Abstract
Incense burning is a common ritual in Asian communities both indoors in residential homes and outdoors in temple premises. Organic particles from burning of incense sticks, incense coils, and mosquito coils after extensive dilution (>1000×) were characterized by the Aerodyne high-resolution time-of-flight aerosol mass spectrometer (HR-ToF-AMS). The obtained mass spectra in general resemble those reported for biomass burning aerosols. Ion peaks with m/z values higher than 100 accounted for 15%–25% of the organic signals in the unit-mass-resolution (UMR) mass spectra. In the high-resolution (HR) mass spectra, the ion peaks at m/z 60 and 73 are found to be related to the sugar anhydrides as in particles from other biomass burning processes. In addition, the ion peaks at m/z 107, 121, 137, 151, 167, and 181, some of which (e.g., m/z 137 and 167) have been observed in particles from biomass burning but not yet assigned, were assigned to lignin-related components. Elemental analysis from the HR data reveals that a large portion of particulate organics from incense burning are oxygenated (O/C between 0.3 and 0.5) and unsaturated (and/or cyclic) in nature. Results from this study also highlight that mass spectra from HR-ToF-AMS measurements concerning primary emissions such as incense burning contain very useful information in the high m/z (>100) region about the chemical characteristics of those primary organic particles.
Copyright 2012 American Association for Aerosol Research
1. INTRODUCTION
Incense burning in temples (Lung and Kao Citation2003) and churches (Weber Citation2006), as well as in residential homes (Cheng et al. Citation1995), are common, especially in Asian communities. In addition, burning herbal incense is a traditional and efficient antimosquito measure that is still widely practiced in tropical and subtropical regions (Liu et al. Citation2003). Due to the potential health risks (Chiang et al. Citation2009), research on the physical and chemical properties of particles from incense burning is of growing interest (Wang et al. Citation2006; See et al. Citation2007).
The sizes of particles from incense burning are typically at the submicron level, making them easily inhalable (Chang et al. Citation2007). Particle concentrations can increase by a factor of up to 15–50 by mass or a factor of 2–5 by number in common house environments (Ji et al. Citation2010). Although the contribution of incense burning to indoor air pollution has been stressed in many studies, incense burning as a pollutant source is an activity in both indoor and outdoor environments. In fact, thousands of tons of incense are burned in temples annually in Taiwan alone (Lin et al. Citation2008; Hu et al. Citation2009), most of which are actually burned outdoors in the temple yards. There are a few studies concerning the outdoor pollutant concentration near busy temples showing that the PM2.5 concentration can reach 100–200 μg/m3 in the vicinity of typical Asian temples (Lin et al. Citation2002; Wang et al. Citation2007). In a recent field study in Hong Kong (Sang et al. Citation2011), elevated biomass burning marker (levoglucosan) concentrations were observed and attributed to the incense and joss paper burning during the traditional Ching Ming festival when worshipping to ancestors was widely practiced (largely outdoors) in this region.
The chemical composition of particles from incense burning is highly complex because they are a mixture of numerous pyrolysis products from equally complex biomass substrates. These substrates can be wood as the incense material, bamboo to act as the stick on which the incense material is coated in the case of an incense stick (IS) or “joss stick,” and herbs as the material for antimosquito incense. Like particles from other biomass burning processes, particles from incense burning are organic-rich thermal degradation products of bio-molecules, including cellulose, hemicellulose, lignin, etc. It is therefore expected that the organic particles from incense burning resemble those from biomass burning [known as biomass burning organic aerosol (BBOA)], even though smoldering is normally the only burning process in incense burning. In fact, the similarity between particles from incense burning and those from other types of biomass burning has been investigated recently (Tsai et al. Citation2010). Detailed chemical speciation of inorganic ions, carboxylic acids, anhydrosugars, and sugar alcohols was provided for particles from the burning of two types of sandalwood-based incense. However, these four categories of compounds only accounted for 5% of the total mass of the particles (Tsai et al. Citation2010), with the remaining constituents being most likely other organic matters (OM). Another study (Wang et al. Citation2006) also showed that organic carbon (OC) comprised over 50%, while elemental carbon (EC) generally comprised <10%, of the PM2.5 mass from incense burning, which essentially is a smoldering process. But little is known about this large portion of organic content, e.g. their functionality, oxidation state, etc., despite these detailed off-line chemical analyses.
The Aerodyne aerosol mass spectrometer (AMS) has been shown to be very useful in studying the bulk chemical composition of BBOA (Schneider et al. Citation2006; Alfarra et al. Citation2007; Weimer et al. Citation2008; Mohr et al. Citation2009; He et al. Citation2010) including more recently incense burning particles (Ji et al. Citation2010). With this online mass spectrometric technique, Schneider et al. (Citation2006) proposed that the ion peaks at m/z 60 and 73 are from sugar anhydrides such as levoglucosan, which is an important tracer of BBOA (Simoneit et al. Citation1999; Simoneit Citation2002), and that they can serve as marker fragments for BBOA. Some other abundant ion peaks at higher m/z values such as 137, 167, and 181 were also observed but not assigned. With similar observation of these marker fragments (m/z 60, 73, 137, etc.), Alfarra et al. (Citation2007) further proved the usefulness of the ion-specific approach, using m/z 60 alone, for quantifying wood burning contributions with higher time resolution than approaches using off-line quantification of levoglucosan. Weimer et al. (Citation2008) investigated the signals of such marker fragments by burning different types of woods and concluded that the differences in the mass spectra are mainly due to different burning phases (flaming versus smoldering) rather than wood type. More recently, He et al. (Citation2010) used a high-resolution time-of-flight AMS (HR-ToF-AMS) to study the organic particles from biomass burning and Chinese style cooking and suggested that the differences in mass spectral features can be used to differentiate the sources.
Ji et al. (Citation2010) investigated particles from incense burning using a compact time-of-flight AMS (c-ToF-AMS). It was concluded that C n H+ 2n−1 and C n H+ 2n+1 homologous ion series are the major contributors to the organic mass spectra, with the former more abundant than the latter. In this first AMS study on incense burning, the unit-mass-resolution (UMR) mass spectral analysis, combined with a number of particle characterization techniques such as SMPS (scanning mobility particle sizer), OPC (optical particle counters), and TEOM (tapered element oscillating microbalance), provided a real-world evaluation of exposure to incense smoke in a typical residential environment. Yet, more detailed chemical features such as functionality and oxidation state are still rare. The development of the HR-ToF-AMS, which has the capability of obtaining more chemical information about the particles than does the UMR AMS, provides a new tool to enhance our understanding of the chemical properties of particles from incense burning.
