peroxide can then be further reduced to the highly reactive hydroxyl radical by transition metals, such as copper and iron, in the Fenton reaction (Ayres et al. Citation2008; Gottipolu et al. Citation2008; DiStefano et al. Citation2009).
The redox activities of the BioSampler PM slurry and the water extracts of the filter and impactor PM samples were compared by three independent assays consisting of the biological ROS in addition to the chemical DTT and DHBA assays. The objective of these comparisons is to characterize the contribution of insoluble particles to the overall PM-induced redox activity.
3.3.1. ROS Assay
PM components implicated in the formation of ROS include transition metals (Goldsmith et al. Citation1998; Prahalad et al. Citation1999) and organic species (Kumagai et al. Citation2002; Chung et al. Citation2006), both of which exist in soluble and PM-bound forms. The relationship between the ROS activities of the PM2.5 suspensions and their water-soluble as well as insoluble PM contents is discussed hereafter.
The average ROS activities of the three PM2.5 suspensions are displayed in , expressed as μg of Zymosan units per mg of analyzed PM. Their standard errors are also reported as error bars. The mean activities of the filtered aqueous extracts of the filter and Nano-MOUDI substrates were comparable (3325.10 ±106.58 and 4210.80 ± 463.30 μg of Zymosan units per mg of PM, respectively) but both were significantly lower than the activity of the unfiltered BioSampler slurry (6126.10 ± 815.47 μg of Zymosan units per mg of PM). The ROS activity of the latter was 1.5–1.8 times greater than the activities of the filtered water extracts of the filter and Nano-MOUDI PM samples. However, filtering of the BioSampler slurry, i.e., removing insoluble PM species, attenuated its redox activity to about the same level as that of the filter and Nano-MOUDI extracts. These results first indicate that the ROS activity of the PM2.5 samples is only partly driven by water-extractable species. The participation of water-soluble metals in ROS induction has been documented in previous studies, in which strong correlations between water-soluble transition metals (e.g., V, Fe, Ni, and Cr) and ROS formation (Hu et al. Citation2008; Verma et al. Citation2009a, Citation2009b) were reported. As such, it is likely that redox-active metals were important drivers of the PM ROS activity measured in this study. Second, these findings clearly demonstrate a measurable and significant contribution of insoluble species to the overall PM2.5 redox activity. The redox activity involving insoluble transition metals (e.g., Fe, Mn, Co, and Ti) and OC has been documented (Prahalad et al. Citation1999; Hu et al. Citation2008). Accordingly, the BioSampler, which circumvents the need for PM removal from collection substrates, constitutes a viable alternative for collecting concentrated particles for measurement of the PM ROS activity.
3.3.2. DTT Assay
This assay uses the DTT consumption rate, which expresses the catalytic reduction of oxygen by PM, as a measure of the particle redox activity (O’Brien Citation1991). Organic species, such as PAH and quinones (O’Brien Citation1991; Kumagai et al. Citation2002), have been primarily associated with the DTT reaction, but high concentrations of transition metals can also oxidize DTT (Netto and Stadtman Citation1996; Kachur et al. Citation1997).
The redox activity of the PM2.5 suspensions was assessed by examining their DTT consumption rates as displayed in on a per milligram basis of analyzed PM. The DTT activity of the water extracts of the filter and impactor PM samples was similar (27.5 ± 1.8 and 24.4 ± 2.6 nmol/min/mg of PM, respectively) yet roughly 60% lower than that of the unfiltered BioSampler slurry. These findings suggest that redox-active insoluble organic compounds and possibly metals, not effectively extracted from the filtration and impaction PM samples, significantly contributed to the overall DTT activity of the BioSampler slurry. These potent PM compounds thus seem to play a key role in inducing the DTT activity and therefore particle redox activity.
3.3.3. DHBA Assay
This assay evaluates the transition metal-based redox activity associated with PM by measuring the rate of formation of DHBA. As previously described, the rate of formation of DHBA is an indirect measure of the ability of transition metals to catalyze the Fenton reaction. The use of Fenton chemistry and the DHBA formation reaction to determine oxidative stress is well established (Coudray and Favier Citation2000; Liu et al. Citation2003) and has been applied to the PM samples (E. DiStefano et al. Citation2009).
