Abstract
Poor air hygiene as a result of bioaerosol contamination has caused diverse forms of adverse health effects and diseases. In addition, global biosecurity is threatened by purposeful use of biowarfare agents and the vulnerability of people to the infectious agents. Accordingly, developments in high-volume biosampling, including aerosol-to-hydrosol techniques with low cut-off size, real-time bioaerosol detection, adequate biological quantification, and exposure control, as well as the investigation of the link between disease outcome and bioaerosol exposure, are current areas of bioaerosol research. Although milestone progress has been achieved both in bioaerosol sampling and analysis techniques since late 1800s, compared to atmospheric chemistry the bioaerosol field is still understudied. This is partially because of the lack of both bioaerosol scientists and multidisciplinary collaboration. It is becoming necessary to develop a pool of scientists with different expertise, e.g., bioaerosol scientists, environmental engineers, biomedical engineers, epidemiologists, microbiologists, chemists, physicists, as well as researchers in other engineering fields, in mitigating bioaerosol-related adverse health effects, eliminating diseases, and preventing and controlling epidemic outbreaks. This work is conducted to broadly review current state-of-the-art sciences and technologies in the bioaerosol field. In tackling the challenges ahead, the review also provides perspectives for bioaerosol research needs, and further reminds bioaerosol scientists of those existing technologies in other fields that can be leveraged. In view of the past, forward-looking hypotheses and revolutional perspectives are needed to be formed in order to allow the bioaerosol research have major impacts in the academic community in this new millennium.
1. OVERVIEW
Numerous studies have shown that the inhalation of biological aerosols (bioaerosols) has caused many adverse health effects, even severe casualties when pathogenic microbial species are involved (Robbins et al. Citation2000; Douwes et al. Citation2003; Fung and Hughson Citation2003). Bioaerosols are generally referred to the particles of biological origins suspended in the gaseous medium with an aerodynamic diameter of up to 100 μm, including viruses, bacteria, fungi, pollen, plant debris, and their derivatives such as endotoxins, glucans, allergens, and mycotoxins (Cox and Wathes Citation1995). Humans and animals, when sneezing, can generate a large amount of bioaerosols. Indoor biaoerosols could comprise respiratory pathogens, dust mite fragments, fungal spores, hyphae, and other biological materials. Residential environments may expose more risks to humans through allergy and infection due to the indoor bioaerosol contamination. Outdoor processes, such as waste recycling, biosolid land application, composting, agriculture, pharmaceutical, and biotech activities, could also generate diverse forms of bioaerosols. Although some studies have suggested that early childhood exposure to endotoxin results in a protective effect for developments of eczema and asthma (Gehring et al. 2001; Tischer et al. Citation2011), bioaerosols typically can cause respiratory distress, microbial infection, allergenic reaction, respiratory sensitization, and possible toxicological reactions. For example, in the United States alone, there were 250 million episodes of respiratory infection, i.e., 75 million physician visits per year, 150 million days lost from work with medical care costs of around $10 billion, plus loss of an income of around $10 billion (Cox and Wathes Citation1995). A thorough review on bioaerosol health effects and exposure assessment was conducted, and pointed out that more research is needed to establish better exposure assessment tools and validate newly developed methods (Douwes et al. Citation2003). The severe acute respiratory syndrome (SARS) outbreak in 2003 and global H1N1 viral infection in 2009 prompted worldwide attention. In the meantime, threat of bioterrorism is also increasing because of the rising regional instability and political conflicts. The intentional release of biowarfare agents presents another possible source of infections, greatly endangering the public health. Besides direct health effects, bioaerosols also impact public health and ecology through their global transport and acting as ice nuclei (IN) and cloud condensation nuclei (CCN). Humans are facing increased threats of biological aerosol infections.
Following its golden age in the 18th century, the bioaerosol research is again getting its new era of rapid developments in this millennium. Early observation of airborne microorganisms was traced back to 1833 when mold spores were found on the dusts collected from the air by Charles Darwin on the Cape Verde Islands. Pasteur Citation(1861) published his paper titled, Memoir on the organized bodies which exist in the atmosphere, which documented that there were airborne organisms, invisible to the naked eye, disproving the theory of spontaneous generation. Haldane and Anderson Citation(1887) published a paper regarded as groundbreaking with respect to airborne mold and bacteria in British schools and public housing. They noted the influence of ventilation as well as diurnal differences in airborne bacteria and mold. In 1900, the influence of temperature of liquid air cooled at −182–−190°C on bacteria was investigated (Macfadyen Citation1899). After that successive reports on the germination of Penicillium spores in humid air (Lesage Citation1901), discovery of Bacillus thuringiensis in the air (Uffenheimer Citation1902), and airborne smallpox transmission in the hospital (Wells Citation1905) appeared. The study about the interaction between bacteria and ozonized air was also conducted in 1904 (Rietsch Citation1904). In 1908, a new method for enumerating airborne bacteria by an impinger-like sampler was first reported in Science journal (Winslow Citation1908).
Subsequently, a large number of studies were carried out to investigate the airborne microbial agents (Keegan Citation1918; Tolman et al. Citation1919; Greenburg and Smith Citation1922; Rooks Citation1948; Rosenstern Citation1948; Cown et al. Citation1957). These studies used various samplers, including an all-glass impinger (Greenburg and Smith Citation1922), a device utilizing the electrical force for collecting bacteria and fungus (Rooks Citation1948), and a critical-orifice liquid Impinger (Cown et al. Citation1957). A key moment for the bioaerosol research came in 1933 when fungal spores, algae, and diatoms were found in the air samples collected during the flight from Maine to Denmark by the US Department of Agriculture. In 1958, the six-stage Andersen sampler was invented (Andersen Citation1958), and thereafter a series of modification and evaluation studies of the sampler were carried out (May Citation1964; Flesch et al. Citation1967; Solomon Citation1970; Jones et al. Citation1985). In 1964, two bioaerosol samplers were recommended during the international symposium on the bioaerosol research (Brachman et al. Citation1964): an all-glass impinger (Wolf et al. Citation1959) and an Andersen sampler (Andersen Citation1958). However, during this time period (1900s and 1980s) agar culturing was mainly applied to enumerating airborne biological agents in most studies. In 1988, polymerase chain reaction (PCR), a major innovation in microbiology field, emerged (Saik et al. Citation1988). PCR, also known as molecular cloning of cell-free system, or a gene amplification technology, can allow a very small amount of target DNA to be specifically amplified by million times, thus substantially enhancing the detection of DNA molecules. The emergence of the PCR technology greatly advances the bioaerosol field as reviewed by Peccia and Hernandez Citation(2006).
During the last two decades, many special efforts had been made to promote the awareness of the bioaerosol field to the academic community. There were three special issues on bioaerosol research published by the Journal of Aerosol Science. The first special issue appeared in 1994, which was edited by Jim Ho and David Griffiths, drawing the attention of international community to the bioaerosol science. The second special issue followed in 1997, edited by late John Lacey with a theme of sampling and rapid assay of bioaerosols. In 2005, the third special issue on bioaerosols was organized, which was edited by Sergey A. Grinshpun and James M. Clark, in the areas of developing advanced bioaerosol measurement and characterization methods. In 1999, the Journal of Aerosol Science and Technology also organized a special issue, edited by Janet M. Macher, on bioaerosol sampling and collection. In 2008, Clean Soil Air Water journal organized a special issue on bioaerosol research edited by Sergey A. Grinshpun and Igor E. Agranovski with an aim of showcasing recent bioaerosol research advances. During the International Aerosol Conference (2010) held in Helsinki, Finland, a total of five bioaerosol-related sessions/symposia were organized. In the meantime, a number of books and chapters were produced on bioaerosols (Burge Citation1995; Cox and Wathes Citation1995; Baron and Willeke Citation2001; Agranovski Citation2010; Reponen et al. Citation2011). These academic activities facilitated a rapid progress in the field of bioaerosol.
Starting from the 19th century, as discussed the bioaerosol field (known as “Atmospheric Micrography” during 1861–1882) has undergone significant progress; however, compared to other traditional disciplines, such as atmospheric chemistry, the field is still under-studied due to lack of funding, resources, and bioaerosol scientists as well as inadequate multidisciplinary collaboration. In an article published in 1999, it was argued that the aerobiology has reached a “plateau” in its scientific development, and productive pathways should be explored for the field to have impact in the new millennium (Paul and Isard Citation1999). However, recent episodes of H1N1 infections, SARS outbreak, anthrax attacks after World Trade Center disaster, and potential danger of bird flu pandemic prompted great attention and triggered a burst of federal funds worldwide; accordingly, a noted progress has been made in the field. Despite the achievements, there is still a long and bumpy road ahead in the field of bioaerosol. For example, adequate information about human exposure to biological aerosols, bioaerosol diversity, dose–response relationship, and its relevant health effects is still lacking. Traditional culturing techniques might be used for semi-qualitative biological analysis or describing biological collection efficiencies of sampling tools, but not adequate for detection of non-culturable bioaerosols. Bioaerosol scientists were unaware or did not utilize otherwise readily available technologies such as qPCR, PCR, reverse transcriptase PCR (RT-PCR), and other biological quantification methods such as enzyme-linked immunosorbent assay (ELISA) for allergens, Limulus Amebocyte Lysate (LAL), and Glucatell for endotoxin and glucan measurements. As a result of rapid developments of environmental biotechnologies, analysis for bioaerosol exposure is made relatively easier. However, high volume robust biosampling, generally the first step for such a task, remains an obstacle in the field of bioaerosol. Real-time bioaerosol detection, a long standing challenge, with a response time of less than 1 min requires many fields of expertise to achieve. Accordingly, it is becoming necessary to develop a pool of scientists with different expertise, yet common interests, in bioaerosol science, e.g., aerosol scientists, environmental engineers, biomedical engineers, epidemiologists, microbiologists, chemists, physicists, as well as researchers in other engineering fields in mitigating bioaerosol-related adverse health effects, eliminating diseases, and preventing epidemic outbreaks.
This work intends to broadly review current state-of-the-art technologies and advances in bioaerosol sampling, quantification, detection, exposure monitoring, and control. In tackling the challenges ahead, this review provides perspectives for bioaerosol research trends, and analyzes the research needs for multidisciplinary collaboration.
