Abstract
Organic carbon (OC) and elemental carbon (EC) are operationally defined due to the lack of definitive standards. Consequently, their quantification is protocol dependent. IMPROVE and NIOSH are the two widely used thermal/optical protocols for OCEC analysis, differing in temperature programs and in the optical method for charring correction. The IMPROVE protocol is often implemented on a DRI analyzer while the NIOSH protocol is often implemented on a Sunset Laboratory Analyzer. Evaluation of the implementation of the IMPROVE protocol on the Sunset Laboratory analyzer or implementation of the NIOSH or NIOSH-derived protocols on the DRI analyzer has rarely been reported. We analyzed OC and EC in about 100 ambient samples collected in the Pearl River Delta in China by implementing the IMPROVE protocol and a NIOSH-derived (ACE-Asia) protocol on both a DRI Model 2001 analyzer and a Sunset Laboratory analyzer. The total carbon (TC) and EC filter loading as determined by the ACE-Asia protocol on the Sunset analyzer varied from 2.6 to 67.0 and 0.2 to 7.4 μg cm−2, respectively. Inter-instrument comparison indicates that the implementation of the IMPROVE protocol on the Sunset analyzer reports TC, EC, and OC measurements to be in good agreement with those made on the DRI analyzer. EC and OC analyzed using the ACE-Asia protocol are also in good agreements for measurements implemented on the Sunset and DRI analyzers. Inter-protocol comparison indicates consistency in TC determination but discrepancies in OC and EC, with the IMPROVE protocol reporting much higher EC than the ACE-Asia protocol. An analysis of different comparison scenarios reveals that the cause of the EC difference could be quantitatively attributed to temperature protocol (thermal effect) and optical pyrolysis correction method (reflectance vs. transmittance). The variation in EC concentrations was more pronounced in samples that produced more charred OC during thermal analysis.
Copyright 2012 American Association for Aerosol Research
1. INTRODUCTION
Carbon in atmospheric aerosols can be divided into three categories: organic carbon (OC), elemental carbon (EC), and carbonate carbon (CC). CC is negligible in fine particles for most regions except those under the influence of mineral dust (e.g., CitationCao et al. 2005). OC and EC are among the major constituents of atmospheric aerosols and their quantification is necessary in understanding the role of aerosols in issues varying from health effects of aerosols to regional visibility degradation and to regional and global climate change (CitationHansen et al. 2005; CitationAndreae and Gelencser 2006). EC is particularly important in understanding how aerosols interact with various components of the climate system due to its strong light absorption capability. A recent study (CitationWu et al. 2009) found a relatively low (∼0.8) single scattering albedo (SSA) across the Pearl River Delta (PRD), implying the abundant black carbon concentration in this region.
Despite their importance, there are not definitive standards for their quantification. Thermal/optical methods are widely used to determine OC and EC in ambient and source aerosols in the atmospheric measurement field (CitationWatson et al. 2005; CitationChow, Yu, et al. 2007). It is also widely recognized that OC and EC are operationally defined by analysis methods, in which analysis temperature programs and optical charring correction means are important factors influencing the concentrations of OC and EC determined. Among the thermal/optical methods, IMPROVE (CitationChow et al. 1993) and NIOSH (CitationBirch and Cary 1996) are two mainstream protocols for OCEC analysis around the world. The two protocols differ in temperature programs and in the optical mean for charring correction.
Total carbon (TC) measurements by the two protocols agree well, within ±10% (CitationChow et al. 2005), but EC concentration was found to differ 2∼10 times when the two protocols were implemented on the DRI analyzer for about 40 ambient samples (Mexico urban and US urban and nonurban samples) and 19 source samples (CitationChow et al. 2001). More recent comparisons of the two protocols on other data sets also consistently report that EC and OC by NIOSH and IMPROVE protocols are not comparable (CitationChow et al. 2005; CitationCheng et al. 2011; CitationZhi et al. 2011). The NIOSH protocol only outlines the necessary principles for operation, rather than specifies detailed parameters at each temperature step [e.g., residence time (RT)]. Therefore, a number of NIOSH-derived protocols existed in the literature. As summarized by CitationWatson et al. (2005), the temperature program in the NIOSH protocol has been modified by various research groups in their analysis to determine aerosol EC and OC. The modified NIOSH protocols typically differ slightly from the original NIOSH method in the temperature and duration of the temperature steps during the analysis stage in inert helium (He) carrier gas. A few examples of the modified NIOSH protocols are the STN (Speciation Trends Network in the United States; Peterson and Richards 2002), the ACE-Asia (CitationSchauer et al. 2003), HKUST-3 (CitationYang and Yu 2002), and HKGL (CitationSin et al. 2002) protocols.
In practice, the two protocols are often implemented on two different analyzers, that is, the IMPROVE protocol is implemented on a DRI/OGC analyzer while the NIOSH protocol is implemented on a Sunset Laboratory Analyzer (Tigard, OR). Recently, a newer DRI analyzer (Model 2001a, Atmoslytic Inc., Calabasas, CA) replaced the old DRI/OGC analyzer for carbon analysis in the IMPROVE network. The default protocol implemented on DRI 2001 analyzers is a modified IMPROVE protocol, termed IMPROVE_A protocol. The temperatures in the IMPROVE_A protocol differ from those in the IMPROVE protocol because a new hardware in the DRI 2001 analyzer represents the sample temperature better than does the old hardware. Consequently, IMPROVE_A protocol temperatures match more closely the sample temperatures experienced in the analysis using the IMPROVE protocol on the previous models of DRI analyzers. Chow, Watson, et al. (2007) have shown that comparable OC, EC, and TC are obtained using either the IMPROVE or the IMPROVE_A temperature protocol with an optical reflectance correction for sample charring. They also observed that the two temperature protocols yield different, but highly correlated, results for thermally resolved carbon fractions. In our current work, we have implemented the IMPROVE temperature protocol for comparison with a NIOSH-derived protocol. Both the DRI analyzer and the Sunset analyzer in its recent model provide filter transmittance and reflectance monitoring via a laser throughout the analysis; however, they differ in their instrument designs as to the arrangement of the laser relative to the filter.