The current study aims at investigating the bulk chemical properties of particles from the burning of three types of incense—incense stick (IS), incense coil (IC), and mosquito coil (MC). The particulate organic composition was characterized using an HR-ToF-AMS with a mass resolving power of approximately 4000 (DeCarlo et al. Citation2006) in the W mode. We focused on three groups of ion peaks that have relatively high intensities—homologous ion series, sugar- and aromatic-related ions, and lignin-related ions. UMR mass spectra from the V mode were analyzed to quantify the contributions of those three groups of ions to the organic signals. High-resolution (HR) mass spectra from the W mode were analyzed to identify the molecular formula of several ions with high m/z values. By comparing with the mass spectra of other BBOA and analyzing HR mass spectra, two features of particles from incense burning—unsaturation/cyclization and oxygenation—are revealed.
2. EXPERIMENTAL
2.1. Burning Experiments
Burning of IS, IC, and MC was conducted in a 30-L burning chamber that coupled to a two-stage dilution setup, as shown in Figure S1. The door of the burning chamber is properly sealed when closed by applying rubber resilient sealing tapes around the edges. The first stage diluter (Dekati, Finland) has a dilution ratio of 20. The second dilution setup is a home-made pressure differential dilutor, with one arm open to allow the flow of HEPA (high-efficiency particulate air) filtered air and the other connected to a coil with an inner diameter of 1/16 in, which is smaller than the 1/4 in diameter of most of other tubing. The second dilution setup gives a dilution ratio of 60–80. The flows from these two arms are merged and directed to a mixing tube before going to dryer and the AMS. The two-stage dilution setup gave a total dilution ratio of 1200–1600, which is a factor of 2–3 of the highest dilution ratios (∼510) used in a study of diesel exhaust and wood smoke (Lipsky and Robinson Citation2006). Lipsky and Robinson (Citation2006) suggested that a dilution ratio of 5000 is needed for sources such as diesel exhaust and wood smoke to give mass concentrations at ambient level, which indicates that our dilution process is between typical laboratory experiments (normally <500) and extensive partitioning/mixing processes in outdoor environments (∼5000). The total flow rate through the setup (∼7 L/min) and the chamber volume (30 L) corresponds to an air-exchange-rate of ∼14 h−1, which is at the higher end (0.5–16 h−1) of a well-ventilated indoor environment (Johnson et al. Citation2004) that approaches the outdoor dilution process. Upon extensive dilution, re-partitioning occurred (Lipsky and Robinson Citation2006) and semivolatile organics in the particles from incense burning evaporated to the gas phase, thereby particulate OM discussed in this study are those that remained in the particle phase, as those could be found far away from the sources such as in outdoor environments.
After ignition, the incense materials were placed inside the chamber immediately. In each experiment, three ISs were burned and the burning lasted for about 30 min. A portion of an IC or an MC was burned for about 60 min. Because of the high concentration of particles produced during the burning experiments, the small tubing (1/16 in) in the second dilution setup (see Figure S1) clogged up in some experiments, which led to abnormal concentration profiles in some experiments. Therefore, three burning experiments with good flow control and similar concentration profiles for each substrate were chosen for further analysis. The chosen regions had much higher organic aerosol mass concentrations (>10 μg/m3) than the background (<1 μg/m3).
2.2. HR-ToF-AMS Measurement
The particles passing through the dilution setups were directed to the Aerodyne HR-ToF-AMS for analysis. The detailed description of the HR-ToF-AMS can be found in DeCarlo et al. (Citation2006). Briefly, the sampling flow rate was controlled to be ∼1.3 cm3/s by a critical orifice and particles passed through an aerodynamic lens for particle focusing. Then the particles traveled in the vacuum particle time-of-flight (pToF) chamber before being flash-vaporized on the heater (600°C). The vaporized compounds were then ionized by electron ionization (EI) at a voltage of 70 eV. The ionized species entered the ion extraction region and then the ion time-of-flight (iToF) chamber, which serves as a mass analyzer. The ToF mass analyzer was operated under two modes (V and W) which provide different traveling distances for the ions, resulting in different mass resolving power (approximately 2000 and 4000, respectively) (DeCarlo et al. Citation2006). The ions were then detected by a multichannel plate (MCP) detector.
2.3. Data Processing
Data from the HR-ToF-AMS were analyzed by Igor-based software packages SQUIRREL (version 1.51H) and PIKA (version 1.10H). The ionization efficiency (IE) of the HR-ToF-AMS was calibrated with size-selected (350 nm in mobility diameter) ammonium nitrate particles. Based on two previous studies that compared fresh biomass burning particle mass concentrations measured by AMS with those measured by other instruments (Alfarra et al. Citation2007; Weimer et al. Citation2008), a collection efficiency (CE) of 0.7 was used. Any uncertainty associated with the choice of CE would affect the absolute concentrations of particulate components but will not affect the mass spectral features discussed later. Elemental analysis (EA) was also performed using the tool kit embedded in PIKA following the methods and calibration factors described previously (Aiken et al. Citation2007, Citation2008). Modification to the fragmentation table is needed and is described later and in the online supplemental information (Section 2).
2.4. Modification to the Fragmentation Table
The AMS signal at m/z 28 is overwhelmingly dominated by N+ 2 from the large air beam signal, therefore the assignment of signals at this m/z value to organic content needs careful consideration (Grieshop et al. Citation2009). The same is true for m/z 18 due to the uncertain contribution from organic dehydration fragments (Grieshop et al. Citation2009), which is complicated by gas-phase as well as particle-phase water. Contributions from organics to these two ions are both related to m/z 44, a commonly observed oxygenated organic ion (CO+ 2), in the fragmentation table correction during AMS data analysis. There are two versions of coefficients in assigning the signals of m/z 18 and 28 to organic compounds as related to m/z 44. In the old version (hereinafter as frag_18/28_v1), m/z 18 is set to equal to m/z 44, and m/z 28 to 0 (assuming all m/z 28 is from N+ 2) (Allan et al. Citation2004). In the updated version (hereinafter as frag_18/28_v2), the coefficients of m/z 18 versus 44 and m/z 28 versus 44 are set to be 0.225 and 1, respectively (Aiken et al. Citation2008). The same coefficients are extended to the HR fragmentation table for H2O+ versus CO+ 2 and CO+ versus CO+ 2, respectively (Aiken et al. Citation2008).
Both versions of assignments have been found suitable in capturing the average contributions of these two ions to the organic mass in a large number of data points, such as those in field campaigns with thousands of spectra. However, caution was raised in a few latest laboratory studies regarding the assignment of these ions. For example, Grieshop et al. (Citation2009) found that for fresh biomass burning aerosols, the contribution of m/z 18 to the organic mass was in the same order of magnitude as that of m/z 44 (close to frag_18/28_v1), while m/z 28 was four to ten times of m/z 44. In a chamber study of secondary organic aerosols (SOA), Chen et al. (Citation2011) also suggested very different coefficients of m/z 18 versus 44 (0.6 to 2.7) and m/z 28 versus 44 (1.1 to 2.6) from the default values (either frag_18/28_v1 or frag_18/28_v2). Also those coefficients seemed to have a slight dependence on the precursors (Chen et al. Citation2011).