2. BIOAEROSOL GENERATION AND SAMPLING
2.1. Bioaerosol Generation
When evaluating a new bioaerosol sampler or a control technology, the microbial species are often first aerosolized in the laboratory chamber. Traditionally, a nebulizer is used to aerosolize microorganisms from their liquid suspensions. However, such a method causes damages to the culturability of microorganisms that were repeatedly being aerosolized. In some studies, a new “gentle aerosolizer” named as “liquid sparging aerosolizer (LSA)” was investigated (Ulevicius et al. Citation1997; Mainelis et al. Citation2005). LSA utilizes a peristaltic pump to deliver microbial liquids from a reservoir; therefore, microorganisms only undergo a one-time aerosolization process, thus sustaining limited amount of injury. The results suggested that LSA could produce stable aerosol/bioaerosol concentrations over a longer period (Ulevicius et al. Citation1997; Mainelis et al. Citation2005). There is a commercial version of this device called “sparging liquid aerosol generator” from CH Technologies, Inc (Westwood NJ, USA). When the device is used for the generation of aerosol, a peristaltic pump is required, which might have added a layer of instrumentation barrier for its wider applications compared to nebulizer.
For fungal spore aerosol generation, dry method from fungi-contaminated surfaces without the use of liquid suspension was also utilized widely (Willeke et al. Citation1996; Górny et al. Citation2001). In a laboratory test it was shown that the method of dry generation from agar surface produces non-aggregated fungal spore aerosols compared to a liquid-based method (Willeke et al. Citation1996). It was shown that fungal species, air velocity above the surface, texture of the surface, and vibration of the contaminated material would affect the fungal spore release (Górny et al. Citation2001). Use of the dry generation method results in fewer effects from the growth medium, and proves to be reproducible and easy to control, as well as simple and inexpensive (Kanaani et al. Citation2008).
In recent years, electrospray is increasingly being applied for generating bioaerosols (Kim et al. Citation2008; Eninger et al. Citation2009; Jung et al. Citation2009, 2010). Electrospray is a method that uses high electric voltages between a nozzle and a ground plate to produce highly charged fine aerosol particles. Compared to the traditional collision nebulization method, the electrospray was shown to significantly reduce the bacterial agglomerates due to same particle charge polarity rendered when used as an aerosol generator (Jung et al. Citation2010). It was also indicated that electrospray together with a charge neutralizer produced more stable and viable bacteriophage aerosol particles compared to the conventional nebulization method (Eninger et al. Citation2009). However, the bioaerosols electrosprayed could carry a charge level of ∼106 elementary units (Kim et al. Citation2008). Such high level of particle charges if not neutralized would have negative impacts on bioaerosol sampling and result in human lung deposition. It was shown that the deposition of 1-μm particles carrying 100 elementary electric charges in the lung was about 10 times greater than that of electrically neutral particles (Bailey 1997). These highly charged particles can pose inhalation risks to lab personnel when adequate protection equipments or measures are not available. These limitations could impact or compromise the wide laboratory applications of the electrospray aerosol generation method. Nonetheless, the electrospray method is getting increased momentum in bioaerosol generation due to its stable output of bioaerosols with a monodisperse size.
2.2. Bioaerosol Sampling
In general, impaction, impingement, and filtration methods are widely used for bioaerosol sampling. These traditional sampling methods are reviewed in a recent book (Reponen et al. Citation2011). This paper focuses on aerosol-to-hydrosol samplers that can be used in conjunction with the modern analytical methods. In order to utilize the available molecular techniques or biochemistry methods for adequate bioaerosol sample analysis, an important part of sampling is the ability of the technique to efficiently transfer aerosols into hydrosols (waterborne particles). Liquid impinger has shown this capability, but there exist sampling inlet losses for larger fungal species and low sampling flow rate. A typical example of impingers commonly used is the BioSampler (SKC) with a flow rate of 12.5 L/min, an improvement to the AGI-30 impinger by minimizing particle bounce and particle reaerosolization (Willeke et al. Citation1998). The BioSampler was designed to mimic human nose sampling by drawing air at the above-described flow rate. The BioSampler can be a choice for biological exposure assessment when combined with microbiological analytical techniques such as ELISA and qPCR. In order to facilitate rapid response to the microbial aerosol contamination, high volume robust aerosol-to-hydrosol sampling is needed. Among other requirements, high flow rate and portability are two critical considerations for the bioaerosol sampling. In general, high volume sampling comes with higher power consumption and difficulty of installation. Sampler portability, on the other hand, demands high efficiency of the battery.
Among other aerosol-to-hydrosol concentrators, the BioGuardian air sampler (InnovaTek, Inc., Richland, WA, USA) operates from 100 to 1000 L/min and collects air sample into 10–15 mL of liquid. The SpinCon air sampler by Specter Industries, Inc. (Kansas City, MO, USA) samples air at a flow rate of 450 L/min and concentrates the air sample into 10 mL of liquid. Another sampler, the BioCapture 650 (MesoSystems Technology, Inc., Albuquerque, NM, USA), achieves a sampling flow rate of 200 L/min and collects particles into 5 mL of liquid. Recently, Lawrence Livermore National Laboratory (LLNL) has introduced a compact (combined dimensions 2.5 L) aerosol collector, which operates at a flow rate of 225 L/min and transfers aerosols from a large volume of air into small amounts of liquids down to 1 mL (Mainelis et al. Citation2002b). This equipment has demonstrated higher aerosol concentration rate in terms of sampling flow rate and volume of used collection liquid. For aerosol-to-hydrosol sampling, a wetted wall bioaerosol sampling cyclone was developed with a sampling flow rate of up to 1250 L/min and a continuous liquid outflow rate of about 1 mL/min through upgrading an existing system (King et al. Citation2009; Mcfarland et al. Citation2010). Besides, single-stage and multi-stage virtual impactors were also developed for concentrating biological aerosols (Bergman et al. Citation2005; Park et al. Citation2009). These types of aerosol-to-hydrosol samplers can be adapted to be automated with advanced bioaerosol detection techniques via a micro-fluid channel as illustrated in .
For microenvironments, some personal bioaerosol samplers were also developed or tested for biological exposure monitoring (Agranovski et al. 2004a; Görner et al. 2006; Haatainen et al. Citation2010). Agranovski and co-workers (2002) have developed a personal bioaerosol sampler by utilizing a porous medium submerged in a liquid layer, and the results indicated that by plate spreading the sampler had detection limits of 15 bacterial CFU/m3 and fungal concentrations of above 5 CFU/m3 when using a sampling time of 8 h. Görner and co-workers (2006) have developed a personal rotating cup sampler in which three particle size selectors allow health-related aerosol fractions to be sampled into liquids according to international inhalation conventions.
As another alternative for collecting bioaerosols, electrostatic precipitation is increasingly being investigated due to their electrical charges (Mainelis et al. Citation1999, 2011; Mainelis et al. Citation2002a, 2002b, 2002c; Lee et al. 2004; Yao and Mainelis Citation2006; Han and Mainelis Citation2008; Sillanpä et al. Citation2008; Tan et al. Citation2011). One study demonstrated that the electrostatic sampler can achieve about 5–9 times higher culturable bacteria or fungi concentrations than the BioStage impactor under certain conditions (Yao and Mainelis Citation2006). The electrostatic technique has been shown to be able to directly transfer aerosols into hydrosols (Yao et al. 2009b; Han et al. Citation2010; Tan et al. Citation2011). It was shown that the electrostatic sampling performs better than the BioSampler when sampling airborne allergens and toxins at low sampling flow rates and high electrostatic fields (Yao et al. 2009). In general, the electrostatic sampling requires much less power consumption compared to impaction-type samplers; and its physical collection efficiency depends on the electrostatic field strength, the sampling flow rate, and the electrical charges carried by microbial species. The collection efficiency of the electrostatic field has been shown to be up to 90% for sampling particles of 0.3–0.5 μm under certain conditions (Yao and Mainelis Citation2006). Such a sampling technique could be combined with molecular techniques for better biological exposure assessment and monitoring.
Recently, a new type of electrostatic sampler with a half-pipe shape has been designed, and the sampler allows the charged particles to be collected onto a superhydrophobic channel (positive electrode) (Han and Mainelis Citation2008; Han et al. Citation2010). The sampler was successfully tested with airborne bacteria, in which the air samples were collected into a 5-μL liquid suspension (Han et al. Citation2010). This study presents an example of efficient aerosol-to-hydrosol sampling techniques. In another study, a newer model of an automated electrostatic sampler (AES) was developed and tested (Tan et al. Citation2011). The sampler consists of a ball-shape electrode and a circular electrode. Above the circular electrode, a plastic cylindrical reservoir was built with a liquid inlet and a liquid outlet made of copper. The AES sampler allows the bioaerosol particles to be collected into the reservoir with a high aerosol concentration rate and to be continuously delivered to a sensor device via the liquid outlet (Tan et al. Citation2011). The integration of AES sampler with a biosensor device could offer a promising platform for the automated bioaerosol sensing (Tan et al. Citation2011). However, the major disadvantage of the electrostatic sampling is its low collection efficiency at high sampling flow rate. Use of higher electrostatic field can improve the collection efficiency, but if too high, could cause the dielectric breakdown of air.
3. BIOAEROSOL QUANTIFICATION AND DETECTION
3.1. Bioaerosol Exposure Quantification
In recent years, PCR-based technologies are increasingly being used to analyze biological cells in air samples (Angenent et al. Citation2005; Kuske Citation2006; Peccia and Hernandez Citation2006; Oppliger et al. Citation2008; Rinsoz et al. Citation2008; West et al. Citation2008; Chen et al. Citation2009; Fallschissel et al. Citation2009; Evgrafov et al. Citation2010). PCR techniques can amplify a tiny amount of DNA or RNA segments extracted from the environmental samples by polymerase; and the initial concentration of the sample can be determined based on the DNA standards and its cycle threshold of fluorescence level (Ct value). Use of PCR techniques will not only significantly lower the bioaerosol detection limits for air samples but also greatly reduce the sampling time that is needed for sufficient air samples. The detection limit of qPCR has been shown varying with studies from 50 copies (Ide et al. Citation2003) to 100 genomic copies per milliliter of liquid (Peccia and Hernandez Citation2006). A recent laboratory study showed that qPCR is capable of detecting bacterial concentration differences in air samples only if the true difference is at least 1.3 to 3.2 times (Hospodsky et al. Citation2010). In another recent investigation, high-density phylogenetic DNA microarray was successfully applied to describing the urban bacterial aerosol diversity (Brodie et al. Citation2007). Unsurprisingly, the PCR technique was also extensively utilized in quantifying fungal spores in air samples (Haugland et al. Citation2002; Meklin et al. Citation2007). Incorporation of PCR techniques with air sampling technique for bioaerosol sample analysis was extensively reviewed elsewhere in an effort to speed up the bioaerosol research (Peccia and Hernandez Citation2006).