In the United States, the PM2.5 STN has shifted its carbon analysis protocol from NIOSH to IMPROVE_A since 2007 (CitationChow, Watson, et al. 2007). In Hong Kong, the NIOSH protocol implemented on Sunset analyzers has been used as the method to determine OC and EC in PM10 in the city's air quality monitoring network since 1998 (CitationSin et al. 2002; CitationYu et al. 2004). In China, the National Technical Committee on Meteorological Disaster Prevention and Mitigation of Standardization Administration of China has recently been discussing to adopt IMPROVE_A as the standard method to determine OC and EC in ambient aerosols (National Technical Committee on Meteorological Disaster Prevention and Mitigation of Standardization Administration of China 2010). These developments in carbon analysis methods have prompted the necessity of evaluating the following two issues: (1) whether the IMPROVE protocol implemented on a Sunset analyzer would give comparable EC and OC to those determined by implementing the IMPROVE protocol on a DRI analyzer and (2) whether the NIOSH or NIOSH-derived protocol implemented on a DRI analyzer would give comparable EC and OC to those determined by implementing the NIOSH protocol on a Sunset analyzer. Previous protocol comparison studies were conducted either on a single analyzer, e.g., comparison of the NIOSH and IMPROVE protocols on the DRI analyzers (CitationChow et al. 2001, Citation2004, Citation2005; CitationZhi et al. 2009) or between the DRI analyzer implementing the IMPROVE protocol and the Sunset analyzer implementing the NIOSH protocol (CitationChow et al. 2005; CitationCheng et al. 2011; CitationZhi et al. 2011). The instrument dependence of EC and OC measurements using the same protocols has yet to be investigated.
FIG. 1 Schematic diagrams of arrangements of quartz tube, sample boat, and monitoring laser for the Sunset analyzer (left) and the DRI Model 2001 analyzer (right). (Color figure available online.)
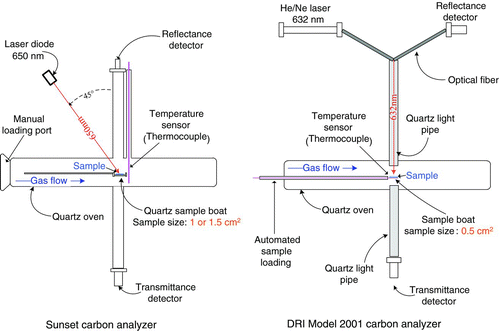
Another objective of this study is to investigate, through inter-protocol comparisons, how variations in OC and EC reported by the two mainstream protocols are related to differences in temperature programs and in optical charring correction.
2. EXPERIMENTAL SECTION
2.1. Sample Description
The samples used in this study were PM2.5 samples collected in multiple locations in the PRD, China, a region of 54,744 km2 that includes several megacities such as Hong Kong, Guangzhou, and Shenzhen. Each sample was collected on prebaked quartz fiber filter for 24 h from midnight to midnight. A total of 61 samples were collected in Nansha, a suburban district of Guangzhou, following a schedule of one sample for every 6 days over a period of 1 year from July 2007 to August 2008. These samples were collected using one of two mid-volume PM2.5 samplers (RAAS, Andersen Instruments, Smyrna, GA and SASS, Met One Instruments, Grants Pass, OR). The two mid-volume samples were deployed during different sampling periods. Another 40 samples were collected at four locations in Hong Kong in November and December 2010, also following a 6-day cycle schedule. These 40 samples were sampled by a high-volume (hi-vol) sampler (Tisch Environmental, Cleves, OH) at a flow rate of 1.13 m3 min−1. The four sampling locations include a roadside site, two urban sites, and a suburban site. After sample collection, the 47 mm quartz filters (Pallflex, Tissuquartz, 2500-QAT-UP) used in the mid-volume samplers were stored in a Petri dish lined with prebaked aluminum foil to reduce contamination and under –20°C to minimize the loss of volatile species. The 20 × 25 cm filters (Pallflex, Tissuquartz, 2500-QAT-UP) from the hi-vol samplers were folded and wrapped in prebaked aluminum foil before storing in the freezer.
TABLE 1 Temperature programs of the IMPROVE and ACE-Asia protocols
2.2. Carbon Analysis
One DRI carbon analyzer (Model 2001; CitationChow et al. 1993) and one Sunset carbon analyzer (CitationBirch and Cary 1996) were used to determine EC and OC in this work. The schematic diagrams of the arrangements of the quartz oven and the laser beam in the two analyzers shown in indicate the relative position between the laser and the filter. Both the Sunset and the DRI analyzers are capable of simultaneously monitoring laser transmittance and reflectance signal for charring correction. The major difference in their optical configurations is that the laser source in the DRI analyzer is installed coaxially with transmittance and reflectance detectors, while in the Sunset design there is a ∼45° angle between the laser source and the two detectors. Another difference is the pathway by which laser travels from source to detector. In the Sunset analyzer, laser goes through the wall of quartz oven before reaching the filter sample and then the reflectance and transmittance detectors. In the DRI analyzer, laser travels in optical fiber outside the oven and is guided by a light pipe when traveling inside the quartz oven.
