Abstract
This article presents a process to calibrate a condensation particle counter (CPC) over the concentration range from 1 particle·cm−3 to 104 particles·cm−3 with traceability to SI units as realized by the National Institute of Standards and Technology (NIST). The process combines two independent steps: a proportionality assessment over the range of the CPC using a consistent diluter and an absolute calibration of the CPC with an aerosol electrometer (AE) at high concentrations. The proportionality assessment, which is the focus of the article, is analogous to the attenuation method for testing the proportionality of high-power laser detectors. This procedure tests the proportionality of one CPC by itself and does not require the use of a calibrated reference CPC. The primary calibration of the CPC with the AE at high concentrations allows for an absolute calibration with NIST traceability, resulting in a simple one-parameter correction to the measured CPC data. The proportionality test enables uncertainties to be assigned to the CPC over an extended range of concentration beyond the lower detection limit of the AE, thereby establishing measurement traceability for low concentrations. The relative expanded uncertainty of the CPC with a coverage factor of k = 2 is 2.8% over the range of about 1 particle·cm−3 to 104 particles·cm−3.
1. INTRODUCTION
In many cases, it is required to perform aerosol number concentration measurements with a condensation particle counter (CPC) over at least four orders of magnitude from 1 particle·cm−3 to 104 particles·cm−3. Specifically, it is important to the military as the CPC is used to verify the ability of quantitative gas mask fit testers to measure the leakage of a test aerosol into military-issue gas masks. Another application of using a CPC over four orders of magnitude is the counting of macromolecules (Lewis et al. 1994). There have also been a number of recent studies on CPC performance motivated by legislated vehicle particle emission measurements. These studies have focused on the effects of particle size, particle type, and concentration on the CPC performance (Giechaskiel et al. 2009; Wang et al. Citation2010). The calibrations of the CPC versus concentration in these studies have been for concentrations larger than 103 particles·cm−3 for which the aerosol electrometer (AE) is a traceable measurement. However, legal requirements call for calibration at much lower concentrations.
Calibrations of a CPC (3760A, TSI, Inc.)Footnote 1 with a monodisperse aerosol have been previously established by comparison with an AE (Fletcher et al. Citation2009; Yli-Ojanpera et al. Citation2010). The comparison of the CPC to the AE is an absolute calibration of the CPC with traceability to SI units. However, due to the limitations of the AE, accurate measurements of aerosol concentration cannot be performed below approximately 103 particles·cm−3 for typical CPCs. Transmission electron microscopy has also been used for CPC calibration, but it is not practical at low concentrations due to poor statistics resulting from the low number of particles in the field of view. Thus, there is currently a lack of an absolute measurement standard for low aerosol number concentration of submicrometer and ultrafine particles.
Since a CPC is expected to have a proportional response at low concentrations, it is common practice to calibrate a CPC with an AE at high concentration and force a linear regression through the origin. This is a practical approach often used for concentrations below the AE calibration point. A CPC calibrated with an AE may also be used to calibrate other CPCs for concentration well below the AE calibration point. However, this approach is not satisfactory for laboratories that require legal and traceable calibrations of CPCs at concentrations below that which can be verified by an AE, because traceability can only be established through an unbroken chain of calibrations with a complete analysis of the associated uncertainty (Taylor and Kuyatt Citation1994). Also calibration laboratory policies may limit extrapolation to a small region beyond the calibration data. Furthermore, rigorous linear least squares regression theory applied to the CPC and AE calibration data will sometimes produce a linear fit with a statistically significant ordinate intercept, causing discrepancies when extrapolating to low concentrations, or a linear least squares fit of the log of the concentration is used, resulting in a nonlinear relationship between the CPC and the AE (Fletcher et al. Citation2009). To establish measurement traceability at low concentrations, this article describes a calibration process that tests the proportionality of a CPC over four orders of magnitude using a diluter.