Certainly, these microbiological techniques have their own limitations such as need for DNA extraction, interference of environmental matrix, including inhibition or amplification, longer detection time, and extensive labor. In addition, microbial fragments and animal allergens cannot be analyzed using qPCR, and the technology does not yield information on the viability, which is, however, especially important for infectious agents. Apart from these limitations, the contamination of DNA sample during the preparation is another problem with the techniques. Nonetheless, use of the PCR-based techniques significantly lower the bioaerosol detection limits over the traditional culturing technique, which has set a milestone in the bioaerosol field. Overall, polymerase-based technologies still remain as powerful biological tools in environmental science and engineering.
In addition to the aforementioned technologies, other molecular tools, such as denaturing gradient gel electrophoresis (DGGE) and terminal restriction fragment length polymorphism (T-RFLP), are also increasingly applied to performing post-PCR analysis, e.g., microbial aerosol diversity (Negrin et al. Citation2007; Nehme et al. Citation2008; Georgakopoulos et al. 2009; Lee et al. Citation2009; Li et al. 2010). DGGE is an electrophoresis process to separate DNA segments by applying chemical gradient, and is increasingly used to describe the microbial diversity differences between bioaerosol samples collected using different sampling tools, obtained from different geological locations, and treated by different inactivation methods. T-RFLP is a molecular biology technique for profiling microbial communities according to the position of a restriction site closest to a labeled end of an amplified gene. This method has also been applied to study airborne microbial structures (Lee et al. Citation2010). Use of these tools contributes to a better understanding of the microbial aerosol community.
FIG. 2 Integration of molecular, biochemistry and nanotechnology-based methods for bioaerosol quantification, detection, and exposure assessment.
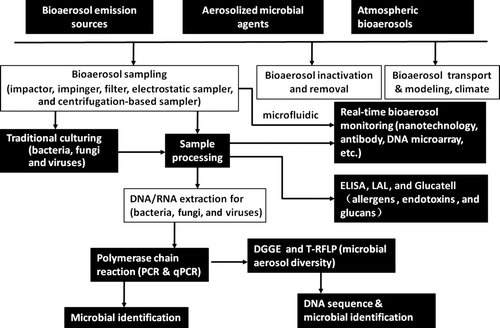
Besides, biochemistry-based assays, such as ELISA and Limulus Amebocyte Lysate (LAL) (Associates of Cape Cod, Inc., East Falmouth, MA, USA), were also applied in the field of bioaerosol (Reponen et al. Citation2011). ELISA is an antibody-based immunoassay, and in general its detection limit can be down to 0.8 ng/mL for most household allergens (Indoor Biotechnologies, Charlottesville, VA, USA). By combining aerosol-to-hydrosol sampling technologies with ELISA, airborne allergens can be described and quantified (Reponen et al. Citation2011). LAL assay, on the other hand, is widely used to measure the endotoxin concentration for biological exposure assessment. LAL is a very sensitive technology and its detection limit is down to 0.5 pg/mL (Associates of Cape Cod, Inc.). (1→3)-β-D-glucan is a fungal cell wall component, which is often used as a surrogate for quantifying fungal biomass as a risk marker for indoor environments (Rylander Citation1999, 2002). Glucatell assay (Associates of Cape Cod, Inc.), a modified LAL, is used to measure (1→3)-β-D-glucan mass in the environments with a detection limit of close to 10 pg/mL (Associates of Cape Cod, Inc.). Use of these techniques can measure nonculturable fractions of airborne biomass in the environments; however, the major problem when using the LAL assay is the sample inhibition or enhancement.
While culturing techniques can be used for a qualitative study, they might fail to detect important microbial species or nonculturable fractions due to their sampling and culturing limitations. This is largely due to the fact that impaction-based collection techniques often have lower collection efficiencies for smaller particles, and a very small percentage (usually less than 1%) of airborne microbial species are culturable. Molecular techniques, such as PCR, qPCR, and RT-PCR, can detect nonculturable cells, thus improving the understanding of airborne microbial community and the estimate of relevant risks. On the other hand, nonculturable biomass, such as endotoxin, glucans, and allergens, cannot be identified or quantified using the culturing method. This represents a significant underestimate for the biological exposure assessment, thus influencing decision-making and control strategies. Use of biochemistry-based methods, such as ELISA and LAL, could help in their detection and analysis. As summarized in , these described tools and techniques together with an advanced bioaerosol sampling can assist in adequate airborne biological exposure assessment for bacteria, fungi, viruses, as well as endotoxins, glucans, and allergens. Thus, the human health risks via inhalation pathway can be well described and evaluated.
3.2. Real-Time Bioaerosol Detection
Real-time detection of bioaerosols is a long-standing challenge in the bioaerosol field. Over the past years, many technologies have been investigated for possible detection of airborne biological agents in a real-time manner. These include bioaerosol mass spectrometry (BAMS), (Fergenson et al. Citation2004; Tobias et al. Citation2005), surface-enhanced Raman spectroscopy (SERS) (a surface sensitive technique that results in the enhancement of Raman scattering by molecules adsorbed on rough metal surfaces) (Sengupta et al. Citation2007), and flow cytometry (FCM) with fluorochrome (a technique for counting and examining microscopic particles, such as cells and chromosomes, by suspending them in a stream of fluid and passing them through an electronic detection apparatus) (Chen and Li Citation2005). Among others, DNA-based, electrical, and nanosensor techniques are emerging as promising platforms for real-time detection of bioaerosols.
Use of mass spectrometry for real-time bioaerosol detection can achieve a rapid analysis of bioaerosols through analyzing the mass-to-charge ratio of biological agents ionized by laser; however, background aerosol particles and analysis of the spectra add difficulties to its overall application. A fluorescent aerodynamic particle sizer (FLAPS) was also described as a front-end optical sensor for “live” particles through measuring fluorescence signals under FCM using ultraviolet (UV) excitation at 340–360 nm (Ho Citation2002). Use of ultraviolet aerodynamic particle sizer (UVAPS, model 3312, TSI Inc., St. Paul, MN, USA), a modified FLAPS, is getting increased attention. The UVAPS/FLAPS was originally developed by Canadian scientists for the real-time detection of microorganisms (Ho et al. Citation1995; Boulet et al. Citation1996). The instrument can yield the total viable bioaerosol concentration in a real-time manner for a particular environment. UVAPS has already been applied to study bioaerosols (Agranovski et al. Citation2003a, 2003b, 2004; Agranovski and Ristovski Citation2005; Huffman et al. Citation2010; Jung et al. Citation2010; Lee et al. Citation2010). However, many problems for UVAPS, such as environmental matrix influence (Huffman et al. Citation2010), particle size dependence (Agranovski et al. Citation2003b; Agranovski and Ristovski Citation2005), and inability of detecting bacterial spores (Agranovski et al. Citation2003b), remain to be investigated.
Although the performance of UVAPS has been compared with culturing and microscope counting techniques (Kanaani et al. Citation2008), it has not been validated with molecular techniques, such as qPCR. A major drawback of UVAPS is its inability to identify species. Nonetheless, it can provide real-time viable bioaerosol concentration profiles, and can serve as a pre-alarm system when the viable bioaerosol concentration substantially exceeds the background level. Apart from UVAPS, there are other commercially available bioaerosol alert equipments, such as the Model 3317 Fluorescence Aerosol Particle Sensor III (FLAPS III)™ (TSI), the IBAC™, and the AirSentinel® (ICx Technologies, Inc., Arlington, VA). These technologies provide bio-threat alerts through assessing the changes in ambient biological particulate levels.
In addition, many other biosensor technologies are available, including DNA microarray (an arrayed series of thousands of microscopic spots of DNA oligonucleotides for hybridization with cDNA or cRNA sample) (Schena et al. Citation1995), immunochemical assay (Mancinia et al. Citation1965), liquid-bead array coupled with PCR (Wilson et al. Citation2005), nanotube and nanowire field effect transistors (these rely on an electric field to control the conductivity of a channel of one type of charge carrier in a semiconductor material) (Patolsky et al. Citation2004; So et al. Citation2005), and other fluorescence-based technologies. However, this work is not designed to carry out an extensive review of biosensors that are mostly focused on liquid or food samples. Instead, this review intends to remind bioaerosol scientists of those available technologies that can be leveraged. In general, an advanced bioaerosol detector generally requires the combination of three features: speed, accuracy, and ease of use (Enserink Citation2002). All of the above-mentioned technologies require efficient bioaerosol sampling (aerosol-to-hydrosol) from environments. For example, the BioSampler was used in combination with a fluorescence spectrometer and a temperature-controlled microwave for online monitoring of anthrax surrogate Bacillus subtilis based on the terbium luminescence assay (Lester and Ponce Citation2002). To the authors’ best knowledge, most of these techniques are in their development stages and have not been fully employed to the field or robustly validated yet. By combining an advanced bioaerosol sampling system, qPCR, PCR, and RT-PCR can significantly lower the detection limits of bioagents in air samples as illustrated in , but it is difficult to automate as an unattended system. In addition, the preparation of DNA sample is labor-intensive and tedious and detection time could be up to several hours. This is far away from the objective of “detect-to-warn” time span, which is generally agreed as 1 min to allow a timely response or rescue in a man-made bioterror event (National Research Council Citation2005).