For carbon analysis on the Sunset analyzer, a filter punch of 1.0 cm2 in size was removed from each filter sample for analysis. On the DRI analyzer, a filter punch of 0.5 cm2 was used. The two protocols adopted for analysis are the ACE-Asia (a NIOSH-derived protocol) and IMPROVE protocols. The ACE-Asia protocol was used for OCEC analysis during the ACE-Asia campaign (CitationSchauer et al. 2003). It is the same as quartz.par method that is preloaded in the Sunset OCEC analyzer. An analysis of the 61 Nansha samples was conducted using both the ACE-Asia and the IMPROVE protocols and on both the Sunset and the DRI analyzers. A total of 244 analysis results were obtained in four combinations of temperature programs and optical charring correction for the 61 Nansha samples on each analyzer. The four combinations are IMPROVE_TOR, IMPROVE_TOT, ACE-Asia_TOT, and ACE-Asia_TOR, where IMPROVE and ACE-Asia refer to the temperature programs while TOR and TOT refer to charring correction using reflectance and transmittance, respectively. The 40 Hong Kong samples were analyzed on the Sunset analyzer using both the IMPROVE and the ACE-Asia protocols but their analyses on the DRI analyzer were only carried out using the IMPROVE_TOR protocol.
The detailed temperature programs of the ACE-Asia and IMPROVE protocols are shown in . The major differences between the two protocols are the following. (1) The maximum temperature in the He stage reaches 870°C in the ACE-Asia protocol versus 550°C in the IMPROVE protocol. Different amounts of charred OC are formed as a result. (2) The ACE-Asia_TOT protocol utilizes transmittance signal while the IMPVOE_TOR protocol adopts reflectance signal for pyrolysis carbon correction. Charred OC and EC have different penetration depths on filter (CitationChow et al. 2004). Filter transmittance and reflectance apparently would exhibit different sensitivities to charred OC and EC on the filter. (3) In the ACE-Asia protocol, the RT is fixed for each temperature step, the duration of which is typically shorter than that needed to return flame ionization detector (FID) signal to baseline. In the IMPROVE protocol, the RT of each temperature step is variable and its duration is driven by FID signal slope to ensure the full isolation of carbon fraction in that temperature step. In the implementation of the IMPROVE protocol, the Sunset analyzer determines the FID baseline at the beginning of the analysis. When the FID reading during a temperature step returns to the initial FID baseline, this temperature step ends and the analysis moves to the next temperature step. As a result, the analysis time of the IMPROVE protocol is more than double that of the ACE-Asia protocol. shows two typical thermograms of a sample obtained with the ACE-Asia and IMPROVE protocols on the Sunset analyzer. The thermograms on the DRI analyzer are very similar and shown in Figure S1 (see supplemental information).
FIG. 2 Example thermograms of an ambient sample using the IMPROVE and ACE-Asia protocols. Temperature: Oven temperature during analysis; Laser T: laser transmittance signal; Laser R: laser reflectance signal; T split: OC/EC split by laser transmittance signal; R split: OC/EC split by laser reflectance signal; FID: flame ionization detector signal. (Color figure available online.)
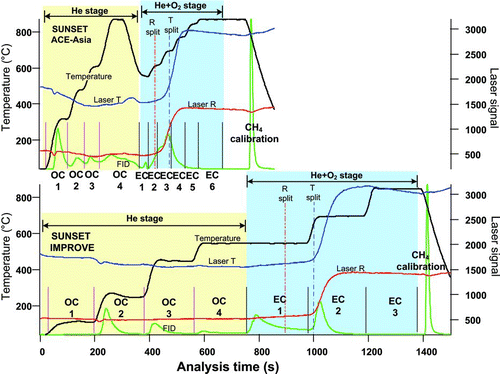
Before the analysis of each batch of samples, the instruments were calibrated in the range of 4–90 μgC with sucrose solutions (Sigma-Aldrich, St. Louis, MO) for the DRI analyzer and with the National Institute of Standards and Technology (NIST) standard reference material SRM1649a for the Sunset analyzer. The sucrose standard concentration was calibrated with SRM1649a. Analysis results (TC, OC, and EC) are calculated using programs supplied by the instrument manufacturers. Precisions on both carbon analyzers determined by replicate analysis of aerosol-laden filters were better than 5% for TC, OC, and EC by the IMPROVE_TOR method and better than 5% for TC and OC and better than 8% for EC by the ACE-Asia_TOT method (Figure S2, online supplemental information). Field blank samples were obtained at the end of every month. In the inter-instrument comparison study, data are corrected for field blank before comparison.
3. RESULTS AND DISCUSSION
Linear regression analyses of the various carbon data in this study were performed using Deming regression, instead of least-squares regression method. The ordinary least-squares regression method assumes that x values are error free, which induces incorrect slope estimation (CitationChu 2005). In comparison, Deming regression (CitationDeming 1943) accounts for both errors in y- and x-axes. Hence, it was adopted in this article for all the correlation analysis.