The focus of this study is to calibrate a CPC over the concentration range from 1 particle·cm−3 to 104 particles·cm−3 with 2%–3% relative expanded uncertainty and traceability to SI units as realized by NIST. This is accomplished by using an independent method of verifying proportional counting by the CPC, combined with a previous measurement of a traceable proportionality constant by comparison of the CPC to the AE at high concentrations performed by NIST (Fletcher et al. Citation2009). This results in a simple one-parameter correction to the measured CPC data but offers greater assurance that the response of the CPC is actually maintaining fixed proportionality throughout the full range of potential measurement results.
Several approaches to verifying the CPC response at low concentrations were considered, all involving diluters. Initially, as the first approach, the development of a traceable diluter capable of diluting by as much as 104 was considered. Brockmann et al. (Citation1984) developed an extraction diluter and Hueglin et al. (Citation1997) a rotating cavity diluter, both with the ability to dilute by four orders of magnitude. However, these dilution measurements were not traceable measurements. A traceable measurement would require an accurate measurement of diluter flow rates and of the particle losses through the diluter, along with a calibrated reference particle detector. The second approach considered involves using two reference CPCs calibrated with an AE from about 103 to 104 particles·cm−3 to calibrate a diluter for a factor of about 10. The diluter is then used to calibrate one reference CPC at about 102 particles·cm−3 by diluting down from the lowest calibrated CPC concentration. Next, the diluter is adjusted to give a factor of about 102, using the highest CPC calibration point and the previously diluted low concentration. This process is continued until the lowest desired CPC concentration is calibrated. While the diluter used in this scheme is simple and inexpensive, the data analysis can be quite involved when multiple dilutions are performed and the uncertainty increases with each successive dilution.
The third approach, which is the focus of this study, is analogous to the attenuation method for testing the proportionality of high-power laser detectors (Li et al. Citation2004). The proportionality test of the laser detector uses a rotating optical chopper as a consistent attenuator. The detector output voltage is measured with and without the attenuator as the laser power is varied from 1 to about 1000 W. For a proportional detector, the measured attenuation will be the same at each power level. In our case, a consistent diluter serves the role of the attenuator.
The principle of the proportionality test can be described based on a single CPC together with a constant output aerosol source and a diluter with a fixed and constant dilution factor. To perform the proportionality test, the aerosol concentration is first measured with the CPC. Next, the concentration is measured with a diluter inserted between the source and the CPC. This process is shown in . These two measurements are then repeated at different source concentration levels over the range of the CPC. For a CPC with a perfectly proportional response to concentration, the ratio of the diluted and undiluted measurements at each concentration level will be equal. This is because the coefficient of proportionality that relates the CPC response to concentration cancels when the ratio is computed. This procedure tests the proportionality of one CPC by itself and is not a calibration of the proportionality of one CPC versus another CPC.
In the actual measurement, the aerosol source is not perfectly constant and it is difficult to insert and remove a diluter between the CPC and the aerosol source without affecting the source. To overcome these two problems, two CPCs are used to take simultaneous measurements: one dummy CPC measures the undiluted source concentration and a second CPC, the unit being calibrated, measures the diluted concentration. This process is shown in . Any drift in the source can be accounted for because of simultaneous measurement. Also the source and all flows are more stable because the diluter is not inserted and removed repeatedly. A relative comparison between the dummy CPC and the CPC to be calibrated is performed at cardinal concentration points that will be used during the proportionality calibration, and a correction factor is computed at each of these cardinal points. This allows the measurement taken by the dummy CPC to be representative of what the CPC under calibration would take when measuring the undiluted aerosol source. Also since this is just a proportionality test of the CPC, neither CPC needs to have an absolute calibration.
Once the CPC is verified to be proportional over the full range of concentrations necessary, the coefficient of proportionality is measured by comparison with an AE at high concentration. This comparison results in an absolute calibration of the CPC and provides traceability to SI units through the NIST realizations of the volt and the ohm. This approach takes advantage of the capabilities of the AE, using it only at the highest concentrations that the CPC can measure, so that measurement uncertainty of the CPC calibration can be reduced.