In this study, we adopted frag_18/28_v1 in the UMR analysis to facilitate comparison of measured mass spectra with those from other BBOA in the AMS mass spectra database (http://cires.colorado.edu/jimenez-group/AMSsd/), most of which used frag_18/28_v1. As discussed in the online supplemental information (Section 2), we noticed that both frag_18/28_v1 and frag_18/28_v2 seem to underestimate the contributions of these ions to organic mass spectra. Therefore, we develop a modified fragmentation table (hereinafter as frag_18/28_v_i), which is based on the ion correlation and pToF data analysis of our measurements. In the HR analysis and subsequent EA, we compared the application of frag_18/28_v2 (Aiken et al. Citation2007, Citation2008) and frag_18/28_v_i. The details of frag_18/28_v_i are given in the online supplemental information (see Section 2, Figures S2–S4, and Tables S1–S3).
3. RESULTS AND DISCUSSION
The organic mass concentrations of several burning experiments performed for IS, IC, and MC are shown in Figure S5. The organic mass concentrations were between 50 and 350 μg/m3 even after a two-stage dilution, with the MC having the highest organic mass concentrations. Organic components dominated in the ultrafine (PM1) nonrefractory particulate mass as measured by the HR-ToF-AMS, in agreement with a previous study on incense burning (Wang et al. Citation2006) showing that over 50% of PM2.5 mass were due to OC mass. By comparison, Lee and Wang (Citation2006) found that on an average mass concentration basis for PM2.5, burning MCs also generated more particles (2.39–4.95 mg/m3) than burning traditional Chinese ISs (0.4–2.7 mg/m3). Furthermore, it was shown that inorganic (NH4 +, NO3 −, SO4 2−, etc.) concentrations were less than 5% of the total particle mass (PM1 as measured by AMS) from the burning of different types of woods (Weimer et al. Citation2008) and other materials (Mohr et al. Citation2009), which is also the case in our experiments.
TABLE 1 Signal contribution (%) and possible ion formula in unit-mass-resolution (UMR) mass spectra of organic particles from the burning of IS, IC, and MC
3.1. Unit Mass Resolution Mass Spectra
shows the UMR mass spectra obtained in the V mode for the burning of the three substrates, using frag_18/28_v1. Three groups of ions, homologous ion series (group I), sugar- and aromatics-related ions (group II), and lignin-related ions (group III), are highlighted for further discussion because of their relatively high intensities. Group I contains ions of m/z 27, 29, 41, 43, 55, and 57, with two adjacent members of the homologous series separated by a CH2 (m/z 14) unit. The first four ion peaks (27, 29, 41, and 43) have usually been observed to have high intensities in a number of studies on primary organic aerosols (POA), including those from biomass burning (Schneider et al. Citation2006; Alfarra et al. Citation2007; Weimer et al. Citation2008), plastic/paper burning (Mohr et al. Citation2009), cooking (Mohr et al. Citation2009; He et al. Citation2010), as well as vehicular exhaust (Schneider et al. Citation2006; Mohr et al. Citation2009). In general agreement with the mass spectral results from wood burning (Schneider et al. Citation2006; Weimer et al. Citation2008), the ion peaks at m/z 29 and 43 were the highest peaks in our incense burning experiments. Note that this is the case only when frag_18/28_v1 is used here in UMR analysis. If modified coefficients (frag_18/28_v_i) that relate m/z 18 and 28 to m/z 44 are used, m/z 29 and 43 are not the highest peaks (see below). Group II contains ions of m/z 60, 73, 77, 85, and 91. Group III contains some ion peaks of relatively high intensities with m/z values larger than 100. Some of these peaks (e.g., m/z 137, 167, and 181) have also been observed in previous studies (Schneider et al. Citation2006; Alfarra et al. Citation2007; Weimer et al. Citation2008) on biomass burning, in which the origin of these ions remained to be assigned. It is worth noting that m/z 44, which is an important marker for oxidized organics in ambient organic aerosols and in organic aerosols from chamber studies concerning oxidative chemistry (Ng et al. Citation2010, Citation2011), is not included in the discussion in this study concerning nascent POA. In the region of m/z 44, the ion CO+ 2 dominates over C2H4O+ in many studies, as highlighted by Ng et al. (2010, 2011). So is the case in this study, as shown in Figures S3 and S4 that 70% of the signal in the region of m/z 44 is due to the ion CO+ 2. The rest 30% by C2H4O+ is a substantial fraction that is higher than those found in field and chamber studies. The relatively high contribution by C2H4O+ was also observed in another laboratory study concerning primary emission (Mohr et al. Citation2009).
FIG. 1 Average unit-mass-resolution (UMR) mass spectra of organic compounds in particles from burning three types of incense: incense stick (IS), incense coil (IC), and mosquito coil (MC). Three groups of ions, including homologous series (Group I), sugar- and aromatics-related ions (Group II), and lignin-related ions (Group III), are chosen for further analysis.
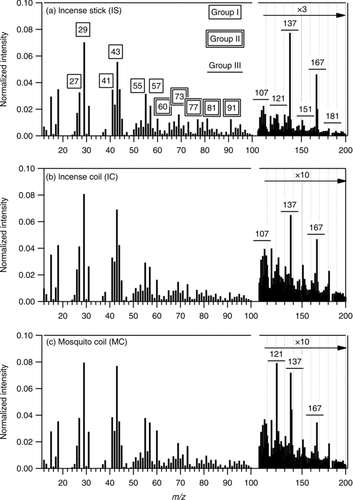
shows the signal percentages of ions in the three groups to the total organic signals based on frag_18/28_v1. The signals of ions in Group I contributed to 2% (for m/z 57) to 8% (for m/z 29) of the organic signals, and these 6 ion peaks altogether accounted for over 20% of the UMR organic signals. These high percentages again demonstrate the dominant contribution of organic signals from these homologous ion series. Ion peaks at m/z 60 and 73 are believed to be formed from the sugar anhydrides (e.g., levoglucosan) that have been used as tracers for particles from biomass burning (Simoneit et al. Citation1999; Simoneit Citation2002; Schneider et al. Citation2006), although there are recent concerns about the stability of levoglucosan in the atmosphere (Hoffmann et al. Citation2009; Hennigan et al. Citation2010; Kessler et al. Citation2010). Weimer et al. (Citation2008) reported the percentages of m/z 60 and 73 for the burning of a variety of woods in the smoldering phase to be 0.6%–4.1% and 0.1%–2.0%, respectively. The percentages of these two ions from our experiments (1.3%–2.8% for m/z 60 and 1.1%–1.7% for m/z 73) are comparable to these ranges, although the substrates burned were not exactly the same. Ion peaks at m/z 77, 91, and 81 are typical for mono-cyclic aromatics such as alkyl-substituted benzene (for m/z 77 and 91) and heterocyclic aromatics (for m/z 81) (McLafferty and Turecek Citation1993) and their contributions to the total signals are around 1%. In fact, ion peaks at m/z 77 and 91 were observed in other burning-related particles (Schneider et al. Citation2006) and ambient aerosols (Zhang et al. Citation2005), and hence they are not specific to biomass burning. The ion peaks of m/z 107, 121, 137, 151, 167, and 181 normally contribute less than 1% to the total signals, although the intensities of these ion peaks are relatively high within the region of m/z 100–200. Two exceptions are the peaks at m/z 137 and 167 for IS, which contribute 2.6% and 1.5% to the total signals, respectively. Note that m/z 167 can be due to phthalates (McLafferty and Turecek Citation1993) instead of organics from biomass burning. However, the HR analysis () has ruled out this possible interference in our case.