3.1. Inter-Instrument and Inter-Protocol Comparison of TC Measurements
The TC loading in the 61 Nansha samples covers a wide range (4–70 μg cm−2). Nansha, located in a suburban district of Guangzhou, is a receptor site year around, impacted by pollution transported from upwind urban areas and having little locally generated emissions. Under the influence of predominant winds, Nansha is downwind urban Guangzhou in fall and winter and downwind Hong Kong in spring. PM2.5 in Nansha, collected over the period of a year, could represent a diverse range of aerosols in this region. The TC measurements of the 61 Nansha samples reported by the two instruments agree well for both the ACE-Asia and the IMPROVE protocols, which is an expected result and consistent with previous comparison studies (CitationChow et al. 2005). As shown in , high correlation coefficients (R 2 > 0.99) and near-unity slopes were found for both protocols (1.06 for both the IMPROVE protocol and the ACE-Asia protocol). TC deviations between the two protocols as a function of filter loading are shown in . Most samples fall in the range of ±20%, confirming good agreement in TC measurements between the two analyzers. Increasing TC deviations, but still within ±25%, were observed in the lower filter loading samples as these levels are closer to the minimum detection limit. The results suggest that both instruments are robust on reporting equivalent TC with both the ACE-Asia and the IMPROVE protocols.
FIG. 3 Inter-instrument comparison (DRI Model 2001 vs. Sunset) of TC. The format for regression line is y = ax + b; p is the p value for paired t-test. (a) TC concentrations by the DRI Model 2001 analyzer vs. by the Sunset analyzer. (Open squares are TC data obtained with the IMPROVE protocol; open circles are TC data obtained with the ACE-Asia protocol.) (b) Deviations in TC determined on the two analyzers. (Color figure available online.)
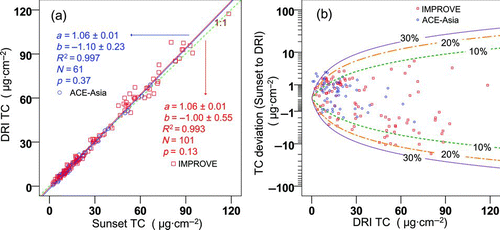
Inter-protocol TC comparison shows good agreement as well, as shown in . Unity slopes (1.02 on the DRI analyzer and 0.97 on the Sunset analyzer) and high correlation (R 2 > 0.99) obtained from this comparison are similar to those reported in a previous study (CitationChow et al. 2001). Deviations in TC (±10%) are small on both the DRI and the Sunset analyzers (), indicating that TC equivalence by the ACE-Asia and IMPROVE protocols is independent of instrument.
FIG. 4 Inter-protocol TC comparison (ACE-Asia vs. IMPROVE). The format for regression line is y = ax + b; p is the p value for paired t-test. (a) TC concentrations by the two protocols on the Sunset analyzer (open circles) and on the DRI Model 2001 analyzer (open squares). (b) Deviations in TC by the two protocols. (Color figure available online.)
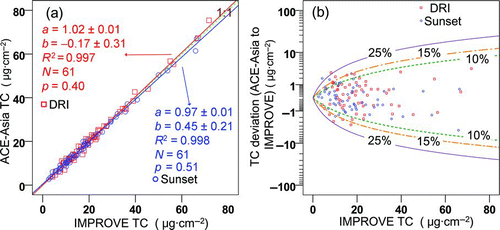
3.2. IMPROVE_TOR Protocol: Inter-Instrument Comparison of EC and OC Measurements
The combined data sets of the 61 Nansha samples and the 40 Hong Kong samples were used in inter-instrument comparison of the IMPROVE protocol. High correlations of IMPROVE_TOR OC and EC (R 2 = 0.99) were observed between measurements made by the two different analyzers (). The regression slopes of IMPROVE_TOR measurements by the two analyzers (Sunset vs. DRI) were 0.92 ± 0.01 for OC and 1.05 ± 0.02 for EC. Both values are close to unity, indicating good agreement between the two instruments. Concentration deviations for most samples are within ±25% for OC () and ±20% for EC (). These results demonstrated that implementing the IMPROVE_TOR protocol on the Sunset analyzer produced OC and EC measurements equivalent to those made using the IMPROVE_TOR protocol on the DRI analyzer.
FIG. 5 Comparison of IMPROVE_TOR OC and EC measurements by the Sunset and DRI Model 2001 analyzers. The samples for comparison include 61 mid-volume samples collected in Nansha and 40 high-volume samples collected at four sites in Hong Kong. The format for regression line is y = ax + b; p is the p value for paired t-test. (a) Deming regression plot of inter-instrument comparison of IMPROVE_TOR OC concentrations. (b) Deviations in IMPROVE_TOR OC measurements by the two analyzers. (c) Deming regression plot of inter-instrument comparison of IMPROVE_TOR EC concentrations. (d) Deviations in IMPROVE_TOR EC concentrations by the two analyzers. (Color figure available online.)
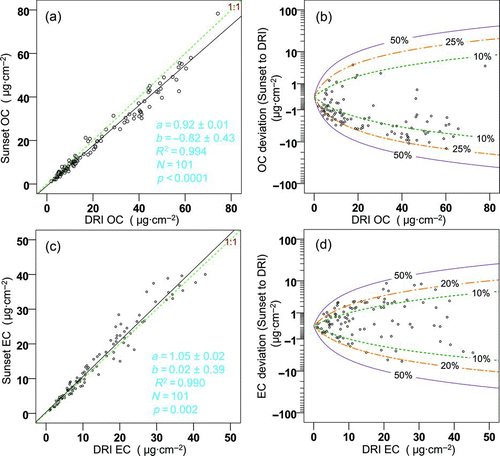
3.3. ACE-Asia_TOT Protocol: Inter-Instrument Comparison of EC and OC Measurements
Slopes close to unity (1.04 for OC and 0.97 for EC) were obtained between measurements made on the two analyzers ( and c), indicating equivalent determination of OC and EC with the ACE-Asia protocol on the two instruments. High correlations were also found, with R 2 = 0.99 for OC and R 2 = 0.93 for EC. OC deviations were mostly within ±10% but larger deviations in EC (±20%) were observed ( and d). Four EC data pairs (marked in the circle in ) were judged to be outliners and excluded in the regression analysis. A close examination of raw data revealed that the laser signal had a minimum value of less than 20 during the analysis of these samples on the DRI analyzer, approaching the lower end of the laser transmittance signal range (0–2500) reported by the analyzer. The DRI analyzer assigns an uncertainty value of 10 with its transmittance measurement. As a result, a greater uncertainty was expected in relying on transmittance for OC and EC demarcation for the low laser samples. The low laser problem only occurred when implementing the ACE-Asia_TOT protocol on the DRI analyzer. This is likely due to more charring formed in the analysis using the ACE-Asia_TOT protocol as a result of the higher maximum temperature in the analysis stage in the He carrier gas.