As portrayed in , and similar to the observed variation in ROS and DTT activities, the DHBA formation rate, on a per-milligram basis of analyzed PM, changed with the nature of the assayed samples. The water extracts from the filtration and impaction PM samples had similar rates (14.7 ± 2.7 and 19.2± 5.8 nmol DHBA/min/mg of PM), while the unfiltered BioSampler slurry had a 2–2.6 times higher rate. Unlike soluble metals, insoluble metals were likely not effectively recovered in the aqueous extracts of the filter and Nano-MOUDI PM samples. Thus, the imbalance in DHBA formation rates implies the possible role of insoluble transition metals in the PM2.5 redox activity.
4. SUMMARY AND CONCLUSIONS
In this study, we compare the chemical and oxidative characteristics of concentration-enriched PM2.5 samples concurrently collected via a filter sampler, a Nano-MOUDI, and a BioSampler. The overall agreement on PM collection efficiency and chemical composition was observed among the different samples. In contrast, dissimilarities in PM oxidative properties were apparent between the BioSampler slurry and aqueous extracts of the filter and impactor samples.
The general good agreement on PM chemical composition among the methods is mainly attributed to minimized volatilization artifacts by means of concentration enrichment. The compositions of both labile and nonlabile inorganic ions were similar among the samples. Moreover, the WSOC and the total and water-soluble elements contents of the filter and Nano-MOUDI substrates were very consistent. The OC levels of the filter and the BioSampler slurry were also in good agreement. However, the recoverable elements from the BioSampler slurry, determined by SF-ICP-MS, were similar to the water-soluble elements of the filtration and impaction samples but not their total components. Incomplete solubilization of the slurry PM, poor recovery of elements associated with particles greater than 0.2 μm in size, as well as losses of particles during sample storage, freezing, and thawing possibly resulted in the incomplete extraction of elements from the BioSampler slurry. On the other hand, while the redox activity of the water extracts of the filter and Nano-MOUDI PM samples was comparable, that of the BioSampler slurry was substantially higher. However, filtering of the BioSampler slurry yielded comparable levels in the ROS activity among the three suspensions, indicating that insoluble PM species contribute to the overall redox activity of PM2.5.
Finally, findings of this study suggest that the contribution of insoluble particles to the PM redox activity should be considered in the evaluation of the overall PM-induced health impacts. Moreover, PM sampling by the BioSampler, which circumvents the need for particle extraction, constitutes a viable alternative for collecting concentrated particles for characterization of the chemical and oxidative properties of PM. This relatively novel sampling technique will aid in developing wider approaches for chemical and oxidative measurements. Further research aimed at collecting high-time resolution PM slurry samples for cellular oxidative assays and online chemical speciation is currently under way.
Acknowledgments
This research was funded by U.S. EPA under the STAR program through Grant RD-8324-1301-0 to University of Southern California and by the United States Public Health service grants (NIH), R01 ES012053 and R01 ES013432. The research described herein has not been subjected to the agency's required peer and policy review and therefore does not necessarily reflect the views of the agency, and official endorsement should not be inferred. The authors gratefully acknowledge the support of the University of Southern California Provost's Ph.D. fellowship. The authors would also like to thank Debra Schmitz and Emma Di Stefano for performing the DTT and DHBA assays.
REFERENCES
- Ayres , J. G. , Borm , P. , Cassee , F. R. , Castranova , V. , Donaldson , K. , Ghio , A. , Harrison , R. M. , Hider , R. , Kelly , F. , Kooter , I. M. , Marano , F. , Maynard , R. L. , Mudway , I. , Nel , A. , Sioutas , C. , Smith , S. , Baeza-Squiban , A. , Cho , A. , Duggan , S. and Froines , J. 2008 . Evaluating the Toxicity of Airborne Particulate Matter and Nanoparticles by Measuring Oxidative Stress Potential—A Workshop Report and Consensus Statement . Inhal. Toxicol , 20 : 75 – 99 .
- Baulig , A. , Garlatti , M. , Bonvallot , V. , Marchand , A. , Barouki , R. , Marano , F. and Baeza-Squiban , A. 2003 . Involvement of Reactive Oxygen Species in the Metabolic Pathways Triggered by Diesel Exhaust Particles in Human Airway Epithelial Cells . Am. J. Physiol. , 285 : L671 – L679 .
- Becker , S. , Soukup , J. M. and Gallagher , J. E. 2002 . Differential Particulate Air Pollution Induced Oxidant Stress in Human Granulocytes, Monocytes and Alveolar Macrophages . Toxicol. In Vitro , 16 : 209 – 218 .
- Birch , M. E. and Cary , R. A. 1996 . Elemental Carbon-Based Method for Monitoring Occupational Exposures to Particulate Diesel Exhaust . Aerosol Sci. Technol. , 25 : 221 – 241 .