As shown in from our results, 15%–25% of organic signals are from ion peaks with m/z values from 100–300, with a substantial fraction in the region of 100–200. This observation indicates that the ion peaks with higher m/z values should not be ignored for both better representation of mass concentrations and valuable information on the molecular speciation. This seems to be more important for biomass-derived particles, which potentially produce high-molecular-weight organics (Engling et al. Citation2006; Lin et al. Citation2010) that might not fragment into small ions, than for those from other primary sources such as vehicular exhaust. He et al. (Citation2010) showed that 11%–13% of MS signals were from ion peaks with m/z values larger than 100 for particles from biomass burning, but only 5%–7% for particles from Chinese style cooking. In an ambient measurement in Mexico City, DeCarlo et al. (Citation2008) also noticed that ion peaks with higher m/z values (>120) contributed more to the signals when the region was affected by plumes from biomass burning.
FIG. 2 High-resolution (HR) mass spectra of lignin-related ions (Group III) from burning three types of incense. A peak in the region of m/z 107 (before C7H7O+), more obviously shown in panels (b) IC and (c) MC, should be C6H3O2 +. It is not included in the fitting here since its peak is much smaller than the others (C7H7O+ and C8H+ 11). At m/z 167, the ion C8H7O4 + can be due to phthalates (McLafferty and Turecek Citation1993) but the other ion (C9H11O3 +) is due to organics from incense burning and it dominates. (Color figure available online.)
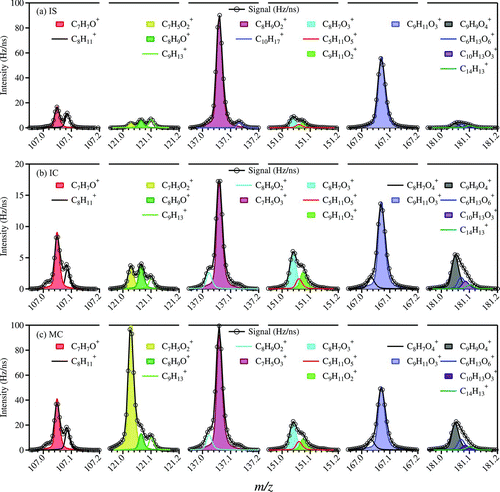
3.2. Comparison with Other BBOA Particles
We also compared the UMR mass spectra from our incense burning experiments with those from other BBOA by correlations of BBOA (including incense burning) spectra with three representative mass spectra of biomass nature. Mass spectra of biomass-derived organic particles from the AMS database (http://cires.colorado.edu/jimenez-group/AMSsd/), including the three representative mass spectra, were compiled. Three mass spectra, namely (1) levoglucosan (Schneider et al. Citation2006), (2) lignin powder (Kroll) (see http://cires.colorado.edu/jimenez-group/AMSsd/), and (3) positive matrix factorization (PMF)-resolved BBOA (Ng et al. Citation2011), were selected to represent the general mass spectral features of BBOA. Signals in all mass spectra were converted to normalized intensity as shown in to facilitate direct correlation with the three representative mass spectra. Ion peaks from m/z 12 to 300 of mass spectra from all substrates burned were correlated with three representative mass spectra to obtain the Pearson's r values and slopes. Ion peaks at m/z 16, 17, 18, and 28 were excluded for the correlation because they are related to m/z 44 in the fragmentation table. So was the ion peak at m/z 39 because in some, but not all, of the mass spectra from the database, it is attributed solely to the inorganic ion K+. Correlations of the mass spectrum from MC with the above three “standard” spectra are shown as examples in a–c. The comparison results (Pearson's r and slope) of all correlations are depicted in d–f, with reference to the mass spectra of levoglucosan, lignin powder, and PMF-resolved BBOA, respectively.
FIG. 3 Example of correlations: the mass spectrum from mosquito coil (MC) with mass spectra from (a) levoglucosan, (b) lignin powder, and (c) PMF-resolved BBOA. Pearson's r values and slopes in the correlations of mass spectra from different BBOA, including particles from incense burning, with those of (d) levoglucosan, (e) lignin powder, and (f) PMF-resolved BBOA.
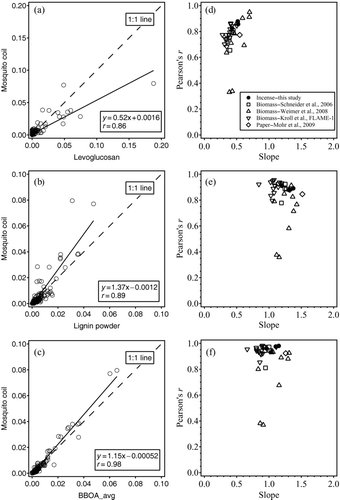
In the correlation with levoglucosan mass spectrum, the slopes for most substrates are significantly below unity. This comparison indicates that the mass spectrum of levoglucosan alone (as one single compound) does not represent the overall features of mass spectra of BBOA, despite the usefulness of m/z 60 and 73 as markers for BBOA. The correlation with the mass spectrum of lignin powders, however, gives fairly good figures of merit with both the Pearson's r values and the slopes being close to unity, with some exceptions for particles from smoldering biomass burning. In the correlation with the PMF-resolved BBOA mass spectrum, the Pearson's r values and slopes are similar to the correlation with the mass spectrum of lignin powder, with several outliers in the region of low Pearson's r values. These comparisons show that the mass spectra from laboratory-generated BBOA, including particles from our incense burning, correlate well with both mass spectra of lignin powder and PMF-resolved BBOA, suggesting that lignin powder captures the main mass spectral features of BBOA better than does levoglucosan.