FIG. 6 Comparison of ACE-Asia_TOT OC and EC measurements by the Sunset and DRI Model 2001 analyzers. The format for regression line is y = ax + b; p is the p value for paired t-test. (a) Deming regression plot of inter-instrument comparison of ACE-Asia_TOT OC concentrations. (b) Deviations in ACE-Asia_TOT OC measurements by the two analyzers. (c) Deming regression plot of inter-instrument comparison of ACE-Asia_TOT EC concentrations (open circles represent normal samples, while open squares represent low laser samples). The low laser samples were excluded in the regression analysis. (d) Deviations in ACE-Asia_TOT EC concentrations by the two analyzers. (Color figure available online.)
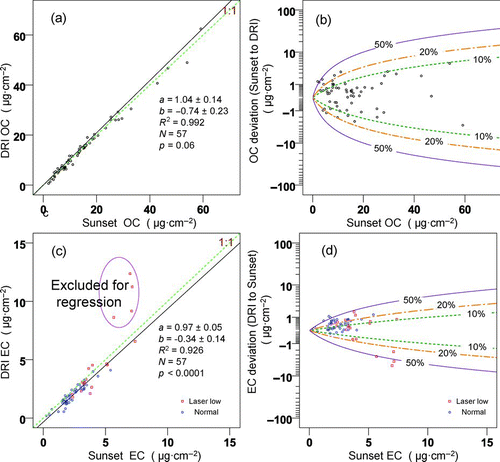
3.4. Comparison of EC and OC by IMPROVE_TOR and ACE-Asia_TOT Protocols
Owing to the different temperature evolution and laser correction method, OC and EC reported by IMPROVE_TOR and NIOSH_TOT are not comparable (CitationChow et al. 2001). Our inter-protocol comparison also confirmed this conclusion as shown in . OC reported by the IMPROVE_TOR is on average 0.56 times the OC by ACE-Asia_TOT on the Sunset analyzer and 0.62 times on the DRI analyzer. EC reported by IMPROVE_TOR is higher than ACE-Asia_TOT as shown in , with a slope of 5.42 on the Sunset and 4.31 on the DRI analyzer. This EC ratio between the IMPROVE_TOR and the NISOH_TOT is not only dependent on analyzer, but also dependent on sample location and sample composition because of the spatiotemporally varying nature of ambient aerosol. The slope obtained in this study is within the range reported by previous studies, including 2∼6 by CitationChow et al. (2001), ∼3.4 by CitationChow et al. (2004), and 1.6 by CitationZhi et al. (2009).
FIG. 7 Inter-protocol comparisons of OC and EC measurements. The format for regression line is y = ax + b. (a) Comparison of ACE-Asia_TOT OC and IMPROVE_TOR OC on the Sunset analyzer (open circles) and on the DRI 2001 analyzer (open squares). (b) Comparison of ACE-Asia_TOT EC and IMPROVE_TOR EC on the Sunset analyzer (open circles) and on the DRI Model 2001 analyzer (open squares). (Color figure available online.)
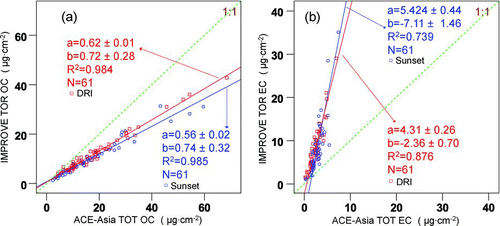
FIG. 8 Equivalence of ACE-Asia OC4 + AEC and IMPROVE AEC. The format for regression line is y = ax + b. (a) Deming regression plots of ACE-Asia OC4 + AEC vs. IMPROVE AEC on the Sunset analyzer (open squares) and on the DRI Model 2001 analyzer (open circles). (b) Deviations between ACE-Asia OC4 + AEC vs. IMPROVE AEC. (Color figure available online.)
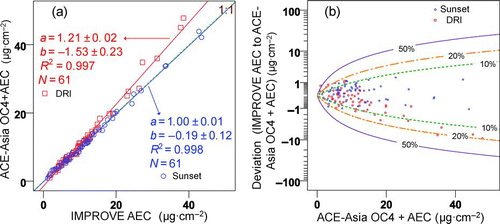
3.5. Discussion on EC Comparison
Potential causes for the difference in EC concentrations determined by the ACE-Asia_TOT and IMPROVE_TOR protocols include the thermal factor (different temperature steps) and laser correction factor (transmittance vs. reflectance). The main differences in the thermal factor between the two protocols are related to the maximum temperature in He stage (T max_He) and RT of each temperature plateau. The T max_He affects not only the allocation of the carbon fraction that is liberated in the He stage in the ACE-Asia protocol or in the He/O2 stage in IMPROVE, but also the amount of char formed in the He stage in both the protocols. RT affects char formation as a longer RT in the first few temperature steps could reduce pyrolysis (Yu et al. Citation2002). CitationZhi et al. (2009) found that the effect of T max_He is more important than RT on the measured EC.