- Brunmark , A. and Cadenas , E. 1989 . Redox and Addition Chemistry of Quinoid Compounds and Its Biological Implications . Free Radic. Biol. Med. , 7 : 435 – 477 .
- Chang , M. C. , Sioutas , C. , Kim , S. , Gong , H. and Linn , W. S. 2000 . Reduction of Nitrate Losses from Filter and Impactor Samplers by Means of Concentration Enrichment . Atmos. Environ. , 34 : 85 – 98 .
- Cheng , Y.-H. and Tsai , C.-J. 1997 . Evaporation Loss of Ammonium Nitrate Particles During Filter Sampling . J. Aerosol Sci. , 28 : 1553 – 1567 .
- Cho , A. K. , Sioutas , C. , Miguel , A. H. , Kumagai , Y. , Schmitz , D. A. , Singh , M. , Eiguren-Fernandez , A. and Froines , J. R. 2005 . Redox Activity of Airborne Particulate Matter at Different Sites in the Los Angeles Basin . Environ. Res , 99 : 40 – 47 .
- Chung , M. Y. , Lazaro , R. A. , Lim , D. , Jackson , J. , Lyon , J. , Rendulic , D. and Hasson , A. S. 2006 . Aerosol-Borne Quinones and Reactive Oxygen Species Generation by Particulate Matter Extracts . Environ. Sci. Technol , 40 : 4880 – 4886 .
- Coudray , C. and Favier , A. 2000 . Determination of Salicylate Hydroxylation Products as an In Vivo Oxidative Stress Marker . Free Radic. Biol. Med. , 29 : 1064 – 1070 .
- Delfino , R. J. , Sioutas , C. and Malik , S. 2005 . Potential Role of Ultrafine Particles in Associations Between Airborne Particle Mass and Cardiovascular Health . Environ. Health Perspect. , 113 : 934 – 946 .
- Dick , C. A. J. , Stone , V. , Brown , D. M. , Watt , M. , Cherrie , J. W. , Howarth , S. , Seaton , A. and Donaldson , K. 2000 . Toxic and Inflammatory Effects of Filters Frequently Used for the Collection of Airborne Particulate Matter . Atmos. Environ. , 34 : 2587 – 2592 .
- DiStefano , E. , Fernandez , A. E. , Delphino , R. J. , Sioutas , C. , Froines , J. R. and Cho , A. K. 2009 . Determination of Metal-Based Hydroxyl Radical Generating Capacity of Ambient and Diesel Exhaust Particles . Inhal. Toxicol. , 21 : 731 – 738 .
- Donaldson , K. , Stone , V. , Borm , P. J. A. , Jimenez , L. A. , Gilmour , P. S. , Schins , R. P. F. , Knaapen , A. M. , Rahman , I. , Faux , S. P. , Brown , D. M. and MacNee , W. 2003 . Oxidative Stress and Calcium Signaling in the Adverse Effects of Environmental Particles (PM10) . Free Radic. Biol. Med. , 34 : 1369 – 1382 .
- Eatough , D. J. , Long , R. W. , Modey , W. K. and Eatough , N. L. 2003 . Semi-Volatile Secondary Organic Aerosol in Urban Atmospheres: Meeting a Measurement Challenge . Atmos. Environ. , 37 : 1277 – 1292 .
- Goldsmith , C. A. , Imrich , A. , Danaee , H. , Ning , Y. Y. and Kobzik , L. 1998 . Analysis of Air Pollution Particulate-Mediated Oxidant Stress in Alveolar Macrophages . J. Toxicol. Environ. Health A , 54 : 529 – 545 .
- Gottipolu , R. R. , Landa , E. R. , Schladweiler , M. C. , McGee , J. K. , Ledbetter , A. D. , Richards , J. H. , Wallenborn , G. J. and Kodavanti , U. P. 2008 . Cardiopulmonary Responses of Intratracheally Instilled Tire Particles and Constituent Metal Components . Inhal. Toxicol , 20 : 473 – 484 .
- Hering , S. , Eldering , A. and Seinfeld , J. H. 1997 . Bimodal Character of Accumulation Mode Aerosol Mass Distributions in Southern California . Atmos. Environ. , 31 : 1 – 11 .
- Hetland , R. B. , Cassee , F. R. , Refsnes , M. , Schwarze , P. E. , Låg , M. , Boere , A. J. F. and Dybing , E. 2004 . Release of Inflammatory Cytokines, Cell Toxicity and Apoptosis in Epithelial Lung Cells After Exposure to Ambient Air Particles of Different Size Fractions . Toxicol. In Vitro , 18 : 203 – 212 .