This report proceeds by describing the experimental setup, the diluter validation process, the calibration process, results, and the uncertainty analysis.
2. EXPERIMENTAL SETUP
The experimental setup for the proportionality test consists of an aerosol generator connected to an aerosol mixer and a splitter that is used to simultaneously expose two CPCs to the calibration aerosol. A fixed diluter is installed at the inlet of the CPC under test to perform the proportionality measurement. The system uses a differential mobility analyzer (DMA) to produce a monodisperse emery oil (1-decene tetramer mixed with 1-decene trimer, hydrogenated; also identified as PAO 4 cSt) aerosol of 100 nm particles in dry air, with a stable and adjustable concentration from about 1 particle·cm−3 to 104 particles·cm−3. The primary aerosol is generated with a Collison nebulizer that sprays a dilute solution of approximately 0.05 mL emery oil in 1 L of reagent-grade isopropanol alcohol. The DMA is operated without recirculation of the sheath air to minimize the concentration of isopropanol vapor flowing into the CPCs. The flow through the CPC critical orifice is inversely proportional to the square root of the average molecular weight of the gas so that the presence of isopropanol decreases the flow. For our conditions of an aerosol flow of 1.5 L/min with 50% makeup air downstream of the DMA, and based on the vapor pressure and diffusion coefficient of isopropanol, the estimated decrease in flow is of order 0.3% and is considered negligible for this study.
A single-stage micro-orifice uniform deposition impactor (MOUDI) with a cutoff size of 115 nm is used to produce a sharp cutoff of the primary aerosol size distribution generated from the Collison atomizer, reducing the number of larger multiply charged particles exiting the DMA. A Scanning Mobility Particle Sizer (SMPS) spectrometer (3936 SMPS, TSI, Inc.) is used to measure the calibration aerosol size distribution before being mixed and split between two CPCs. The size distribution measurements show that only about 0.3% of the aerosol is made up of larger diameter double-charged particles, and that all larger diameter particles with three or more charges are effectively eliminated. Before the SMPS, dry particle-free makeup air is introduced into the calibration aerosol to balance the flow through the DMA with the SMPS and CPC flows. The system is shown in .
3. DILUTER VALIDATION
To perform the proportionality test of the CPC, the diluter must produce a dilution factor that is stable with respect to time and across a range of aerosol concentrations. A simple adjustable diluter consisting of a flow restricting orifice, valve, and new high-efficiency particulate air (HEPA) filter was used to perform the proportionality test, as shown in . Other diluters can be used if they maintain a constant dilution factor over the range of concentrations of interest and over an extended period of time. It was verified that the penetration through the HEPA filter was less than 0.1% for concentrations in the range of 1 particle·cm−3 to 104 particles·cm−3. The total pressure drop across the diluter was measured at the positions labeled Pup and Pdown at the CPC inlets shown in .
For the dilution factor to be stable with respect to time, the diluter flow rates must be stable and the percentage of particle loss through the diluter must remain constant. The constancy of the flow through the diluter is monitored by the total pressure drop across the diluter, and pressure changes between the two flow paths within the diluter are minimized by using a new filter and testing for short time periods of less than 1 h. Alternatively, the pressure drop across the diluter orifice and filter can be monitored individually. To keep particle loss through the diluter constant, a straightforward way is to use a monodisperse aerosol with a constant particle diameter for all proportionality measurements, because size-dependent particle loss will be constant. In this study, the aerosol size distribution may shift with time as the solvent evaporates, since the aerosol is generated by nebulizing a mixture of isopropanol and emery oil. A change in the size distribution will result in a change in the fraction of multiply charged particles exiting the DMA. Since the losses through the diluter are size dependent, this may result in a slight change in the dilution ratio as a function of time. To avoid this issue, most of the multiply charged particles are removed by using an impactor downstream of the nebulizer. Finally, repeat measurements of the dilution factor measured at the initial test concentration are performed throughout the process to provide a further check of diluter drift with time.