3.3. High-Resolution Mass Spectra
The HR mass spectra of ions in Groups I and II are shown in the online supplemental information (Figures S6 and S7), with fitted ion peaks compared (see Section 4 in the online supplemental information) with those in the literature (Mohr et al. Citation2009; He et al. Citation2010), in which detailed description of these ions is available. One general feature is that the intensities of C n H+ 2n−1 ions are higher than those of C n H+ 2n+1 ions in the homologous series. In some UMR regions (e.g., m/z 29, 43, 55, 57, 69, 71, 83, and 85), however, intensities of oxygenated ions are similar to or even higher than those of C n H+ 2n−1 and C n H+ 2n+1 ions. He et al. (Citation2010) proposed that this feature, which resulted in higher intensities at m/z 29 and 43 than m/z 41 and 55, can be used to distinguish BBOA and Chinese cooking aerosols.
DeCarlo et al. (Citation2008) proposed that ions at higher m/z values could originate from molecules that have higher molecular weights and those that are resistant to EI fragmentation. The methoxyphenolic units from lignin in the biomass seem to fit this description. First, even the simplest monomeric unit of p-hydroxyphenyl (H) has a molecular mass higher than 100. Second, the delocalization effect of the aromatic ring helps retain the phenolic oxygen, the methoxy group(s), and the ring structure. On the other hand, lignin is an essential biopolymer in biomass (Simoneit Citation2002) and its abundance in biomass is second to cellulose (Boerjan et al. Citation2003). It has been widely observed that particles from biomass burning contain substantial amounts of lignin monomeric compounds and their derivatives (Simoneit Citation2002). Hence, it is expected that the mass spectra of particles from incense burning as well as those from other biomass burning have some features related to this important constituent in biomass-derived particles.
The HR mass spectra of ions in Group III are shown in . The shadowed peaks at m/z 107 and 121, 137 and 151, and 167 and 181 correspond to ions from the p-hydroxyphenyl (H), guaiacyl (G), and syringyl (S) units, respectively ( and ). Similar fragments have been observed in the mass spectra of lignin-related compounds using standard EI–MS (Kjallstrand et al. Citation1998), pyrolysis-GC (gas chromatography)/MS (Izumi and Kuroda Citation1997), and ToF-SIMS (Time-of-Flight Secondary Ion Mass Spectrometry) (Saito et al. Citation2005). For IS (a), the signals of m/z 137 and 167 were relatively high and were dominated by ions from the G and S units (C8H9O+ 2 and C9H11O+ 3, respectively). The same is true for IC (b), except that the contribution of the H unit ion (m/z 107, C7H7O+) was slightly higher than that for IS. For MC (c), the contributions of m/z 121 (C7H5O+ 2) of the H unit and m/z 137 (C8H9O+ 2) of the G unit were higher than those of the other lignin-related ions. The contributions from different lignin monomeric units to particles from biomass burning depend on the substrates in the biomass (Simoneit Citation2002 and references therein) and on the burning phase (flaming or smoldering). It has been shown that hardwoods contain more “heavy” monomeric units such as the S unit, while softwoods and grass contain more “light” ones such as the H unit (Boerjan et al. Citation2003). In terms of the substrates of the different types of incense that were burned in our experiments, IS and IC contain woody powder as the main ingredient, while IS has a bamboo stick to hold the powder coating. MC, however, mainly contains herbal materials. This is consistent with the observation that MC generates more H unit ions than do IS and IC.
FIG. 5 High-resolution mass spectra of particulate organics from burning (a) incense stick (IS), (b) incense coil (IC), and (c) mosquito coil (MC), using the modified fragmentation table (frag_18/28_v_i). The inserted pie charts on the left represent the percentages of hydrocarbon ions (CxHy), oxygenated ions (CxHyOz), and others (N- and S-containing compounds). See Section 3.3 for description of these three categories. The pie charts on the right represent the percentages of ions with molecular formula of C n H2n+1 (saturated) and C n H<2n+1 (unsaturated or cyclic). (Color figure available online.)
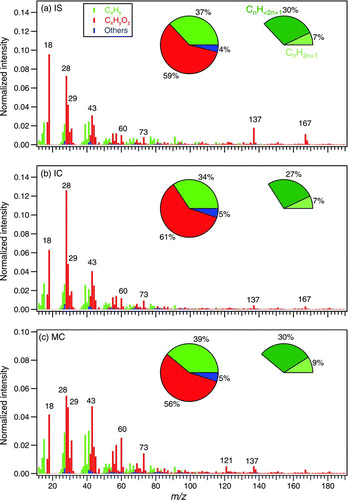
Using the modified HR fragmentation table (frag_18/28_v_i, see Section 2.4 and online supplemental information Section 2), we obtained HR mass spectra of organic particles from incense burning as shown in . The families of Cx and CH are grouped as the CxHy (hydrocarbon) category; the families of CHO1 (ions with only one oxygen atom), CHOgt1 (ions with more than one oxygen atoms), and HO are grouped as the CxHyOz (oxygenated) category; other N- and S-containing families are grouped as the “others” category. As mentioned above, when the modified fragmentation table is used, the highest peak is not m/z 29 or 43. Instead, m/z 18 (mainly H2O+) and 28 (mainly CO+) contribute substantially to the organic signals, indicating that the modification to the fragmentation table significantly affects the estimation of oxidation state of the organic particles. Other than these differences due to the use of different fragmentation tables, the mass spectra from V and W modes are comparable for the same substrate in terms of the relative intensity of ion signals in the integral mass region. In the inserted pie charts (left) in , it is shown that 56%–61% of the organic signals were contributed by the category of CxHyOz, suggesting that most of the organic moieties in the particles are oxygenated. The category of CxHy made up another 34%–39%. It is interesting to note that only 7%–9% out of this 34%–39% of the “hydrocarbon” ion signals (CxHy) were due to saturated hydrocarbon ions (C n H+ 2n+1). A majority (∼30%) of the “hydrocarbon” ion signals were due to ions of different degrees of unsaturation or cyclization (C n H+ <2n+1). Note that fragmentation of organic compounds involves a number of mechanisms such that it does not allow us to conclusively infer the exact degree of saturation or cyclization of the original compounds based on the fragments alone. To a large extent, however, most saturated aliphatic hydrocarbons do preserve their saturated nature in their most abundant fragments (Gross Citation2004). Hence it is not unreasonable to expect that the hydrocarbon fraction (not necessarily individual molecules, can also be hydrocarbon moiety) of organic compounds in the particles from incense burning is largely unsaturated or cyclic (or both) in nature. The abundance of unsaturated/cyclic moieties in OM from incense burning (and possibly from other types of biomass burning) suggests that they can undergo further oxidative reactions with OH and O3 (Grieshop et al. Citation2009; Cubison et al. Citation2011; Hennigan et al. Citation2011; Heringa et al. Citation2011), which needs further investigations. In the category of “others” (mainly N-containing compounds), about 3% of the organic signals were due to CxHyN+ z, probably from N-heterocyclic alkaloid compounds as in other BBOA (Laskin et al. Citation2009).