CitationChow et al. (2001) found that OC4 in the NIOSH protocol was approximately equivalent to the difference between EC by the two protocols. This conclusion is confirmed by our comparison for samples collected in the PRD, China (). A comparison was made between ACE-Asia OC4 + AEC (apparent EC, sum of all EC fractions that evolve after the carrier gas was switched to the O2/He mixture) and IMPROVE AEC. Clear equivalence relations can be found from the close-to-unity slope values and high R 2 values (0.99) on both instruments (). Small differences (±15%) between these two quantities, as shown in , also confirmed that this agreement is instrument independent. However, we need to note that the relative proportions of pyrolyzed OC and native EC (the EC that is originally present in the sample before the thermal analysis) in the two equivalent quantities are dependent on the analysis temperature programs. They are different between two analyses of the same sample obtained with different temperature programs.
Different laser correction methods are envisioned to result in different OC/EC split as laser reflectance and transmittance respond to different parts of pyrolyzed OC and EC on the filter. CitationChow et al. (2004) revealed that char penetrated deeper into quartz fiber filters than EC and that liberation of char and EC by oxidation is from surface to deeper part of the filter. As transmittance signal accounts for EC and char throughout the entire filter while reflectance signal only accounts for EC and char on the filter surface, the reflectance signal would return to the initial value earlier than the transmittance signal, which leads to earlier OC/EC split by the reflectance method as shown in . CitationChen et al. (2004) did a model study using K-M theory and concluded that the diversity of char and EC absorption efficiencies further contributes to the variation in EC reported by different laser correction methods.
TABLE 2 Deming regression results of EC determined using different combinations of temperature program and charring correction
FIG. 9 Comparisons of EC measurements on the Sunset analyzer by different combinations of temperature programs and charring correction methods. The format for regression line is y = ax + b. (a) EC by the IMPROVE temperature program vs. EC by the ACE-Asia temperature program. Open circles are data derived from charring correction by reflectance and open squares are data derived from charring correction by transmittance. (b) EC derived from reflectance-based vs. transmittance-based charring correction. Open circles are measurements using the IMPROVE temperature program and open squares are measurements using the ACE-Asia temperature program. (Color figure available online.)
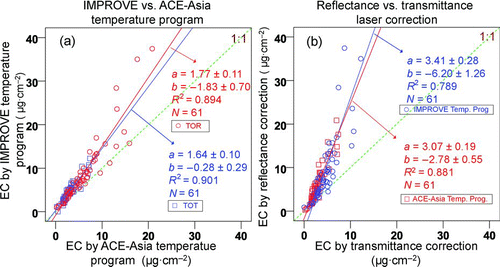
For the exploration of the effects of temperature steps and the laser correction and the instrument setup, four scenarios of different combinations of these factors are compared for each analyzer. They include: (1) ACE-Asia_TOT, (2) ACE-Asia_TOR, (3) IMPROVE_TOT, and (4) IMPROVE_TOR. Correlations of EC measurements between different pairs of analysis scenarios are summarized in .
For the investigation of the thermal effect, pairs of analysis scenarios with the same laser correction method but different temperature programs are compared in for data obtained on the Sunset analyzer. With TOR charring correction, EC by the IMPROVE temperature program was ∼1.77 times the EC by the ACE-Asia temperature program. This result is different from that reported by CitationChow et al. (2004), which found equivalency of TOR EC by the IMPROVE and NIOSH protocols. Further study is needed to investigate whether reflectance correction is independent of temperature protocol in thermal/optical analysis. With TOT charring correction, EC by the IMPROVE temperature program was ∼1.64 times the EC by the ACE-Asia temperature program. It can be interpreted that the difference between the IMPROVE and the ACE-Asia temperature programs caused a factor of ∼1.7 (average of 1.77 and 1.64) difference on EC concentration, regardless of whether TOT or TOR was used for charring correction. Data obtained on the DRI analyzer also found similar thermal factors between the two temperature programs (1.65 for reflectance-based measurements and 1.62 for transmittance-based measurements; ).
The effect of laser correction for charring on EC results is examined in for measurements made on the Sunset analyzer. The plot clearly shows that the reflectance-based EC measurements are higher than the transmittance-based EC measurements by a factor of 3.07 (IMPROVE temperature program) and 3.41 (ACE-Asia temperature program). Similar laser correction effects were also found on the DRI analyzer, i.e., 2.60 for measurements using the IMPROVE temperature program and 3.36 for measurements using the ACE-Asia temperature program. CitationChow et al. (2004) also examined the laser correction factor using EC data by the IMPROVE and NISOH temperature programs obtained on a DRI analyzer. They found that the reflectance-based EC measurements were 1.4 times the transmittance-based EC measurements using the IMPROVE temperature program and the factor was 3.3–5.0 using the NIOSH temperature protocol. In our samples, the variability in EC determined by reflectance (ECTOR) versus transmittance (ECTOT) was more pronounced for samples that produced more charred OC during the analysis. The difference between ECTOR and ECTOT was roughly proportional to the amount of charred OC (). This observation confirms the suggestion by CitationChen et al. (2004) that the EC bias caused by charring correction methods was larger for samples having higher ratios of charred OC/EC. A recent study in Europe (CitationMaenhaut et al. 2011) also found that the ratio of EC by IMPROVE_TOR to EC by NIOSH_TOT increased in samples significantly influenced by wood burning, which are expected to have more char formation during analysis.