Next, the diluter must be proven to be stable with respect to a changing aerosol concentration. There is the possibility of the flow decreasing at high particle concentrations. This possibility was assessed by measuring the change of the diluter flow rates when exposed to concentrations of 100 nm particles from 0 to 10,000 particles·cm−3. The flow through the diluter orifice was measured at high and low concentrations for three measurement sequences, and the ratio was within ±0.5% of unity for all three sequences. This value is equal to the standard uncertainty of the volume displacement flow meter (BIOS 510-L, Bios, Inc.) and is considered a negligible effect. The effect of aerosol concentration on the inlet flow of the CPC, which is the total diluter flow rate, is also measured at high and low concentrations. The volumetric flow rate did not change by more than 0.002 L/min, indicating that the total flow is not significantly affected by the challenge aerosol.
Since the diluter flow rates are verified to be constant and independent of the presence of aerosol particles over the concentration range of interest, and the particle losses through the diluter are constant with time, the diluter can be confidently used to perform the proportionality test.
4. TEST PROCEDURE
4.1. Direct Comparison
The direct comparison measurement is required to account for any differences between the two CPCs when measuring the dilution factor. The direct comparison process consists of two steps: measurement of the CPC inlet flow rates and the comparison of concentration measurements taken simultaneously by both CPCs. The setup in is used to perform these measurements, with the diluter for proportionality testing removed from the inlet of the CPC under calibration.
The inlet volumetric flow rate of each CPC is controlled by a critical orifice, providing a steady volumetric flow rate that is independent of pressure and is a weak function of temperature. Thus, a single flow measurement prior to performing concentration measurements is sufficient, given that the temperature does not change by more than 1°C. An accurate volume displacement flow meter (Bios Defender 510-M, Bios, Inc.) is used to measure the inlet flow. The measured flow rate is used to correct the concentration measurement, which is initially based on a nominal flow, and to perform the coincidence correction.
The direct comparison measurement is performed by splitting the flow from the aerosol source to both CPCs simultaneously. Measurements of concentration are taken at the seven test points of 10,000, 3000, 1000, 300, 100, 30, and 10 particles·cm−3. The ratio of concentration measured by the dummy CPC, Cdummy , to the CPC under test, CUT , is computed for each concentration. For example, the ratio for CUT = 10 particles·cm−3 and Cdummy = 10.1 particles·cm−3 is given by
As discussed below, this constant is used to correct the reading of the undiluted aerosol, Cdummy , to predict the undiluted reading for CUT when performing the proportionality test.
4.2. Proportionality Test
The dilution factor is measured using the setup in . The diluter is first adjusted to give a factor of about 10 to 1. The concentrations were then varied according to a randomized sequence of the prescribed seven test points of 10,000, 3000, 1000, 300, 100, 30, and 10 particles·cm−3. The CPC inlet temperatures were within ±0.1°C and the CPC inlet pressures were held constant within ±0.1% over the 1-h period for dilution measurements for a single day. The constancy of the inlet pressure and temperature are essential to minimize the drift in the dilution factor. The dilution factor, RD , is computed by using EquationEquation (2), where the concentration of the diluted aerosol, CUT , is in the numerator, and the concentration measured by the dummy CPC is corrected using the k factor from EquationEquation (1):
All concentrations were computed using 10-s sample intervals for a total collection time of 2 min to reduce the uncertainty due to Poisson counting errors, except for the highest concentration that was collected for 1 min. The average dilution factor for each measurement is computed as

5. RESULTS
5.1. Proportionality Test
The average dilution factor, , and the standard deviation of the mean,
, were calculated for each measurement. shows graphically that the dilution ratio is nearly constant over a wide range of concentrations with a narrow range of values for each day. Note that in , we are concerned with the constancy of the dilution factor for a single day's test; the absolute magnitude of the dilution factor can change day to day.
FIG. 5 The dilution ratio is plotted versus the time-ordered test number for the data collected on each of the three days. The letters next to the symbols indicate points where repeat measurements were taken for nominally identical undiluted particle concentrations. The dashed lines are least squares regression lines assuming a linear drift.