Traditionally, the bacterial impedance-based technique has been applied to detect and/or quantify food-borne microorganisms (Yang and Bashir Citation2008). In recent years, as a result of its rapid measurement for bacterial concentrations (Suehiro et al. Citation2003; Wu et al. Citation2005; Yang Citation2008), such technique has received attention from researchers in other fields. In some studies, the dielectrophoretic impedance measurement method was combined with antigen–antibody reaction (DeSilva et al. Citation1999; Suehiro et al. Citation2003; Yang et al. Citation2004). In other studies, the electrical impedance measurements were used to detect ionic metabolites produced by bacterial growth (Noble et al. Citation1999; Silley and Mortimer Citation2003). In another study, it was applied to differentiating between dead and live cells (Suehiro et al. Citation2003). Recently, a study has investigated the detection of bacterial cells by making use of impedance properties of bacterial cell suspensions using interdigitated microelectrodes (Yang Citation2008). Use of bacterial impedance together with dielectrophoretic manipulation can also differentiate between different bacillus species through monitoring current change when the species pass through two electrodes (Beck et al. Citation2008). Due to its fast response, electrical-based method holds a broad promise when coupled with an air sampling technique in the real-time monitoring bioaerosols.
With the advent of nanotechnology, label-free (without second antibody compared to immunoanalysis) nanowire sensor has been successfully used to detect biological species in the fluid by translating molecular binding events into microelectronic signals via a field-effect transistor (FET) (Cui et al. Citation2001; Patolsky et al. Citation2004). This creates a critical bridge between physical and biological disciplines. Use of this nanowire-based biosensor would also require technology that is able to efficiently transfer bioaerosols into hydrosols without damaging their biological integrity. As discussed previously, some sampling technologies, such as, electrostatic precipitator and wetted cyclone sampler, can deposit airborne biological agents into small amounts of liquids (Yao et al. 2009; Han et al. Citation2010; McFarland et al. Citation2010; Tan et al. Citation2010). Advances in microfluidic channel, as reviewed by Whitesides Citation(2006), can enable the efficient transport of virus-laden liquids onto the chips that are preoccupied with specific antibody or functional biomolecule-coated FETs constructed using silicon nanowires as illustrated in . Upon the binding of an antibody to the target virus, individual nano-devices would generate a small electronic signal for implying a conductance change of the nanowire (Cui et al. Citation2001; Patolsky et al. Citation2004).
Pre-amplifier and lock-in amplifier can be used in amplifying small conductance change of a nanowire device when the attached antibody binds to a virus. The amplified signal can be further transmitted via wireless technologies as suggested in . The combination of these described technologies holds great promise in continuously monitoring microbial profiles in the air, possibly leading to the innovation of a device that is able to show the levels of classified agents in a real-time manner. Yet, roadblocks still exist ahead. For extreme low concentration of agents, high volume bioaerosol sampling is required, which correspondingly places a significant barrier on the development of a human wearable device. Another challenge is that the lifetime of an antibody-coated nano-device might be short when exposed to environmental conditions, thus limiting its ability of continuous monitoring. Aptamer, a bio-functional molecule, on the other hand, was reported to have higher affinities and longer shelf life than antibodies (National Research Council Citation2005). Thus, the aptamer might be a better alternative for chemically modifying the nanowire device for environmental biosensing. With the increasing risks from both natural and man-made biothreats, a real-time bioaerosol detection system is greatly needed in the coordinated effort to allow immediate countermeasures such as isolation and evacuation put in place swiftly during an emergent situation. The development of such a system would go a long way toward battle against an infectious disease outbreak or a man-made bioattack. Out of other considerations, robust bioaerosol sensing technologies would need to be label-free, single molecule detection, portable, compact, low maintenance, fast, and automatic. There is certainly a long road ahead to achieve such objectives.
4. BIOAEROSOL CONTROL AND INACTIVATION
Over the years, a variety of newer technologies, including thermal treatment, nanomaterial filtration, and microwave irradiation, have been developed in controlling airborne biological agents. Traditionally, the prevention and control of bioaerosol exposure generally have three levels of intervention, namely, elimination at source, controlling the source, and controlling the exposure. For indoor environments, humidity control (below 50%) and careful use of appliances that are susceptible to contamination with microorganisms can prevent amplification and production of bioaerosols (Burge Citation1995). Biofiltration, generally used in air cleaning systems (Kummer Citation2009), was applied at a composting facility, showing more effectiveness for controlling the emissions of fugal species than bacterial species as a result of aerodynamic size difference (Sanchez-Monedero et al. Citation2003).
Among other techniques, X-Ray-enhanced electrostatic field was shown to achieve a removal efficiency of 4 log values when collecting ultrafine viral particles (Hogan et al. 2004); and electrostatic fields have also been attempted in activating airborne and surface-borne microorganisms (Yao et al. Citation2005). In activating bioaerosols, photocatalytic materials, such as Titanium dioxide (TiO2), have been investigated (Pal et al. Citation2007). An inactivation of about 75% of B. subtilis spores was achieved through combining unipolar ion emission and photocatalytic oxidation (Grinshpun et al. 2007b). In another study, when the surrounding air temperature exceeded 350°C or 400°C, 99% of airborne Aspergillus versicolor and Cladosporium cladosporioides, as well as certain bacterial bioaerosols, were shown to lose their culturability in about 0.2 s (Jung et al. Citation2009a, 2009b). Significant damages were observed to the surface morphologies of fungal spores as a result of the treatment (Jung et al. Citation2009a). Thermal inactivation was also shown to effectively inactivate Bacillus subtilis var niger endospores, achieving an efficiency of 99.999% at higher temperature(Grinshpun et al. Citation2010).
Traditional air-conditioning system has limited ability in inactivating collected biological agents. The filter inside the system often fails to collect smaller biological agents, and those collected may still grow and propagate when the humidity level is adequate. In recent years, antimicrobial materials are also incorporated into air filter to inhibit the growth of collected microorganisms (Foarde et al. Citation2000; Cecchini et al. Citation2004; Lee et al. 2008a). The filtration technologies developed using nanoscale materials for water treatments (Brady-Estevez et al. Citation2010; Schoen et al. Citation2010) can certainly be adapted for airborne microbial aerosol control. In a recent study, carbon nanotube-based filters were applied to controlling bioaerosols, and the results indicated that a physical removal efficiency of 95% was obtained for B. subtilis var niger at a carbon nanotube loading of 1.6 μg/cm2 (Guan and Yao Citation2010). Studies indicated that carbon nanotube exhibited strong toxicity toward Escherichia coli (Kang et al. Citation2009; Brady-Estevez et al. Citation2010). In another study, carbon nanotube-made filter was shown to be capable of both collecting and inactivating airborne microbial species (Xu and Yao Citation2011). In addition, particulate air (HEPA)-UV air filters were also investigated in controlling microbial aerosols (Kujundzic et al. Citation2005). For personal exposure protection, respiratory filter masks, such as N95 respirators, and surgical masks are widely adopted (Burton et al. Citation2007; Lee et al. 2008). The bioaerosol filtration efficiencies of these respirators were extensively studied, and it was shown that the N95 filtering face piece respirators may not provide the expected protection level against small virions (Balazy et al. 2006a, 2006b; Eninger et al. Citation2008a, 2008b; Shaffer and Rengasamy Citation2009). In a review article, it was pointed out that the respiratory protection against bioaerosol exposure is dependent on several factors: bioaerosol size and filter characteristics, flow rate, face-fitting characteristics, and efficiency degradation of filter material (Rengasamy et al. Citation2004).
Cold plasma (Birmingham and Hammerstrom 1999), UV light (Peccia and Hernandez Citation2001; Menzies et al. Citation2003; Xu et al. Citation2003, 2005; Kujundzic et al. Citation2005, 2006, 2007; Tseng and Li Citation2005; Ryan et al. Citation2010), and microwave irradiation (Wu and Yao Citation2010; Zhang et al. Citation2010) have also been used in controlling bioaerosols. It was shown that use of UV germicidal lights in office buildings could result in significant reduction of microbial and endotoxin concentrations on irradiated surfaces within the ventilation systems and substantially improve the health conditions of office workers (Menzies et al. Citation2003). Among other factors, humidity levels were shown to play an important role in the microbial inactivation efficacy of UV germicidal lights (Peccia and Hernandez Citation2001; Kujundzic et al. 2007. In a recent study, the results showed that the exposure of Bacillus subtilis var niger to microwave irradiation at 2450 MHz for about 1.5 min can achieve an inactivation of ∼70% (Wu and Yao Citation2010). Scanning electron images and transmission electron images showed visible damages to the exposed cells (Wu and Yao Citation2010). In another study, microwave irradiation coupled with nanofibrous filters was also investigated in inactivating bioaerosols (Zhang et al. Citation2010). It was indicated that power level and exposure time of microwave irradiation play an important role in the microbial inactivation (Wu and Yao Citation2010; Zhang et al. Citation2010). In addition, negative air ions were also shown to effectively inactivate airborne biological agents (Holt et al. Citation1999) and control airborne transmission of newcastle disease virus (Mitchell and King Citation1994). These technologies have already been used or have the potential application in practical environments.
Control of the air stream flow direction, e.g., creating negative pressure inside a room or hospital where infectious patients are being treated, on the other hand, may effectively limit the flow of airborne biological agents to a local microenvironment or the contamination source, thus preventing further spreading of diseases. Minimizing biological exposure in public places, e.g., railway station, subways, airport, is especially important since some of the passengers might have infectious diseases, which may spread through breathing, coughing, talking, and sneezing. These places need effective biological monitoring as well as effective control measures. However, such devices or measures are not in place even in developed countries. In future research endeavors, simple yet environment friendly, high-volume air sterilization method is expected to receive wide attention and recognition.
5. CONCLUDING REMARKS
Poor air hygiene as a result of bioaerosol contamination has caused diverse forms of adverse health effects and diseases. In addition, global biosecurity is threatened by purposeful use of biowarfare agents and the vulnerability of people to infectious diseases. Accordingly, developments in high-volume sampling, including high flow aerosol-to-hydrosol techniques with low cutoff size, real-time bioaerosol detection technology, adequate biological quantification and control system, as well as the investigation of link between disease outcome and bioaerosol exposure, are subject to extensive studies. Although significant progress in the bioaerosol field has been made since late 1800s, compared to other disciplines, e.g., the atmospheric chemistry, the field is still understudied. In face of the increased environmental challenges, bioaerosol scientists need to pair up with their counterparts in other fields to move the science forward while solving real world problems. Currently, the most difficult part of the field is the real-time bioaerosol identification and quantification, and the integration of different technologies might go a long way toward the hurdle. However, broad hypotheses and revolutional perspectives need to be formed in order to allow the bioaerosol research to have major impacts in the academic community in this new millennium. Many topics discussed here merit a separate individual review; however, this work is not intended to provide an exhaustive review on specific technologies in the bioaerosol field, but to compile the achievements and analyze the existing technologies in other fields that can be leveraged by the bioaerosol scientists. In the meantime, for the investigators in other fields this review might also serve as a reference to apply their expertise in bioaerosol fields.