FIG. 10 Difference in EC as determined using filter reflectance vs. transmittance as a function of the amount of charred OC. (a) EC determined using the ACE-Asia temperature program. (b) EC determined using the IMPROVE temperature program. (Color figure available online.)
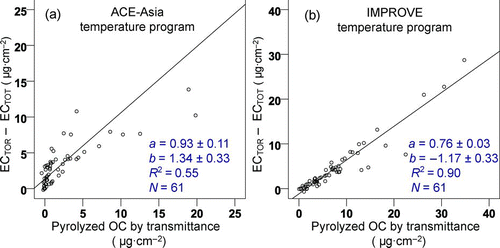
We further found that the variation between EC by IMPROVE_TOR and ACE-Asia_TOT can be quantitatively described to be the combined impact of charring optical correction and temperature program. On the Sunset analyzer, the multiplication of the thermal effect factor (1.71) and the laser correction factor (3.24) yields a value of 5.54, very close to the regression slope value of 5.42 between EC data obtained with the IMPROVE_TOR and ACE-Asia_TOT protocols (Table S1). Similarly, on the DRI analyzer, the product of the two factors is 4.89, also close to the regression slope value of 4.31 between EC data obtained with the IMPROVE_TOR and ACE-Asia_TOT protocols.
4. CONCLUSIONS
For historical reasons, the IMPROVE_TOR protocol is often implemented on DRI carbon analyzers while the NIOSH_TOT protocol is often implemented on Sunset analyzers. In this work, we have demonstrated that EC and OC determined by implementing the IMPROVE_TOR protocol on a Sunset carbon analyzer are equivalent to those obtained with a DRI 2001 carbon analyzer implementing the IMPROVE_TOR protocol. We also showed that the ACE-Asia-TOT protocol, a NIOSH-derived protocol, implemented on a DRI analyzer reports equivalent EC and OC concentrations to those obtained with a Sunset analyzer. In other words, despite the difference in their instrument design in monitoring filter transmittance and reflectance, the two types of analyzers are capable of reporting equivalent EC and OC when using the same analysis protocol.
Inter-protocol comparison of OC and EC indicates that measurements by the IMPROVE_TOR and ACE-Asia_TOT protocols are not comparable. The same conclusion was reached in previous comparison studies by other researchers. On the Sunset analyzer, EC concentrations reported by the IMPROVE_TOR method were on average 5.4 times than those by the ACE-Asia_TOT protocol for about 100 ambient samples collected in the PRD, China. The cause of the EC difference can be quantitatively attributed to differences in temperature programs and in optical charring correction methods, with the latter playing a more important role. The difference in EC by the two protocols was further found to be more prominent in samples that produced more charring during the analysis. This implies that the variation in EC concentrations as determined by the two commonly adopted protocols is sample dependent and that there is not a universal factor to convert EC determined by one protocol to that by the other protocol.
uast_a_649313_sup_23323621.zip
Download Zip (157.1 KB)Acknowledgments
[Supplementary materials are available for this article. Please go to the publisher's online edition of Aerosol Science and Technology for the free supplementary files.]
This work was partially supported by HKUST Fok Ying Tung Graduate School, Natural Science Foundation of China (U0733004 and 40775011), China National Basic Research and Development Program-2011CB403403, and NSFC-GD Joint Funding Key Project (U1033001). We thank Guangzhou Institute of Tropical and Marine Meteorology for providing the DRI analyzer and logistic support at its Panyu field station. Three anonymous reviewers are gratefully acknowledged for their constructive comments.
REFERENCES
- Andreae , M. O. and Gelencser , A. 2006 . Black Carbon or Brown Carbon? The Nature of Light-Absorbing Carbonaceous Aerosols . Atmos. Chem. Phys. , 6 : 3131 – 3148 .
- Birch , M. E. and Cary , R. A. 1996 . Elemental Carbon-Based Method for Monitoring Occupational Exposures to Particulate Diesel Exhaust . Aerosol Sci. Technol. , 25 : 221 – 241 .
- Cao , J. J. , Lee , S. C. , Zhang , X. Y. , Chow , J. C. , An , Z. S. Ho , K. F. 2005 . Characterization of Airborne Carbonate over a Site near Asian Dust Source Regions During Spring 2002 and Its Climatic and Environmental Significance . J. Geophys. Res. , 110 : D03203
- Chen , L. W. A. , Chow , J. C. , Watson , J. G. , Moosmuller , H. and Arnott , W. P. 2004 . Modeling Reflectance and Transmittance of Quartz-Fiber Filter Samples Containing Elemental Carbon Particles: Implications for Thermal/Optical Analysis . J. Aerosol Sci. , 35 : 765 – 780 .
- Cheng , Y. A. , He , K. B. , Duan , F. K. , Zheng , M. , Du , Z. Y. Ma , Y. L. 2011 . Ambient Organic Carbon to Elemental Carbon Ratios: Influences of the Measurement Methods and Implications . Atmos. Environ. , 45 : 2060 – 2066 .
- Chow , J. C. , Watson , J. G. , Chen , L. W. A. , Arnott , W. P. and Moosmuller , H. 2004 . Equivalence of Elemental Carbon by Thermal/Optical Reflectance and Transmittance with Different Temperature Protocols . Environ. Sci. Technol. , 38 : 4414 – 4422 .