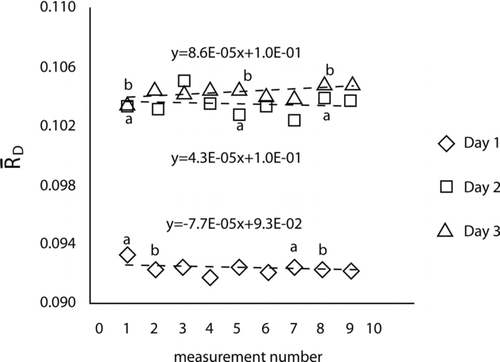
It is also of interest to assess whether there is a drift in the dilution ratio as a function of time. We have replotted the results as a function of measurement number, which is roughly proportional to time, as shown in . A total of nine dilution measurements were taken on each day. The letters next to the symbols in indicate the points where repeat measurements were taken for nominally identical undiluted particle concentrations. It is seen that there is little variation in the values for points a and b. More quantitative assessments of drift were also carried out. One approach was to compute the best linear fit to each day's data. The fact that the slopes of these lines are nearly zero, and no other clear systematic variation is visible in the data, indicates that there is no significant drift in the dilution ratios throughout the day. The regression results were further quantified using a statistical test based on the t-distribution. The t-statistics for the test of the slope each day versus a hypothesized value of zero were observed to be t = −1.56, t = −0.35, and t = 0.93. The fact that the absolute values of the t-statistic are smaller than the critical value, t (95%) = 2.36 based on seven degrees of freedom, indicates that none of these slopes are significantly different from zero.
The average and standard deviation of the mean of all measurements taken in a single day were also calculated for each of the three days of testing. These values give an estimate of the constancy of the dilution factor over a range of concentrations for each day. The square root of the average of the three relative variances, with a value of 0.0022 corresponding to 0.22%, is used as the typical standard uncertainty associated with the dilution testing.
The proportionality test indicates that the proportional model for the CPC is valid with a relative standard uncertainty of 0.22% over this concentration range, when used with the specified sample times, flow correction, and coincidence correction. and show that within the measurement uncertainty, there is no concentration-dependent bias of the dilution factor measurement, and that there is no bias from drift in this data. This also verifies that the coincidence correction factor accurately accounts for coincidence effects for concentrations up to 13,000 particles·cm−3.
Another way to assess the effect of the coincidence correction is to compare the dilution ratios computed with and without the coincidence correction for a single day of measurements. At concentrations below 103 particles·cm−3, the dilution ratios are nearly identical as indicated in . As the concentration increases above 103 particles·cm−3, the dilution ratios for the data with no coincidence corrections applied increase, thus revealing a deviation from proportionality in the CPC. The increase in the dilution ratio is due to the undercounting of the high concentration measured by the dummy CPC, caused by coincidence. This graph indicates that the dilution process described in this article is capable of detecting a deviation from proportionality in the CPC response.
5.2. Absolute Calibration
An NIST traceable calibration of the CPC using an AE was carried out for the Army Primary Standards Laboratory (Fletcher et al. Citation2009) over the concentration range from about 300 to 13,000 particles·cm−3. We make use of this calibration data for establishing a traceable CPC over the concentration range from 1 particle·cm−3 to 13,000 particles·cm−3. The dilution experiments quantify the proportionality of the CPC over this concentration range. The traceable calibration is needed to obtain an accurate proportionality constant for the relationship between the CPC concentration and the NIST traceable concentration measured by the AE, CAE . To determine the proportionality constant, we compute the ratio of CAE to CCPC for the six sets of data at the largest concentrations in the range from about 9500 to almost 13,000 particles·cm−3. The largest concentrations are used to minimize the effect of a possible systematic bias in the AE at low concentrations. The values used are shown in . The mean value of the proportionality constant, Cpro , and the standard deviation of the mean are 1.0592 ± 0.00098. The residuals from a proportional fit to the data in are plotted in , and they show an even distribution about zero.