Acknowledgments
This study was funded by the National High Technology Research and Development Program of China (Grant 2008AA062503), National Science Foundation of China (Grants 20877004, 21077005), and the Peking University “100 Scholar Program” fund. This work is also supported by special funds of State Key Joint Laboratory of Environmental Simulation and Pollution Control (10Y04ESPCP, 11Z02ESPCP). The authors would like to thank anonymous reviewers and editor Tiina Reponen for their great comments in improving the manuscript.
REFERENCES
- Agranovski , I. E. 2010 . “ Filtration of Liquid and Solid Aerosols on Liquid-Coated Filters ” . In Aerosols: Science and Technology , 315 – 318 . New York : Wiley-VCH . I. Agranovski
- Agranovski , I. E. , Agranovski , V. , Reponen , T. , Willeke , K. and Grinshpun , S. A. 2002 . Development and Evaluation of a New Personal Sampler for Culturable Airborne Microorganisms . Atmos. Environ. , 36 : 889 – 898 .
- Agranovski , I. E. , Safatov , A. S. , Borodulin , A. I. , Pyankov , O. V. , Petrishchenko , V. A. Sergeev , A. N. 2004a . Inactivation of Viruses in Bubbling Processes Utilized for Personal Bioaerosol Monitoring . Appl. Environ. Microbiol. , 70 : 6963 – 6967 .
- Agranovski , V. and Ristovski , Z. D. 2005 . Real-time Monitoring of Viable Bioaerosols: Capability of the UVAPS to Predict the Amount of Individual Microorganisms in Aerosol Particles . J. Aerosol Sci. , 36 : 665 – 676 .
- Agranovski , V. , Ristovski , Z. D. , Ayoko , G. A. and Morawska , L. 2004b . Performance Evaluation of the UVAPS in Measuring Biological Aerosols: Fluorescence Spectra from NAD(P). H Coenzymes and Riboflavin . Aerosol Sci. Techno. , 38 : 354 – 364 .
- Agranovski , V. , Ristovski , Z. , Blackall , P. J. and Morawska , L. 2004c . Size-Selective Assessment of Airborne Particles in Swine Confinement Building with the UVAPS . Atmos. Environ. , 38 : 3893 – 3901 .
- Agranovski , V. , Ristovski , Z. , Hargreaves , M. , Blackall , P. J. and Morawska , L. 2003b . Performance Evaluation of the UVAPS: Influence of Physiological Age of Airborne Bacteria and Bacterial Stress . J. Aerosol Sci. , 34 : 1711 – 1727 .
- Agranovski , V. , Ristovski , Z. , Hargreaves , M. , Blackall , P. J. and Morawska , L. 2003a . Real-Time Measurement of Bacterial Aerosols with the UVAPS: Performance Evaluation . J. Aerosol Sci. , 34 : 301 – 317 .
- Andersen , A. 1958 . A New Sampler for the Collection, Sizing and Enumeration of Viable Airborne Particles . J. Bacteriol. , 76 : 471 – 484 .
- Angenent , L. T. , Kelley , S. T. , St Amand , A. , Pace , N. R. and Hernandez , M. T. 2005 . Molecular Identification of Potential Pathogens in Water and Air of A Hospital Therapy Pool . Proc. Natl. Acad. Sci. USA , 102 : 4860 – 4865 .
- Bailey , A. 1997 . The Inhalation and Deposition of Charged Particles within the Human Lung . J. Electrostat. , 42 : 25 – 32 .
- Bałazy , A. , Toivola , M. , Adhikari , A. , Sivasubramani , S. K. , Reponen , T. and Grinshpun , S. A. 2006a . Do N95 Respirators Provide 95% Protection Level against Airborne Viruses, and How Adequate Are Surgical Masks? . Am. J. Infect. Control , 34 : 51 – 57 .
- Balazy , A. , Toivola , M. , Reponen , T. , Podgórski , A. , Zimmer , A. and Grinshpun , S. A. 2006b . Manikin-Based Performance Evaluation of N95 Filtering-Facepiece Respirators Challenged with Nanoparticles . Ann. Occup. Hyg. , 50 : 259 – 269 .
- Baron , P. A. and Willeke , K. 2001 . Aerosol Measurement: Principles, Techniques, and Applications , 2nd ed , New York : John Wiley & Sons .
- Beck , J. D. , Shang , L. , Li , B. , Marcus , M. S. and Hamers , R. J. 2008 . Discrimination Between Bacillus Species by Impedance Analysis of Individual Dielectrophoretically Positioned Spores . Anal. Chem. , 80 : 3757 – 3761 .
- Bergman , W. , Shinn , J. , Lochner , R. , Sawyer , S. , Milanovich , F. and Mariella , R. Jr. 2005 . High Air Flow, Low Pressure Drop, Bio-Aerosol Collector Using a Multi-Slit Virtual Impactor . J. Aerosol Sci. , 36 : 619 – 638 .
- Birmingham , J. and Hammerstrom , D. 1999 . Apparatus and Method for Lysing Bacterial Spores to Facilitate Their Identification. U.S. Patent 5989824 , Richland, WA, USA : MesoSystems Technology, Inc. .
- Boulet , C. , Ho , J. , Stadnyk , L. , Thompson , H. , Spence , M. Luoma , G. Defence Research Establishment Suffield, memorandum no. SR 652 (unclassified) . Canadian Defense Documents available at Information Holdings-Operations Department of National Defence . Ottawa, Ont., Canada.
- Brachman , P. S. , Erlich , R. , Eichenwald , H. F. , Cabelli , V. J. , Kethley , T. W. Madein , S. H. 1964 . Standard Sampler for Assay of Airborne Microorganisms . Science , 144 : 1295
- Brady-Estevez , A. S. , Schnoor , M. H. , Kang , S. and Elimelech , M. 2010 . SWNT-MWNT Hybrid Filter Attains High Viral Removal and Bacterial Inactivation . Langmuir , 26 : 19153 – 19158 .
- Brodie , E. L. , DeSantis , T. Z. , Parker , J. P. M. , Zubietta , I. X. , Piceno , Y. M. and Andersen , G. L. 2007 . Urban Aerosols Harbor Diverse and Dynamic Bacterial Populations . Proc. Natl. Acad. Sci. USA , 104 : 299 – 304 .
- Burge , H. A. 1995 . “ Bioaerosols in the Residential Environment ” . In Bioaerosols Handbook , Edited by: Cox , C. and Wathes , C. 579 – 591 . Boca Raton, FL : CRC Press .
- Burton , N. C. , Grinshpun , S. A. and Reponen , T. 2007 . Physical Collection Efficiency of Filter Materials for Bacteria and Viruses . Anns. Occup. Hyg. , 51 : 143 – 151 .
- Cecchini , C. , Verdenelli , M. C. , Orpianesi , C. , Dadea , G. M. and Cresci , A. 2004 . Effects of Antimicrobial Treatment on Fiberglass Acrylic Filters . J. Appl. Microbiol. , 97 : 371 – 377 .
- Chen , P. S. and Li , C. S. 2005 . Bioaerosol Characterization by Flow Cytometry with Fluorochrome . J. Environ. Monit. , 7 : 950 – 959 .
- Chen , P. S. , Lin , C. K. , Tsai , F. T. , Yang , C. Y. , Lee , C. H. Liao , Y. S. 2009 . Quantification of Airborne Influenza and Avian Influenza Virus in a Wet Poultry Market Using a Filter/Real-Time qPCR Method . Aerosol Sci. Technol. , 43 : 290 – 297 .
- Cown , W. B. , Kethley , T. W. and Fincher , E. L. 1957 . The Critical-Orifice Liquid Impingers as A Sampler for Bacterial Aerosols . Appl. Microbiol. , 5 : 119 – 124 .
- Cox , C. S. and Wathes , C. M. 1995 . Bioaerosols Handbook , 618 – 623 . New York : CRC Press .
- Cui , Y. , Wei , Q. , Park , H. and Lieber , C. M. 2001 . Nanowire Nanosensors for Highly Sensitive and Selective Detection of Biological and Chemical Species . Science , 293 : 1289 – 1292 .
- DeSilva , M. S. , Zhang , Y. , Hesketh , P. J. , Maclay , G. J. , Gendel , S. M. and Stetter , J. R. 1999 . Impedance-Based Sensing of the Specific Binding Reaction Between Staphylococcus Enterotoxin-b and Its Antibody on an Ultra-Thin Platinum Film . Biosens. Bioelectron. , 10 : 675 – 682 .
- Douwes , J. , Thorne , P. , Pearce , N. and Heederik , D. 2003 . Bioaerosol Health Effects and Exposure Assessment: Progress and Prospects . Anns. Occup. Hyg. , 3 : 187 – 200 .
- Eninger , R. M. , Hogan , C. J. , Biswas , P. , Adhikari , A. , Reponen , T. and Grinshpun , S. A. 2009 . Electrospray Versus Nebulization for Aerosolization and Filter Testing with Virus Particles . Aerosol Sci. Technol. , 43 : 298 – 304 .
- Eninger , R. M. , Adhikari , A. , Reponen , T. and Grinshpun , S. A. 2008a . Differentiating Between Physical and Viable Penetrations When Challenging Respirator Filters with Bioaerosols . CLEAN – Soil, Air, Water , 36 : 615 – 621 .
- Eninger , R. M. , Honda , T. , Adhikari , A. , Heinonen-Tanski , H. , Reponen , T. and Grinshpun , S. A. 2008b . Filter Performance of N99 and N95 Facepiece Respirators Against Viruses and Ultrafine Particles . Ann. Occup. Hyg. , 52 : 385 – 396 .
- Enserink , M. 2002 . Bioterrorism: How Devastating Would a Small Pox Attack Really be . Science , 296 : 1592 – 1595 .
- Evgrafov , D. , Rodriguez , M. , Effery , J. W. , Norman , R. P. and Mark , T. H. 2010 . Molecular Source Tracking of Bioaerosols in the Quarantined Katrina Flood Zone . Aerosol Sci. Technol., , 44 : 230 – 239 .