3.4. Elemental Analysis
EA with HR-ToF-AMS data has been shown to be useful in obtaining the average elemental composition in organic aerosols (Aiken et al. Citation2007, Citation2008). When frag_18/28_v2 as in Aiken et al. (Citation2008) was used, the estimated O/C, H/C, and OM/OC ratios of particles from the three types of incense burning were 0.29–0.33, 1.51–1.58, and 1.54–1.60, respectively (), with 5%–10% variations for the same substrate. These narrow ranges suggest that in terms of average elemental composition, organic particles from these three types of incense burning are similar, although certain features are different as shown earlier. Also shown in are some values of atomic ratios of BBOA from the literature (Aiken et al. Citation2008; DeCarlo et al. Citation2008; Mohr et al. Citation2009; He et al. Citation2010), which were also obtained based on frag_18/28_v2.
TABLE 2 Oxygen to carbon elemental ratio (O/C), hydrogen to carbon elemental ratio (H/C), and organic matter to organic carbon (OM/OC) ratio of organic compounds in particles from the burning of IS, IC, and MC
When the modified fragmentation table (frag_18/28_v_i) is used, however, the estimated O/C ratios of all three substrates (IS, IC, and MC) are significantly higher (). This indicates that particles from our incense burning experiments are more oxygenated than those estimated using frag_18/28_v2, which underestimates the contribution of H2O+ and CO+ ions to the organic signals in particles from incense burning. As Weimer et al. (Citation2008) showed that smoldering generated particles with more oxygenated ions, it is also likely that incense burning, in which only smoldering occurs, generates more oxygenated particles than other BBOA from flaming process. Extensive dilution that approaches an ambient partitioning process (Section 2.1) may also be a reason for more oxygenated (hence nonvolatile) organics to remain in the particle phase, resulting in higher O/C ratios (oxidation state) of organic particles from our incense burning experiments. The actual O/C ratio of organic aerosols from biomass burning in general is an important issue regarding the oxidation state of organic aerosols derived from biomass burning under different circumstances, which warrants further investigations. In this study, we conclude that the O/C ratios from our incense burning experiments are between 0.3 using frag_18/28_v2 and 0.5 using the modified frag_18_28_v_i, which assumes that all H2O+ signals are from organic compounds (see Section 2 in the online supplemental information).
4. CONCLUSIONS
Particulate organics emitted from the burning of three types of commonly used incense (ISs, ICs, and MCs) were characterized using an Aerodyne HR-ToF-AMS. Both UMR and HR mass spectral analyses indicated high similarity between particles from incense burning and those from biomass burning. Besides high intensities in ion peaks at lower m/z values (m/z 29, 43, etc.) and the well-known marker fragments at m/z 60 and 73 from sugar anhydrides (e.g., levoglucosan), UMR mass spectra also showed that a substantial fraction of signals was due to ions of high m/z values (100–200), especially from m/z 121, 137, 167, etc. Evidence suggests that these ions at high m/z values are structurally related to the lignin components in the substrates of the incense, which are woody or herbal substances. First, strong correlations of mass spectra from incense burning, as well as most of other BBOA, with the mass spectrum of lignin powder were observed. Second, HR mass spectra showed that the formulas of these ions are related to lignin monomers, as found in previous studies using a number of other mass spectrometric techniques. HR mass spectral results from this study also showed that the organics in particles from incense burning are generally oxygenated and unsaturated (and/or cyclic) in nature. These results are consistent with the moderately high O/C ratio (0.3–0.5) and low H/C ratio (1.5–1.7) of particles from incense burning compared with other POA, such as those from vehicular exhaust which mainly consist of saturated and reduced hydrocarbons (Schneider et al. Citation2006).
The oxygenated nature of the organic particles from incense burning may be due to the presence of water-soluble compounds such as sugar anhydrides and di-carboxylic acids (Tsai et al. Citation2010). On the other hand, the unsaturated nature might be due to the lignin-related contents. Although the relative abundance of the characteristic peaks (m/z 137, 167, etc.) for lignin-related compounds is small (∼1% in UMR organic mass spectra), the large fraction of total unsaturated and/or cyclic ions (∼30% in HR organic mass spectra) suggests that a significant fraction of these unsaturated, and probably aromatic, compounds is present in particles from incense burning. In light of strong correlation of mass spectra from biomass/incense burning with that of lignin powder, the fragments (m/z 107, 121, 137, 157, 167, and 181) attributable to the lignin monomers identified may also be useful as potential marker ions for biomass burning, including incense burning, in AMS analysis. Overall, results from this study highlight the usefulness of mass spectral information in the high m/z region (>100) from HR-ToF-AMS measurements concerning primary emissions such as incense burning for the chemical characteristics of organic particles from those primary sources.
uast_a_653017_sup_23558208.zip
Download Zip (2.2 MB)Acknowledgments
This work was supported by the Research Grants Council of Hong Kong (project no. 610909) and the University Grants Committee of Hong Kong (Special Equipment Grant, SEG-HKUST07).
[Supplementary materials are available for this article. Go to the publisher's online edition of Aerosol Science and Technology to view the free supplementary files.]
REFERENCES
- Aiken , A. C. , DeCarlo , P. F. and Jimenez , J. L. 2007 . Elemental Analysis of Organic Species with Electron Ionization High-Resolution Mass Spectrometry . Anal. Chem. , 79 : 8350 – 8358 .
- Aiken , A. C. , Decarlo , P. F. , Kroll , J. H. , Worsnop , D. R. , Huffman , J. A. Docherty , K. S. 2008 . O/C and OM/OC Ratios of Primary, Secondary, and Ambient Organic Aerosols with High-Resolution Time-of-Flight Aerosol Mass Spectrometry . Environ. Sci. Technol. , 42 : 4478 – 4485 .
- Alfarra , M. R. , Prevot , A. S. H. , Szidat , S. , Sandradewi , J. , Weimer , S. Lanz , V. A. 2007 . Identification of the Mass Spectral Signature of Organic Aerosols from Wood Burning Emissions . Environ. Sci. Technol. , 41 : 5770 – 5777 .