TABLE 1 Data from the AE and CPC comparison at high concentrations, along with the coefficient of proportionality computed as the ratio of the AE to CPC concentration
FIG. 7 Residuals from the proportional fit to the data from the AE and CPC comparison at high concentrations.
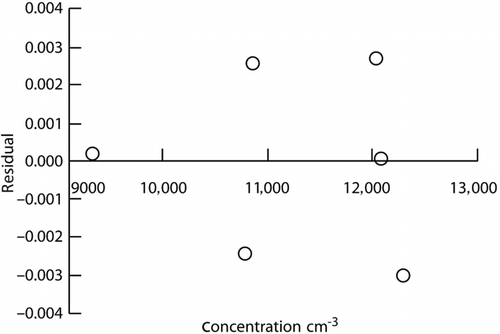
The concentrations measured in the primary calibration were computed with a standard volumetric flow rate referenced to 101,325 Pa and 25°C. The following expression based on the ideal gas law relates the concentrations based on the actual volumetric flow rate to the concentration referenced to standard conditions:
In EquationEquation (4), C, P, and T are the observed concentration, pressure, and absolute temperature, while Cref , Pref , and Tref are the reference values.
5.3. Uncertainty Analysis
The measurement uncertainty of the proportionality constant consists of two main components: the uncertainty in the proportionality measurement and the uncertainty in the primary calibration with the AE. The uncertainty in the proportionality measurement consists of the uncertainty from the constancy of the dilution ratio RD , which is computed to be 0.0022. This value was computed as the square root of the average of the relative variances for the three days.
TABLE 2 Uncertainty components’ analysis for CPC proportionality calibration
The relative uncertainty in the primary calibration, u 1(Cpro ), is computed as the root-sum-of-squares (RSS) of the relative uncertainty based on the standard deviation of the mean of the values of Cpro from , computed to be 0.00093, and the relative standard uncertainty in the AE measurement of particle concentration that is estimated to be 0.013 for the high concentration range of 12,000 from Fletcher et al. (Citation2009). This gives a final value for the relative standard uncertainty from the primary calibration of u 1(Cpro ) to be 0.01303.
The total uncertainty in the proportionality constant is computed as the RSS of the relative uncertainty in the proportionality measurement, 0.0022, and the relative uncertainty from the absolute calibration, 0.01303. The propagation of uncertainty values results in a combined standard uncertainty of uc (Cpro ) = 0.014. The corresponding relative expanded uncertainty is U = 0.028, computed using a coverage factor of k = 2. The uncertainty components are shown in .
6. CONCLUSIONS
This work has developed a method to calibrate a CPC over a wide range of concentrations with traceability to SI units as realized by NIST by using a stable diluter to perform an independent proportionality calibration of the CPC, along with an AE to measure the coefficient of proportionality. It has been shown that the CPC tested in this study is proportional over four orders of magnitude, provided that the measured concentrations are properly corrected for flow and coincidence. The relative expanded uncertainty of the CPC with a coverage factor of k = 2 is 2.8% over this range. These calibrations enable the CPC to perform accurate measurements of concentration with assigned uncertainties as low as 1 particle·cm−3 compared with a concentration of about 103 particles·cm−3 with the AE calibration alone, and provide a solution for laboratories that require traceable calibration of CPCs at low concentrations. The authors believe that this is an economical and analytically simple approach to calibrating a CPC at low concentrations, as well as providing an accurate estimate of the uncertainty that is required to establish measurement traceability.
The proportionality measurement is shown to be capable of detecting deviations from proportionality in a CPC using a diluter with an unknown dilution factor, as long as the diluter is proven to have a constant dilution factor independent of time and particle concentration. The nonlinearity errors expected from the CPC are mainly due to the coincidence effect, which contains an exponential term, and can be identified using the process described in this article, as shown in . The ability of the coincidence correction to restore the CPC to a proportional response is directly tested using this calibration process. However, the proportionality measurement using a diluter with an unknown dilution factor cannot detect nonlinearity resulting from a power law relationship between the measured and the true concentration. To detect this type of relationship, a diluter with a calibrated dilution factor must be employed in a measurement process similar to that described in this article. Based on the operating principle of the CPC with the single particle counting at low concentrations, it is highly unlikely that a power law relationship would occur over the entire range of the detector.