- Fallschissel , K. , Kampfer , P. and Jackel , U. 2009 . Direct Detection of Salmonella Cells in the Air of Livestock Stables by Real-Time PCR . Ann. Occup. Hyg. , 53 : 859 – 868 .
- Fergenson , D. P. , Pitesky , M. E. , Tobias , H. J. , Steele , P. T. , Czerwieniec , G. A. Russell , S. C. 2004 . Reagentless Detection and Classification of Individual Bioaerosol Particles in Seconds . Anal. Chem. , 76 : 373 – 378 .
- Flesch , J. P. , Norris , C. H. and Nugent , A. E. Jr . 1967 . Calibradting Particulate Air Samplers with Monodisperse Aerosols – Application to Andersen Cascade Impactor . Am. Ind. Hyg. Assoc. J. , 28 : 507 – 516 .
- Foarde , K. K. , Hanley , J. T. and Veeck , A. C. 2000 . Efficacy of Antimicrobial Filter Treatments . Ashrae. J. , 42 : 52 – 58 .
- Fung , F. and Hughson , W. G. 2003 . Health Effects of Indoor Fungal Bioaerosol Exposure . Appl. Occup. Environ. Hyg. , 18 : 535 – 544 .
- Gehring , U. , Bolte , G. , Borte , M. , Bischof , W. , Fahlbusch , B. Wichmann , H. E. 2001 . Exposure to Endotoxin Decreases the Risk of Atopic Eczema in Infancy: A Cohort Study . J. Allergy Clin. Immunol. , 108 : 847 – 854 .
- Georgakopoulos , D. G. , Després , V. , Frölich-Nowosky , J. , Psenner , R. , Ariya , P. A. , Pósfai , M. , Ahern , H. E. , Moffett , B. F. and Hill , T. C. J. 2009 . Microbiology and Atmospheric Processes: Biological, Physical, and Chemical Characterization of Aerosol Particles . Biogeoscience , 6 : 724 – 737 .
- Görner , P. , Fabries , J. F. , Duquenne , P. , Witschger , O. and Wrobel , R. 2006 . Bioaerosol Sampling by a Personal Rotating Cup Sampler CIP 10-M . J. Environ. Monit. , 8 : 43 – 48 .
- Górny , R. L. , Reponen , T. , Grinshpun , S. A. and Willeke , K. 2001 . Source Strength of Fungal Spore Aerosolization from Moldy Building Material . Atmos. Environ. , 35 : 4853 – 4862 .
- Greenburg , L. and Smith , G. W. 1922 . A New Insutrument for Sampling Aerial Dust. Report of Investatigatioins, Serial No. 2392 , Pittsburgh, PA : Department of the Interior. US Bureau of Mines .
- Grinshpun , S. A. , Adhikari , A. , Cho , S. H. , Kim , K. Y. , Lee , T. and Reponen , T. 2007a . Small Change in the Design of a Slit Bioaerosol Impactor Significantly Improves Its Collection Characteristics . J. Environ. Monit. , 9 : 855 – 861 .
- Grinshpun , S. A. , Adhikari , A. , Honda , T. , Kim , K. Y. , Toivola , M. Rao , K. S. R. 2007b . Control of Aerosol Contaminants in Indoor Air: Combining the Particle Concentration Reduction with Microbial Inactivation . Environ. Sci. Technol. , 41 : 606 – 612 .
- Grinshpun , S. A. , Adhikari , A. , Li , C. , Reponen , T. , Yermakov , M. Schoenitz , M. 2010 . Thermal Inactivation of Airborne Viable Bacillus Subtilis Spores by Short-Term Exposure in Axially Heated Air Flow . J. Aerosol Sci. , 41 : 352 – 363 .
- Guan , T. and Yao , M. 2010 . Use of Carbon Nanotube Filter in Removing Bioaerosols . J. Aerosol Sci. , 6 : 611 – 620 .
- Haatainen , S. , Laitinen , J. , Linnainmaa , M. , Reponen , T. and Kalliokoski , P. 2010 . The Suitability of the IOM Foam Sampler for Bioaerosol Sampling in Occupational Environments . J. Occup. Environ. Hyg. , 7 : 1 – 6 .
- Haldane , J. and Anderson , A. 1887 . The Carbonic Acid, Organic Matter, and Micro-Organisms in Air, More Especially of Dwellings and Schools . Philos. Trans. R. Soc. Lond. , 178 : 61 – 111 .
- Han , T. , An , H. R. and Mainelis , G. 2010 . Performance of an Electrostatic Precipitator with Superhydrophobic Surface when Collecting Airborne Bacteria . Aerosol Sci. Technol. , 44 : 339 – 348 .
- Han , T. and Mainelis , G. 2008 . Design and Development of an Electrostatic Sampler for Bioaerosols with High Concentration Rate . J. Aerosol Sci. , 39 : 1066 – 1078 .
- Haugland , R. A. , Brinkman , N. and Vesper , S. J. 2002 . Evaluation of Rapid DNA Extraction Methods for the Quantitative Detection of Fungi Using Real-Time PCR Analysis . J. Microbiol. Methods , 50 : 319 – 323 .
- Ho , J. 2002 . Future of Biological Aerosol Detection . Anal. Chim. Acta. , 457 : 125 – 148 .
- Ho , J. , Spence , M. and Fisher , G. Detection of BW Agents: Dugway Trinational BW Field Trial 20–30 October 1993, Dugway, Utah, Mobile Aerosol Sampling Unit Data Analysis . Report no. DRES-619. Defence Research Establishment Suffield, . Ralston, Alberta, Canada.
- Hogan , C. J. , Lee , M. H. and Biswas , P. 2004 . Capture of Viral Particles in Soft X-Ray-Enhanced Corona Systems: Charge Distribution and Transport Characteristics . Aerosol Sci. Technol. , 38 : 475 – 486 .
- Holt , P. S. , Mitchell , B. W. , Seo , K. H. and Gast , R. K. 1999 . Use of Negative Air Ionization for Reducing Airborne Levels of Salmonella enterica serovar Enteritidis in a Room Containing Infected Caged Layers . J. Appl. Poult. Res. , 8 : 440 – 446 .
- Hospodsky , D. , Yamamoto , N. and Peccia , J. 2010 . Accuracy, Precision, and Method Detection Limits of Quantitative PCR for Airborne Bacteria and Fungi . Appl. Environ. Microbiol. , 76 : 7004 – 7012 .
- Huffman , J. A. , Treutlein , B. and P¨oschl , U. 2010 . Fluorescent Biological Aerosol Particle Concentrations and Size Distributions Measured with an Ultraviolet Aerodynamic Particle Sizer (UV-APS). in Central Europe . Atmos. Chem. Phys. , 10 : 3215 – 3233 .
- Ide , T. , Kumashiro , R. , Koga , Y. , Tanaka , E. , Hino , T. Hisamochi , A. 2003 . A Real-Time Quantitative Polymerase Chain Reaction Method for Hepatitis B Virus in Patients with Chronic Hepatitis B Treated with Lamivudine . Am. J. Gastroenterol. , 98 : 2048 – 2051 .
- Jones , W. , Morring , K. , Morey , P. and Sorenson , W. 1985 . Evaluation of the Andersen Viable Impactor for Single Stage Sampling . Am. Ind. Hyg. Assoc. J. , 46 : 294 – 298 .
- Jung , J. H. , Lee , J. E. and Kim , S. S. 2009a . Thermal Effects on Bacterial Bioaerosols in Continuous Air Flow . Sci. Total Environ. , 407 : 4723 – 4730 .
- Jung , J. H. , Lee , J. E. and Kim , S. S. 2009b . Generation of Nonagglomerated Airborne Bacteriophage Particles Using an Electrospray Technique . Anal. Chem. , 81 : 2985 – 2990 .
- Jung , J. H. , Lee , J. E. , Lee , C. H. , Kim , S. S. and Lee , B. U. 2009 . Treatment of Fungal Boaerosols by a High-Temperature, Short-Time Process in a Continuous-Flow System . Appl. Environ. Microbiol. , 75 : 2742 – 2749 .
- Jung , J. , Lee , J. , Hwang , G. , Lee , B. , Lee , S. Jurng , J. 2010 . Reagentless Detection and Classification of Individual Bioaerosol Particles in Seconds . Anal. Chem. , 82 : 664 – 671 .
- Kanaani , H. , Hargreaves , M. , Smith , J. , Ristovskia , Z. , Agranovskia , V. and Morawska , L. 2008 . Performance of UVAPS With Respect to Detection of Airborne Fungi . J. Aerosol Sci. , 39 : 175 – 189 .
- Kang , S. , Mauter , M. S. and Elimelech , M. 2009 . Microbial Cytotoxicity of Carbon-Based Nanomaterials: Implications for River Water and Wastewater Effluent . Environ. Sci. Technol. , 43 : 2648 – 2653 .
- Keegan , J. J. 1918 . The Prevailing Pandemic of Influenza . J. Am. Med. Assoc. , 71 : 1051 – 1055 .
- Kim , K. , Kim , W. , Yun , S. H. , Lee , J. H. , Kim , S. and Lee , B. U. 2008 . Use of an Electrospray for the Generation of Bacterial Bioaerosols . J. Aerosol Sci. , 39 : 365 – 372 .
- King , M. D. , Thien , B. F. , Tiirikainen , S. and McFarland , A. R. 2009 . Collection Characteristics of a Batch-Type Wetted Wall Bioaerosol Sampling Cyclone . Aerobiologia , 25 : 239 – 247 .
- Kujundzic , E. , Hernandez , M. and Miller , S. L. 2007 . Ultraviolet Germicidal Irradiation Inactivation of Airborne Fungal Spores and Bacteria in Upper-Room Air and In-Duct Configurations . J. Environ. Eng. Sci. , 6 : 1 – 9 .
- Kujundzic , E. , Matalkah , F. , Howard , C. J. , Hernandez , M. and Miller , S. L. 2006 . Air Cleaners and Upper-Room Air UV Germicidal Irradiation for Controlling Airborne Bacteria and Fungal Spores . J. Occup. Environ. Hyg. , 3 : 536 – 546 .
- Kujundzic , E. , Zander , D. A. , Hernandez , M. , Angenent , L. T. , Henderson , D. E. and Miller , S. L. 2005 . Effects of Ceiling-Mounted HEPA-UV Air Filters on Airborne Bacteria Concentrations in an Indoor Therapy Pool Building . J. Air Waste Manag. Assoc. , 55 : 210 – 218 .