- Chow , J. C. , Watson , J. G. , Chen , L. W. A. , Chang , M. C. O. , Robinson , N. F. Trimble , D. 2007 . The IMPROVE-A Temperature Protocol for Thermal/Optical Carbon Analysis: Maintaining Consistency with a Long-Term Database . J. Air Waste Manage. Assoc. , 57 : 1014 – 1023 .
- Chow , J. C. , Watson , J. G. , Crow , D. , Lowenthal , D. H. and Merrifield , T. 2001 . Comparison of IMPROVE and NIOSH Carbon Measurements . Aerosol Sci. Technol. , 34 : 23 – 34 .
- Chow , J. C. , Watson , J. G. , Louie , P. K. K. , Chen , L. W. A. and Sin , D. 2005 . Comparison of PM2.5 Carbon Measurement Methods in Hong Kong, China . Environ. Pollut. , 137 : 334 – 344 .
- Chow , J. C. , Watson , J. G. , Pritchett , L. C. , Pierson , W. R. , Frazier , C. A. and Purcell , R. G. 1993 . The Dri Thermal Optical Reflectance Carbon Analysis System—Description, Evaluation and Applications in United States Air-Quality Studies . Atmos. Environ. A Gen. , 27 : 1185 – 1201 .
- Chow , J. C. , Yu , J. Z. , Watson , J. G. , Ho , S. S. H. , Bohannan , T. L. Hays , M. D. 2007 . The Application of Thermal Methods for Determining Chemical Composition of Carbonaceous Aerosols: A Review . J. Environ. Sci. Health, Part A , 42 : 1521 – 1541 .
- Chu , S. H. 2005 . Stable Estimate of Primary OC/EC Ratios in the EC Tracer Method . Atmos. Environ. , 39 : 1383 – 1392 .
- Deming , W. E. 1943 . Statistical Adjustment of Data , New York : Wiley .
- Hansen , J. , Sato , M. , Ruedy , R. , Nazarenko , L. , Lacis , A. Schmidt , G. A. 2005 . Efficacy of Climate Forcings . J. Geophys. Res. , 110 : D18104
- Maenhaut , W. , Claeys , M. , Vercauteren , J. and Roekens , E. 2011 . “ Comparison of Reflectance and Transmission in EC/OC Measurements of Filter Samples from Flanders, Belgium ” . In Proceedings of the 10th International Conference on Carbonaceous Particles in the Atmosphere Vienna
- National Technical Committee on Meteorological Disaster Prevention and Mitigation of Standardization Administration of China . 2010 . “ Technical standard of Aerosol Carbon Analysis (draft) ” . http://sac345.org.cn/upload/userfiles/tfx.pdf
- Peterson , M. R. and Richards , M. H. 2002 . “ Thermal-Optical-Transmittance Analysis for Organic, Elemental, Carbonate, Total Carbon, and OCX2 in PM2.5 by the EPA/NIOSH Method ” . In Symposium on Air Quality Measurement Methods and Technology , Edited by: Winegar , E. D. and Tropp , R. J. Pittsburgh , PA : Air and Waste Management Association . 83-1–83-19
- Schauer , J. J. , Mader , B. T. , Deminter , J. T. , Heidemann , G. , Bae , M. S. Seinfeld , J. H. 2003 . ACE-Asia Intercomparison of a Thermal-Optical Method for the Determination of Particle-Phase Organic and Elemental Carbon . Environ. Sci. Technol. , 37 : 993 – 1001 .
- Sin , D. W. M. , Fung , W. H. and Lam , C. H. 2002 . Measurement of Carbonaceous Aerosols: Validation and Comparison of a Solvent Extraction-Gas Chromatographic Method and a Thermal Optical Transmittance Method . Analyst , 127 : 614 – 622 .
- Watson , J. G. , Chow , J. C. and Chen , L. -W. A. 2005 . Summary of Organic and Elemental Carbon/Black Carbon Analysis Methods and Intercomparisons . Aerosol Air Qual. Res. , 5 : 65 – 102 .
- Wu , D. , Mao , J. T. , Deng , X. J. , Tie , X. X. , Zhang , Y. H. Zeng , L. M. 2009 . Black Carbon Aerosols and Their Radiative Properties in the Pearl River Delta Region . Sci. China Ser. D Earth Sci. , 52 : 1152 – 1163 .
- Yang , H. and Yu , J. Z. 2002 . Uncertainties in Charring Correction in the Analysis of Elemental and Organic Carbon in Atmospheric Particles by Thermal/Optical Methods . Environ. Sci. Technol. , 36 : 5199 – 5204 .
- Yu , J. Z. , Tung , J. W. T. , Wu , A. W. M. , Lau , A. K. H. , Louie , P. K. K. and Fung , J. C. H. 2004 . Abundance and Seasonal Characteristics of Elemental and Organic Carbon in Hong Kong PM10 . Atmos. Environ. , 38 : 1511 – 1521 .
- Yu , J. Z. , Xu , J. H. and Yang , H. 2002 . Charring Characteristics of Atmospheric Organic Particulate Matter in Thermal Analysis . Environ. Sci. Technol. , 36 : 754 – 761 .
- Zhi , G. R. , Chen , Y. J. , Sheng , G. Y. and Fu , J. M. 2009 . Effects of Temperature Parameters on Thermal-Optical Analysis of Organic and Elemental Carbon in Aerosol . Environ. Monit. Assess. , 154 : 253 – 261 .
- Zhi , G. R. , Chen , Y. J. , Sun , J. Y. , Chen , L. G. , Tian , W. J. Duan , J. C. 2011 . Harmonizing Aerosol Carbon Measurements Between Two Conventional Thermal/Optical Analysis Methods . Environ. Sci. Technol. , 45 : 2902 – 2908 .