- Allan , J. D. , Delia , A. E. , Coe , H. , Bower , K. N. , Alfarra , M. R. Jimenez , J. L. 2004 . A Generalised Method for the Extraction of Chemically Resolved Mass Spectra from Aerodyne Aerosol Mass Spectrometer Data . J. Aerosol Sci. , 35 : 909 – 922 .
- Boerjan , W. , Ralph , J. and Baucher , M. 2003 . Lignin Biosynthesis . Annu. Rev. Plant Biol. , 54 : 519 – 546 .
- Chang , Y.-C. , Lee , H.-W. and Tseng , H.-H. 2007 . The Formation of Incense Smoke . J. Aerosol Sci. , 38 : 39 – 51 .
- Chen , Q. , Liu , Y. J. , Donahue , N. M. , Shilling , J. E. and Martin , S. T. 2011 . Particle-Phase Chemistry of Secondary Organic Material: Modeled Compared to Measured O:C and H:C Elemental Ratios Provide Constraints . Environ. Sci. Technol. , 45 : 4763 – 4770 .
- Cheng , Y. S. , Bechtold , W. E. , Yu , C. C. and Hung , I. F. 1995 . Incense Smoke-Characterization and Dynamics in Indoor Environments . Aerosol Sci. Tech. , 23 : 271 – 281 .
- Chiang , K. C. , Chio , C. P. , Chiang , Y. H. and Liao , C. M. 2009 . Assessing Hazardous Risks of Human Exposure to Temple Airborne Polycyclic Aromatic Hydrocarbons . J. Hazard. Mater. , 166 : 676 – 685 .
- Cubison , M. J. , Ortega , A. M. , Hayes , P. L. , Farmer , D. K. , Day , D. Lechner , M. J. 2011 . Effects of Aging on Organic Aerosol from Open Biomass Burning Smoke in Aircraft and Lab Studies . Atmos. Chem. Phys. Discuss. , 11 : 12103 – 12140 .
- DeCarlo , P. F. , Dunlea , E. J. , Kimmel , J. R. , Aiken , A. C. , Sueper , D. Crounse , J. 2008 . Fast Airborne Aerosol Size and Chemistry Measurements Above Mexico City and Central Mexico During the MILAGRO Campaign . Atmos. Chem. Phys. , 8 : 4027 – 4048 .
- DeCarlo , P. F. , Kimmel , J. R. , Trimborn , A. , Northway , M. J. , Jayne , J. T. Aiken , A. C. 2006 . Field-Deployable, High-Resolution, Time-of-Flight Aerosol Mass Spectrometer . Anal. Chem. , 78 : 8281 – 8289 .
- Engling , G. , Herckes , P. , Kreidenweis , S. M. , Malm , W. C. and Collett , J. L. Jr . 2006 . Composition of the Fine Organic Aerosol in Yosemite National Park during the 2002 Yosemite Aerosol Characterization Study . Atmos. Environ. , 40 : 2959 – 2972 .
- Grieshop , A. P. , Donahue , N. M. and Robinson , A. L. 2009 . Laboratory Investigation of Photochemical Oxidation of Organic Aerosol from Wood Fires 2: Analysis of Aerosol Mass Spectrometer Data . Atmos. Chem. Phys. , 9 : 2227 – 2240 .
- Gross , J. H. 2004 . Mass Spectrometry: A Textbook , Heidelberg , , Germany : Springer .
- He , L. Y. , Lin , Y. , Huang , X. F. , Guo , S. , Xue , L. Su , Q. 2010 . Characterization of High-Resolution Aerosol Mass Spectra of Primary Organic Aerosol Emissions from Chinese Cooking and Biomass Burning . Atmos. Chem. Phys. , 10 : 11535 – 11543 .
- Hennigan , C. J. , Miracolo , M. A. , Engelhart , G. J. , May , A. A. , Presto , A. A. Lee , T. 2011 . Chemical and Physical Transformations of Organic Aerosol from the Photo-Oxidation of Open Biomass Burning Emissions in an Environmental Chamber . Atmos. Chem. Phys. , 11 : 7669 – 7686 .
- Hennigan , C. J. , Sullivan , A. P. , Collett , J. L. Jr. and Robinson , A. L. 2010 . Levoglucosan Stability in Biomass Burning Particles Exposed to Hydroxyl Radicals . Geophys. Res. Lett. , 37 : L09806
- Heringa , M. F. , DeCarlo , P. F. , Chirico , R. , Tritscher , T. , Dommen , J. Weingartner , E. 2011 . Investigations of Primary and Secondary Particulate Matter of Different Wood Combustion Appliances with a High-Resolution Time-of-Flight Aerosol Mass Spectrometer . Atmos. Chem. Phys. , 11 : 5945 – 5957 .
- Hoffmann , D. , Tilgner , A. , Iinuma , Y. and Herrmann , H. 2009 . Atmospheric Stability of Levoglucosan: A Detailed Laboratory and Modeling Study . Environ. Sci. Technol. , 44 : 694 – 699 .
- Hu , M. T. , Chen , S. J. , Huang , K. L. , Lin , Y. C. , Lee , W. J. Chang-Chien , G. P. 2009 . Characteritization of, and Health Risks from, Polychlorinated Dibenzo-p-Dioxins/Dibenzofurans from Incense Burned in a Temple . Sci. Total Environ. , 407 : 4870 – 4875 .
- Izumi , A. and Kuroda , K. 1997 . Pyrolysis Mass Spectrometry Analysis of Dehydrogenation Lignin Polymers with Various Syringyl/Guaiacyl Ratios . Rapid Commun. Mass Sp. , 11 : 1709 – 1715 .
- Ji , X. , Le Bihan , O. , Ramalho , O. , Mandin , C. , D’Anna , B. Martinon , L. 2010 . Characterization of Particles Emitted by Incense Burning in an Experimental House . Indoor Air , 20 : 147 – 158 .
- Johnson , T. , Myers , J. , Kelly , T. , Wisbith , A. and Ollison , W. 2004 . A Pilot Study Using Scripted Ventilation Conditions to Identify Key Factors Affecting Indoor Pollutant Concentration and Air Exchange Rate in a Residence . J. Expo. Anal. Env. Epid. , 14 : 1 – 22 .
- Kessler , S. H. , Smith , J. D. , Che , D. L. , Worsnop , D. R. , Wilson , K. R. and Kroll , J. H. 2010 . Chemical Sinks of Organic Aerosol: Kinetics and Products of the Heterogeneous Oxidation of Erythritol and Levoglucosan . Environ. Sci. Technol. , 44 : 7005 – 7010 .
- Kjallstrand , J. , Ramnas , O. and Petersson , G. 1998 . Gas Chromatographic and Mass Spectrometric Analysis of 36 Lignin-Related Methoxyphenols from Uncontrolled Combustion of Wood . J. Chromatogr. A , 824 : 205 – 210 .