- Kummer , V. 2009 . Technologies and Control Measures for Reducing Bioaerosols in Livestock Farming According to the Guideline VDI 4255, Part 2 . Gefahrstoffe Reinhaltung der luft , 69 : 343 – 347 .
- Kuske , C. R. 2006 . Current and Emerging Technologies for the Study of Bacteria in the Outdoor Air . Curr. Opin. Biotechnol. , 17 : 291 – 296 .
- Lee , J. H. , Wu , C. Y. , Wysocki , K. M. , Farrah , S. and Wander , J. 2008a . Efficacy of Iodine-Treated Biocidal Filter Media Against Bacterial Spore Aerosols . J. Appl. Microbiol. , 105 : 1318 – 1326 .
- Lee , S. A. , Willeke , K. , Mainelis , G. , Adhikari , A. , Wang , H. , Reponen , T. and Grinshpun , S. A. 2004 . Assessment of Electrical Charge on Airborne Microorganisms by a New Bioaerosol Sampling Method . J. Occup. Environ. Hyg. , 1 : 127 – 138 .
- Lee , S. H. , Lee , H. J. , Hyung , S. J. , Lee , H. M. , Kang , H. J. and Kim , Y. P. 2010 . Identification of Airborne Bacterial and Fungal Community Structures in an Urban Area by T-RFLP Analysis and Quantitative Real-Time PCR . Sci. Total Environ. , 408 : 1349 – 1357 .
- Lee , S. , Choi , B. , Yi , S. M. and Ko , G. 2009 . Characterization of Microbial Community During Asian Dust Events in Korea . Sci. Total Environ. , 407 : 5308 – 5314 .
- Lee , S. , Grinshpun , S. and Reponen , T. 2008b . Respiratory Performance Offered by N95 Filtering Facepiece Respirators and Surgical Masks: Human-Subject Evaluation with NaCl Aerosol Representing Bacterial and Viral Particle Size Range . Anns. Occup. Hyg. , 52 : 177 – 185 .
- Lesage , P. 1901 . Germination of the Spores of Penicillium in Humid Air . Comptes Rendus Hebdomadaires Des Seances De L Academie Des Sciences, , : 174 – 176 . 133
- Lester , D. E. and Ponce , A. 2002 . An Anthrax “Smoke” Detector . IEEE Eng. Med. Biol. , 21 : 38 – 42 .
- Macfadyen , A. 1899 . On the Infuence of the Temperature of Liquid Air on Bacteria . Lancet , 155 : 849 – 849 .
- Mainelis , G. , Adhikari , A. , Willeke , K. , Lee , S. A. , Reponen , T. and Grinshpun , S. A. 2002a . Collection of Airborne Microorganisms by a New Electrostatic Precipitator . J. Aerosol Sci. , 33 : 1417 – 1432 .
- Mainelis , G. , Berry , D. , An , H. R. , Yao , M. , DeVoe , K. Fennell , D. E. 2005 . Design and Performance of a Single-Pass Bubbling Bioaerosol Generator . Atmos. Environ. , 39 : 3521 – 3533 .
- Mainelis , G. , Grinshpun , S. A. , Willeke , K. , Reponen , T. , Ulevicius , V. and Hintz , P. J. 1999 . Collection of Airborne Microorganisms by Electrostatic Precipitation . Aerosol Sci. Technol. , 30 : 127 – 144 .
- Mainelis , G. , Willeke , K. , Adhikari , A. , Reponen , T. and Grinshpun , S. A. 2002b . Design and Collection Efficiency of a New Electrostatic Precipitator for Bioaerosol Collection . Aerosol Sci. Technol. , 36 : 1073 – 1085 .
- Mainelis , G. , Willeke , K. , Baron , P. , Grinshpun , S. A. and Reponen , T. 2002c . Induction Charging and Electrostatic Classification of Micrometer-Size Particles for Investigating the Electrobiological Properties of Airborne Microorganisms . Aerosol Sci. Technol. , 36 : 479 – 491 .
- Mainelis , G. , Willeke , K. , Baron , P. , Reponen , T. , Grinshpun , S. A. Gorny , R. L. 2001 . Electrical Charges on Airborne Microorganisms . J. Aerosol Sci. , 32 : 1087 – 1110 .
- Mancinia , G. , Carbonaraa , A. O. and Heremans , J. F. 1965 . Immunochemical Quantitation of Antigens by Single Radial Immunodiffusion . Immunochemistry , 2 : 235 – 254 .
- May , K. R. 1964 . Calibration of a Modified Andersen Bacterial Aerosol Sampler . Appl. Environ. Microbiol. , 12 : 37 – 43 .
- McFarland , A. R. , Haglund , J. S. , King , M. D. , Hu , S. , Phull , M. S. Moncla , B. W. 2010 . Wetted Wall Cyclones for Bioaerosol Sampling . Aerosol Sci. Technol. , 44 : 241 – 252 .
- Meklin , T. , Reponen , T. , McKinstry , C. , Cho , S. H. , Gninshpun , S. A. Nevalainen , A. 2007 . Comparison of Mold Concentrations Quantified by MSQPCR in Indoor and Outdoor Air Sampled Simultaneously . Sci. Total Environ. , 382 : 130 – 134 .
- Menzies , D. , Popa , J. , Hanley , J. A. , Rand , T. and Milton , D. K. 2003 . Effect of Ultraviolet Germicidal Lights Installed in Office Ventilation Systems on Workers’ Health and Well being: Double-Blind Multiple Cross-over Trial . Lancet , 362 : 1785 – 1791 .
- Mitchell , B. W. and King , D. J. 1994 . Effect of Negative Air Ionization on Airborne Transmission of Newcastle Disease Virus . Avian Dis. , 38 : 725 – 732 .
- National Research Council . 2005 . Sensor Systems for Biological Agent Attacks: Protecting Buildings and Military Bases , Washington, DC : The National Academies Press .
- Negrin , M. M. , Del Panno , M. T. and Ronco , A. E. 2007 . Study of Bioaerosols and Site Influence in the La Plata Area (Argentina). Using Conventional and DNA (Fingerprint)-Based Methods . Aerobiologia, , 23 : 249 – 258 .
- Nehme , B. , Letourneau , V. , Forster , R. J. , Veillette , M. and Duchaine , C. 2008 . Culture-Independent Approach of the Bacterial Bioaerosol Diversity in the Standard Swine Confinement Buildings, and Assessment of the Seasonal Effect . Environ. Microb. , 10 : 665 – 675 .
- Noble , P. A. , Dziuba , M. , Harrison , D. J. and Albritton , W. L. 1999 . Factors Influencing Capacitance-Based Monitoring of Microbial Growth . J. Microbiol. Methods , 37 : 51 – 64 .
- Oppliger , A. , Charriere , N. , Droz , P. O. and Rinsoz , T. 2008 . Exposure to Bioaerosols in Poultry Houses at Different Stages of Fattening; Use of Real-Time PCR for Airborne Bacterial Quantification . Anns. Occup. Hyg. , 52 : 405 – 412 .
- Pal , A. , Pehkonen , S. O. , Yu , L. E. and Ray , M. B. 2007 . Photocatalytic Inactivation of Gram-Positive and Gram-Negative Bacteria Using Fluorescent Light . J. Photochem. Photobio. A-Chem. , 186 : 335 – 341 .
- Park , D. , Kim , Y.-H. , Park , C. W. , Hwang , J. and Kim , Y.-J. 2009 . New Bio-Aerosol Collector Using a Micromachined Virtual Impactor . J. Aerosol Sci. , 40 : 415 – 422 .
- Pasteur , L. 1861 . On the Organized Bodies which Exist in the Atmosphere . Annales des Sciences Naturelles, 4th ser. , 16 : 5 – 98 .
- Patolsky , F. , Zheng , G. F. , Hayden , O. , Lakadamyali , M. , Zhuang , X. W. and Lieber , C. M. 2004 . Electrical Detection of Single Viruses . Proc. Natl. Acad. Sci. USA , 101 : 14017 – 14022 .
- Paul , C. and Isard , S. 1999 . Aerobiology: Coming of Age in a New Millennium . Aerobiologia , 15 : 259 – 266 .
- Peccia , J. and Hernandez , M. 2001 . Photoreactivation in Airborne Mycobacterium Parafortuitum . Appl. Environ. Microbiol. , 67 : 4225 – 4232 .
- Peccia , J. and Hernandez , M. 2006 . Incorporating Polymerase Chain Reaction-Based Identification, Population Characterization, and Quantification of Microorganisms into Aerosol Science: A Review . Atmos. Environ. , 40 : 3941 – 3961 .
- Rengasamy , A. , Zhuang , Z. Q. and Berry , R. A. 2004 . Respiratory Protection Against Bioaerosols: Literature Review and Research Needs . Am. J. Infect Control , 32 : 345 – 354 .
- Reponen , T. , Willeke , K. , Grinshpun , S. and Nevalainen , A. 2011 . “ Biological Particle Sampling ” . In Aerosol Measurement, Principles, Techniques, and Applications , 3rd ed. , Edited by: Kulkarni , P. , Baron , P. and Willeke , K. San Francisco, CA : John Wiley & Sons .
- Rietsch , G. 1904 . Sensitivity of Eberth's Bacillus to Ozonized Air . Comptes Rendus Des Seances De La Societe De Biologie Et De Ses Filiale, , 56 : 1102 – 1105 .
- Rinsoz , T. , Duquenne , P. , Greff-Mirguet , G. and Oppliger , A. 2008 . Application of Real-Time PCR for Total Airborne Bacterial Assessment: Comparison with Epifluorescence Microscopy and Culture-Dependent Methods . Atmos. Environ. , 42 : 6767 – 6774 .
- Robbins , C. A. , Swenson , L. J. , Nealley , M. L. , Gots , R. E. and Kelman , B. J. 2000 . Health Effects of Mycotoxins in Indoor Air: A Critical Review . Appl. Occup. Environ. Hyg. , 15 : 773 – 784 .
- Rooks , R. 1948 . A Device for the Electrostatic Precipitation of Bacteria and Fungus Spores upon Culture Plates . J. Allergy , 19 : 200 – 205 .