- Hu , S. , Polidori , A. , Arhami , M. , Shafer , M. M. , Schauer , J. J. , Cho , A. and Sioutas , C. 2008 . Redox Activity and Chemical Speciation of Size Fractioned PM in the Communities of the Los Angeles-Long Beach Harbor . Atmos. Chem. Phys , 8 ( 21 ) : 6439 – 6451 .
- Jalava , P. I. , Hirvonen , M.-R. , Sillanpää , M. , Pennanen , A. S. , Happo , M. S. , Hillamo , R. , Cassee , F. R. , Gerlofs-Nijland , M. , Borm , P. J. A. , Schins , R. P. F. , Janssen , N. A. H. and Salonen , R. O. 2009 . Associations of Urban Air Particulate Composition with Inflammatory and Cytotoxic Responses in RAW 246.7 Cell Line . Inhal. Toxicol , 21 : 994 – 1006 .
- Kachur , A. V. , Held , K. D. , Koch , C. J. and Biaglow , J. E. 1997 . Mechanism of Production of Hydroxyl Radicals in the Copper-Catalyzed Oxidation of Dithiothreitol . Radiat. Res. , 147 : 409 – 415 .
- Khlystov , A. , Zhang , Q. , Jimenez , J. L. , Stanier , C. , Pandis , S. N. , Canagaratna , M. R. , Fine , P. , Misra , C. and Sioutas , C. 2005 . In Situ Concentration of Semi-Volatile Aerosol Using Water-Condensation Technology . J. Aerosol Sci. , 36 : 866 – 880 .
- Kim , S. , Jaques , P. A. , Chang , M. , Barone , T. , Xiong , C. , Friedlander , S. K. and Sioutas , C. 2001a . Versatile Aerosol Concentration Enrichment System (VACES) for Simultaneous In Vivo and In Vitro Evaluation of Toxic Effects of Ultrafine, Fine and Coarse Ambient Particles. Part II: Field Evaluation . J. Aerosol Sci. , 32 : 1299 – 1314 .
- Kim , S. , Jaques , P. A. , Chang , M. , Froines , J. R. and Sioutas , C. 2001b . Versatile Aerosol Concentration Enrichment System (VACES) for Simultaneous In Vivo and In Vitro Evaluation of Toxic Effects of Ultrafine, Fine and Coarse Ambient Particles. Part I: Development and Laboratory Characterization . J. Aerosol Sci. , 32 : 1281 – 1297 .
- Kumagai , Y. , Koide , S. , Taguchi , K. , Endo , A. , Nakai , Y. , Yoshikawa , T. and Shimojo , N. 2002 . Oxidation of Proximal Protein Sulfhydryls by Phenanthraquinone: A Component of Diesel Exhaust Particles . Chem. Res. Toxicol , 15 : 483 – 489 .
- Landreman , A. P. , Shafer , M. M. , Hemming , J. C. , Hannigan , M. P. and Schauer , J. J. 2008 . A Macrophage-Based Method for the Assessment of the Reactive Oxygen Species (ROS) Activity of Atmospheric Particulate Matter (PM) and Application to Routine (Daily-24 h) Aerosol Monitoring Studies . Aerosol Sci. Technol , 42 : 946 – 957 .
- Lawson , D. R. 1990 . The Southern California Air Quality Study . J. Air Waste Manag. Assoc. , 40 : 156 – 165 .
- Li , N. , Kim , S. , Wang , M. , Froines , J. , Sioutas , C. and Nel , A. 2002 . Use of a Stratified Oxidative Stress Model to Study the Biological Effects of Ambient Concentrated and Diesel Exhaust Particulate Matter . Inhal. Toxicol , 14 : 459 – 486 .
- Liu , J. , Steinberg , S. M. and Johnson , B. J. 2003 . A High Performance Liquid Chromatography Method for Determination of Gas-Phase Hydrogen Peroxide in Ambient Air Using Fenton's Chemistry . Chemosphere , 52 : 815 – 823 .
- Misra , C. , Fine , P. M. , Singh , M. and Sioutas , C. 2004 . Development and Evaluation of a Compact Facility for Exposing Humans to Concentrated Ambient Ultrafine Particles . Aerosol Sci. Technol. , 38 : 27 – 35 .
- Netto , L. E. and Stadtman , E. R. 1996 . The Iron-Catalyzed Oxidation of Dithiothreitol Is a Biphasic Process: Hydrogen Peroxide Is Involved in the Initiation of a Free Radical Chain of Reactions . Arch. Biochem. Biophys. , 333 : 233 – 242 .