- Laskin , A. , Smith , J. S. and Laskin , J. 2009 . Molecular Characterization of Nitrogen-Containing Organic Compounds in Biomass Burning Aerosols Using High-Resolution Mass Spectrometry . Environ. Sci. Technol. , 43 : 3764 – 3771 .
- Lee , S. C. and Wang , B. 2006 . Characteristics of Emissions of Air Pollutants from Mosquito Coils and Candles Burning in a Large Environmental Chamber . Atmos. Environ. , 40 : 2128 – 2138 .
- Lin , P. , Engling , G. and Yu , J. Z. 2010 . Humic-Like Substances in Fresh Emissions of Rice Straw Burning and in Ambient Aerosols in the Pearl River Delta Region, China . Atmos. Chem. Phys. , 10 : 6487 – 6500 .
- Lin , T. C. , Chang , F. H. , Hsieh , J. H. , Chao , H. R. and Chao , M. R. 2002 . Characteristics of Polycyclic Aromatic Hydrocarbons and Total Suspended Particulate in Indoor and Outdoor Atmosphere of a Taiwanese Temple . J. Hazard. Mater. , 95 : 1 – 12 .
- Lin , T.-C. , Krishnaswamy , G. and Chi , D. 2008 . Incense Smoke: Clinical, Structural and Molecular Effects on Airway Disease . Clin. Mol. Allergy , 6 : 3
- Lipsky , E. M. and Robinson , A. L. 2006 . Effects of Dilution on Fine Particle Mass and Partitioning of Semivolatile Organics in Diesel Exhaust and Wood Smoke . Environ. Sci. Technol. , 40 : 155 – 162 .
- Liu , W. L. , Zhang , J. F. , Hashim , J. H. , Jalaludin , J. , Hashim , Z. and Goldstein , B. D. 2003 . Mosquito Coil Emissions and Health Implications . Environ. Health Perspect. , 111 : 1454 – 1460 .
- Lung , S. C. C. and Kao , M. C. 2003 . Worshippers’ Exposure to Particulate Matter in Two Temples in Taiwan . J. Air Wast Manage. , 53 : 130 – 135 .
- McLafferty , F. W. and Turecek , F. 1993 . Interpretation of Mass Spectra , Mill Valley , CA : University Science Books .
- Mohr , C. , Huffman , J. A. , Cubison , M. J. , Aiken , A. C. Docherty , K. S. 2009 . Characterization of Primary Organic Aerosol Emissions from Meat Cooking, Trash Burning, and Motor Vehicles with High-Resolution Aerosol Mass Spectrometry and Comparison with Ambient and Chamber Observations . Environ. Sci. Technol. , 43 : 2443 – 2449 .
- Ng , N. L. , Canagaratna , M. R. , Jimenez , J. L. , Zhang , Q. , Ulbrich , I. M. and Worsnop , D. R. 2011 . Real-Time Methods for Estimating Organic Component Mass Concentrations from Aerosol Mass Spectrometer Data . Environ. Sci. Technol. , 45 : 910 – 916 .
- Ng , N. L. , Canagaratna , M. R. , Zhang , Q. , Jimenez , J. L. , Tian , J. Ulbrich , I. M. 2010 . Organic Aerosol Components Observed in Northern Hemispheric Datasets from Aerosol Mass Spectrometry . Atmos. Chem. Phys. , 10 : 4625 – 4641 .
- Saito , K. , Kato , T. , Takamori , H. , Kishimoto , T. and Fukushima , K. 2005 . A New Analysis of the Depolymerized Fragments of Lignin Polymer Using ToF-SIMS . Biomacromolecules , 6 : 2688 – 2696 .
- Sang , X.-F. , Chan , C.-Y. , Engling , G. , Chan , L.-Y. , Wang , X.-M. Zhang , Y.-N. 2011 . Levoglucosan Enhancement in Ambient Aerosol during Springtime Transport Events of Biomass Burning Smoke to Southeast China . Tellus B , 63 : 129 – 139 .
- Schneider , J. , Weimer , S. , Drewnick , F. , Borrmann , S. , Helas , G. Gwaze , P. 2006 . Mass Spectrometric Analysis and Aerodynamic Properties of Various Types of Combustion-Related Aerosol Particles . Int. J. Mass Spectrom. , 258 : 37 – 49 .
- See , S. W. , Balasubramanian , R. and Joshi , U. M. 2007 . Physical Characteristics of Nanoparticles Emitted from Incense Smoke . Science and Technology of Advanced Materials , 8 : 25 – 32 .
- Simoneit , B. R. T. 2002 . Biomass Burning—A Review of Organic Tracers for Smoke from Incomplete Combustion . Appl. Geochem. , 17 : 129 – 162 .
- Simoneit , B. R. T. , Schauer , J. J. , Nolte , C. G. , Oros , D. R. , Elias , V. O. Fraser , M. P. 1999 . Levoglucosan, a Tracer for Cellulose in Biomass Burning and Atmospheric Particles . Atmos. Environ. , 33 : 173 – 182 .
- Tsai , Y. I. , Wu , P.-L. , Hsu , Y.-T. and Yang , C.-R. 2010 . Anhydrosugar and Sugar Alcohol Organic Markers Associated with Carboxylic Acids in Particulate Matter from Incense Burning . Atmos. Environ. , 44 : 3708 – 3718 .
- Wang , B. , Lee , S. C. and Ho , K. F. 2006 . Chemical Composition of Fine Particles from Incense Burning in a Large Environmental Chamber . Atmos. Environ. , 40 : 7858 – 7868 .
- Wang , B. , Lee , S. C. , Ho , K. F. and Kang , Y. M. 2007 . Characteristics of Emissions of Air Pollutants from Burning of Incense in Temples, Hong Kong . Sci. Total Environ. , 377 : 52 – 60 .
- Weber , S. 2006 . Exposure of Churchgoers to Airborne Particles . Environ. Sci. Technol. , 40 : 5251 – 5256 .
- Weimer , S. , Alfarra , M. R. , Schreiber , D. , Mohr , M. , Prevot , A. S. H. and Baltensperger , U. 2008 . Organic Aerosol Mass Spectral Signatures from Wood-Burning Emissions: Influence of Burning Conditions and Wood Type . J. Geophys. Res.-Atmos. , 113 : D10304
- Zhang , Q. , Alfarra , M. R. , Worsnop , D. R. , Allan , J. D. , Coe , H. Canagaratna , M. R. 2005 . Deconvolution and Quantification of Hydrocarbon-Like and Oxygenated Organic Aerosols Based on Aerosol Mass Spectrometry . Environ. Sci. Technol. , 39 : 4938 – 4952 .