- Rosenstern , I. 1948 . Control of Air-Borne Infections in a Nursery for Young Infants . Am. J. Dis. Child. , 75 : 193 – 202 .
- Ryan , K. , McCabe , K. , Clements , N. , Hernandez , M. and Miller , S. L. 2010 . Inactivation of Airborne Microorganisms Using Novel Ultraviolet Radiation Sources in Reflective Flow Through Control Devices . Aerosol Sci. Technol. , 44 : 541 – 550 .
- Rylander , R. 1999 . Indoor Air-Related Effects and Airborne (1–> 3)-beta-D-glucan . Environ. Health Perspect. , 107 : 501 – 503 .
- Rylander , R. 2002 . Review: Endotoxin in the Environment – Exposure and Effects . J. Endotoxin Res., , 8 : 241 – 252 .
- Saik , R. , Gelfand , D. H. , Stoffel , S. , Scharf , S. J. , Higuchi , R. Horn , G. T. 1988 . Primer-Directed Enzymatic Amplification of DNA with a Thermostable DNA Polymerase . Science , 239 : 487 – 491 .
- Sanchez-Monedero , M. A. , Stentiford , E. I. and Mondini , C. 2003 . Biofiltration at Composting Facilities: Effectiveness for Bioaerosol Control . Environ. Sci. Technol. , 37 : 4299 – 4303 .
- Schena , M. , Shalon , D. , Davis , R. W. and Brown , P. O. 1995 . Quantitative Monitoring of Gene Expression Patterns with a Complementary DNA Microarray . Science , 270 : 467 – 470 .
- Schoen , D. T. , Schoen , A. P. , Hu , L. , Kim , H. S. , Heilshorn , S. C. and Cui , Y. 2010 . High Speed Water Sterilization Using One-Dimentional Nanostructures . Nano Letters , 10 : 3628 – 3632 .
- Sengupta , A. , Brar , N. and Davis , E. J. 2007 . Bioaerosol Detection and Characterization by Surface-Enhanced Raman Spectroscopy . J. Cournal Colloid Interfaces Sci. , 309 : 36 – 43 .
- Shaffer , R. E. and Rengasamy , S. 2009 . Respiratory Protection Against Airborne Nanoparticles: A Review . J. Nano. Res. , 11 : 1661 – 1672 .
- Sillanpä , M. , Gellera , M. D. , Phuleria , H. C. and Sioutas , C. 2008 . High Collection Efficiency Electrostatic Precipitator for In Vitro Cell Exposure to Concentrated Ambient Particulate Matter (PM) . J. Aerosol Sci. , 39 : 335 – 347 .
- Silley , P. and Mortimer , F. 2003 . Rapid Microbiological Methods in the Pharmaceutical Industry , Washington, DC : CRC Press .
- So , H. M. , Won , K. , Kim , Y. H. , Kim , B. K. , Ryu , B. H. Na , P. S. 2005 . Single-Walled Carbon Nanotube Biosensors Using Aptamers as Molecular Recognition Elements . J. Am. Chem. Soc. , 127 : 11906 – 11907 .
- Solomon , W. R. 1970 . A Simplified Application of the Andersen Sampler to the Study of Airborne Fungus Particles . J. Allergy , 45 : 1 – 13 .
- Suehiro , J. , Hamada , R. , Noutomi , D. , Shutou , M. and Hara , M. 2003 . Selective Detection of Viable Bacteria Using Dielectrophoretic Impedance Measurement Method . J. Electrost. , 57 : 157 – 168 .
- Tan , M. , Shen , F. , Yao , M. and Zhu , T. 2011 . Development of an Automated Electrostatic Sampler (AES) for Bioaerosol Detection . Aerosol Sci. Techno. , 45 : 1154 – 1160 .
- Tischer , C. , Gehring , U. , Chen , C-M. , Kerkhof , M. , Koppelman , G. Sausenthaler , S. 2011 . Respiratory Health in Children, and Indoor Exposure to (1,3)-β-d-glucan, EPS Mould Components and Endotoxin . ERJ , 37 : 1050 – 1059 .
- Tobias , H. J. , Schafer , M. P. , Pitesky , M. , Fergenson , D. P. , Horn , J. Frank , M. 2005 . Bioaerosol Mass Spectrometry for Rapid Detection of Individual Airborne Mycobacterium Tuberculosis H37Ra Particles . Appl. Environ. Microbiol. , 71 : 6086 – 6095 .
- Tolman , C. R. , Guernsey , W. E. , Charleston , D. V. and Dougherty , H. R. 1919 . The Protection Afforded by Various Filters Against Bacterial Suspensions in Air . J. Infect. Dis. , 24 : 637 – 647 .
- Tseng , C. C. and Li , C. S. 2005 . Inactivation of Virus-Containing Aerosols by Ultraviolet Germicidal Irradiation . Aerosol Sci. Technol. , 39 : 1136 – 1142 .
- Uffenheimer , A. 1902 . A New Air Borne Bacillus (Bacillus Aerogenes agilis, nov spec.) . Beitrage Zur Pathologischen Anatomie Und Zur Allgemeinen Pathologie, , 31 : 383 – 418 .
- Ulevicius , V. , Willeke , K. , Grinshpun , S. A. , Donnelly , J. , Lin , X. J. and Mainelis , G. 1997 . Aerosolization of Particles from a Bubbling Liquid: Characteristics and Generator Development . Aerosol Sci. Technol. , 26 : 175 – 190 .
- Wells , H. B. 1905 . Smallpox Hospitals and Air Borne Contagion . J. Am. Med. Assoc. , 45 : 56
- West , J. S. , Atkins , S. D. , Emberlin , J. and Fitt , B. D. L. 2008 . PCR to Predict Risk of Airborne Disease . Trends Microbiol. , 16 : 380 – 387 .
- Whitesides , M. G. 2006 . The Origins and the Future of Microfluidics . Nature , 442 : 368 – 373 .
- Willeke , K. , Lin , X. J. and Grinshpun , S. A. 1998 . Improved Aerosol Collection by Combined Impaction and Centrifugal Motion . Aerosol Sci. Technol. , 28 : 439 – 456 .
- Willeke , K. , Ulevicious , V. , Reponen , T. and Grinshpun , S. A. 1996 . Development and Evaluation of Aerosol Generators for Biological Materials . J. Aerosol Sci. (Suppl.) , 27 : s249 – s250 .
- Wilson , W. J. , Erler , A. M. , Nasarabadi , S. L. , Skowronski , E. W. and Imbro , P. M. A. 2005 . Multiplexed PCR-Coupled Liquid Bead Array for the Simultaneous Detection of Four Biothreat Agents . Mol. Cell Probe , 19 : 137 – 144 .
- Winslow , C. E. A. 1908 . A New Method of Enumerating Bacteria in Air . Science , 28 : 28 – 31 .
- Wolf , H. , Skaliy , P. , Hall , L. , Harris , M. , Decker , H. Buchanan , L. 1959 . Sampling Microbiological Aerosols , Washington, DC : US Department of Health, and Welfare .
- Wu , J. , Ben , Y. and Chang , H. C. 2005 . Particle Detection by Electrical Impedance Spectroscopy with Asymmetric-Polarization AC Electroosmotic Trapping . Microfluid. Nanofluid., , 1 : 161 – 167 .
- Wu , Y. and Yao , M. 2010 . Inactivation of Bacteria and Fungus Aerosols Using Microwave Irradiation . J. Aerosol Sci. , 41 : 682 – 693 .
- Xu , P. , Kujundzic , E. , Peccia , J. , Schafer , M. P. , Moss , G. Hernandez , M. 2005 . Impact of Environmental Factors on Efficacy of Upper-Room Air Ultraviolet Germicidal Irradiation for Inactivating Airborne Mycobacteria . Environ. Sci. Technol. , 39 : 9656 – 9664 .
- Xu , P. , Peccia , J. , Fabian , P. , Martyny , J. W. , Fennelly , K. P. Hernandeza , M. 2003 . Efficacy of Ultraviolet Germicidal Irradiation of Upper-Room Air in Inactivating Bacterial Spores and Mycobacteria in Full-Scale Studies . Atmos. Environ. , 37 : 405 – 419 .
- Xu , Z. and Yao , M. 2011 . Effects of Single-Walled Carbon Nanotube Filter on Culturability and Diversity of Environmental Bioaerosols . J. Aerosol Sci. , 42 : 387 – 396 .
- Yang , L. 2008 . Electrical Impedance Spectroscopy for Detection of Bacterial Cells in Suspensions Using Interdigitated Microelectrodes . Talanta , 74 : 1621 – 1629 .
- Yang , L. and Bashir , R. 2008 . Electrical/Electrochemical Impedance for Rapid Detection of Foodborne Pathogenic Bacteria . Biotechno. Adv. , 26 : 135 – 150 .
- Yang , L. , Li , Y. and Erf , G. F. 2004 . Interdigitated Array Microelectrode-Based Electrochemical Impedance Immunosensor for Detection of Escherichia Coli O157: H7 . Anal. Chem. , 76 : 1107 – 1113 .
- Yao , M. and Mainelis , G. 2006 . Investigation of Cut-off Sizes and Collection Efficiencies of Portable Microbial Samplers . Aerosol Sci. Technol. , 40 : 595 – 606 .
- Yao , M. , Mainelis , G. and An , H. R. 2005 . Inactivation of Microorganisms Using Electrostatic Fields . Environ. Sci. Technol., , 39 : 3338 – 3344 .
- Yao , M. , Wu , Y. , Zhen , S. and Mainelis , G. 2009a . A Comparison of Airborne and Dust-Borne Allergens and Toxins Collected from Home, Office, and Outdoor Environments Both in New Haven, United States and Nanjing, China . Aerobiologia , 25 : 183 – 192 .
- Yao , M. , Zhang , H. , Dong , S. , Zhen , S. and Chen , X. 2009b . Comparison of Electrostatic Collection and Liquid Impinging Methods When Collecting Airborne House Dust Allergens, Endotoxin and (1,3).-β-d-glucans . J. Aerosol Sci. , 40 : 492 – 502 .
- Zhang , Q. , Damit , B. , Welch , J. , Park , H. , Wu , C. Y. and Sigmund , W. 2010 . Microwave-Assisted Nanofibrous Air Filtration for Disinfection of Bioaerosols . J. Aerosol Sci. , 41 : 880 – 888 .