- Ning , Z. , Geller , M. D. , Moore , K. F. , Sheesley , R. , Schauer , J. J. and Sioutas , C. 2007 . Daily Variation in Chemical Characteristics of Urban Ultrafine Aerosols and Inference of Their Sources . Environ. Sci. Technol. , 41 : 6000 – 6006 .
- O’Brien , P. J. 1991 . Molecular Mechanisms of Quinone Cytotoxicity . Chem.-Biol. Interact , 80 : 1 – 41 .
- Pope , C. A. and Dockery , D. W. 2006 . Health Effects of Fine Particulate Air Pollution: Lines That Connect . J. Air Waste Manag. Assoc. , 56 : 709 – 742 .
- Prahalad , A. K. , Soukup , J. M. , Inmon , J. , Willis , R. , Ghio , A. J. , Becker , S. and Gallagher , J. E. 1999 . Ambient Air Particles: Effects on Cellular Oxidant Radical Generation in Relation to Particulate Elemental Chemistry . Toxicol. Appl. Pharmcol , 158 : 81 – 91 .
- Schafer , M. , Schafer , C. , Ewald , N. , Piper , H. M. and Noll , T. 2003 . Role of Redox Signaling in the Autonomous Proliferative Response of Endothelial Cells to Hypoxia . Circ. Res. , 92 : 1010 – 1015 .
- Squadrito , G. L. , Cueto , R. , Dellinger , B. and Pryor , W. A. 2001 . Quinoid Redox Cycling as a Mechanism for Sustained Free Radical Generation by Inhaled Airborne Particulate Matter . Free Radic. Biol. Med , 31 : 1132 – 1138 .
- Stone , E. A. , Hedman , C. J. , Sheesley , R. J. , Shafer , M. M. and Schauer , J. J. 2009 . Investigating the Chemical Nature of Humic-Like Substances (HULIS) in North American Atmospheric Aerosols by Liquid Chromatography Tandem Mass Spectrometry . Atmos. Environ. , 43 : 4205 – 4213 .
- Tsapakis , M. and Stephanou , E. G. 2003 . Collection of Gas and Particle Semi-Volatile Organic Compounds: Use of an Oxidant Denuder to Minimize Polycyclic Aromatic Hydrocarbons Degradation During High-Volume Air Sampling . Atmos. Environ , 37 : 4935 – 4944 .
- Turpin , B. J. , Huntzicker , J. J. and Hering , S. V. 1994 . Investigation of Organic Aerosol Sampling Artifacts in the Los Angeles Basin . Atmos. Environ. , 28 : 3061 – 3071 .
- Vedal , S. 1997 . Ambient Particles and Health: Lines That Divide . J. Air Waste Manag. Assoc. , 47 : 551 – 581 .
- Verma , V. , Ning , Z. , Cho , A. K. , Schauer , J. J. , Shafer , M. M. and Sioutas , C. 2009a . Redox Activity of Urban Quasi-Ultrafine Particles from Primary and Secondary Sources . Atmos. Environ. , 43 : 6360 – 6368 .
- Verma , V. , Polidori , A. , Schauer , J. J. , Shafer , M. M. , Cassee , F. R. and Sioutas , C. 2009b . Physicochemical and Toxicological Profiles of Particulate Matter in Los Angeles During the October 2007 Southern California Wildfires . Environ. Sci. Technol. , 43 : 954 – 960 .
- Xia , T. , Kovochich , M. and Nel , A. 2006 . The Role of Reactive Oxygen Species and Oxidative Stress in Mediating Particulate Matter Injury . Clin. Occup. Environ. Med. , 5 : 817 – 836 .
- Zhang , Y. , Schauer , J. J. , Shafer , M. M. , Hannigan , M. P. and Dutton , S. J. 2008 . Source Apportionment of In Vitro Reactive Oxygen Species Bioassay Activity from Atmospheric Particulate Matter . Environ. Sci. Technol , 42 : 7502 – 7509 .
- Zhao , Y. , Bein , K. J. , Wexler , A. S. , Misra , C. , Fine , P. M. and Sioutas , C. 2005 . Field Evaluation of the Versatile Aerosol Concentration Enrichment System (VACES) Particle Concentrator Coupled to the Rapid Single-Particle Mass Spectrometer (RSMS-3) . J. Geophys. Res.-Atmospheres , 110 ( D7 ) Art. No. D07S02