Finally, this calibration method illustrates and accounts for the fact that the CPC is proportional within a stated level of uncertainty. The designation of a CPC as nonlinear is a question for the CPC user to decide based on how accurate he or she needs their measurement to be. If the uncertainty in the CPC's proportional response is greater than the user's requirements, then additional corrections could be applied. One approach would be to consider a quadratic dependence of the true concentration on the measured concentration, such as done by Li et al. (Citation2004) in their study of nonlinearity in optical sensors.
Acknowledgments
The authors would like to thank the US Air Force Primary Standards Laboratory in Heath, Ohio, for sponsoring this project.
Notes
Certain commercial equipment, instruments, or materials are identified here in order to specify the experimental procedure adequately. Such identification is not intended to imply recommendation or endorsement by the NIST, nor is it intended to imply that the materials or equipment identified are necessarily the best available for the purpose.
REFERENCES
- Brockmann , J. G. , Lui , B. Y. H. and McMurry , P. H. 1984 . A Sample Extraction Diluter for Ultrafine Aerosol Sampling . Aerosol Sci. Technol. , 3 ( 4 ) : 441 – 451 .
- Fletcher , R. A. , Mulholland , G. W. , Winchester , M. R. , King , R. L. and Klinedinst , D. B. 2009 . Calibration of a Condensation Particle Counter Using a NIST Traceable Method . Aerosol Sci. Technol. , 43 : 425 – 441 .
- Giechaskiel , B. , Wang , X. , Horn , H.-G. , Spielvogel , J. , Gerhart , C. , Southgate , J. , Jing , L. , Kasper , M. , Drossinos , Y. and Krasenbrink , A. 2009 . Calibration of Condensation Particle Counters for Legislated Vehicle Number Emission Measurements . Aerosol Sci. Technol. , 43 : 1164 – 1173 .
- Hueglin , C. , Scherrer , L. and Burtscher , H. 1997 . An Accurate, Continuously Adjustable Dilution System (1:10 to 1:104) for Submicron Aerosols . J. Aerosol Sci. , 28 : 1049 – 1055 .
- Lewis , K. C. , Dohmeier , D. M. , Jorgenson , J. W. , Kaufman , S. L. , Zarrin , F. and Dorman , F. D. 1994 . Electrospray-Condensation Particle Counter: A Molecule-Counting LC Detector for Macromolecules . J. Anal. Chem. , 66 : 2285 – 2292 .
- Li , X. , Scott , T. , Yang , S. , Cromer , C. and Dowell , M. 2004 . Nonlinearity Measurements of High-Power Laser Detectors at NIST . J. Res. Natl. Inst. Stand. Technol., , 109 : 429 – 434 .
- NIST . 2009 . NIST Policy on Traceability Retrieved from http://www.nist.gov/traceability/
- Taylor , B. N. and Kuyatt , C. E. 1994 . Guidelines for Evaluating and Expressing the Uncertainty of the NIST Measurement Results. NIST Technical Note 1297 , Washington , DC : US Government Printing Office .
- Wang , X. , Caldow , R. , Sem , G. J. , Hama , N. and Sakurai , H. 2010 . Evaluation of a Condensation Particle Counter for Vehicle Emission Measurement: Experimental Procedure and Effects of Calibration Aerosol Material . J. Aerosol Sci. , 41 ( 3 ) : 306 – 318 .
- Yli-Ojanpera , J. , Makela , J. , Marjamaki , M. , Rostedt , A. and Keskinen , J. 2010 . Towards Traceable Particle Number Concentration Standard: Single Charged Aerosol Reference (SCAR . J. Aerosol Sci. , 41 : 719 – 728 .