Abstract
For rapid detection, airborne micro-organisms can be collected by impaction on silicone sealants immediately followed by fluorescence or non-fluorescence staining and microscopy. At present, it is not known which combination of silicone, dye, and additional treatment provides best staining results. In laboratory experiments, selected groups of micro-organisms were prepared, aerosolized in a test tubing system, and collected by impaction on microscope slides that were coated with a silicone sealant before sampling. After sampling, different staining procedures were performed and the stained particles were counted under a fluorescence microscope. Two different sealants, 16 different dyes and staining procedures, and 21 additional reagents (buffers, solvents, and acids/bases) that may improve staining results were tested. In order to estimate the possible loss rate of particles caused by staining, washing, and additional treatment, the particles adhered to the silicones were counted under the microscope both before and after the procedures. The average losses of adhered micro-organisms and latex particles depended on the type of the silicone, the time between impaction of the particles and the application of the dye, the particle size, and the kind of treatment. Particle losses were less than 1% in most cases when staining solutions were applied 5 min after sampling. Most of the tested dyes showed good or even excellent staining properties on both types of silicone sealants and allowed to distinguish collected airborne bacteria from background debris. Best performance was achieved by “Grimm Silicone 1228” and the dye SYBR® Safe using a portable fluorescence microscope. The method was also tested successfully for the rapid detection of airborne micro-organisms in animal housings.
Copyright 2012 American Association for Aerosol Research
INTRODUCTION
In recent years fluorescence microscopy has become a fast alternative to the classical cultivation methods for the detection and counting of airborne micro-organisms (Moschandreas et al. Citation1996; Chi and Li Citation2005, Citation2006). The bacteria are usually collected on adherent surfaces, gels, or filters, and stained with dyes so that the cells can be distinguished from background material or debris. The success of this method strongly depends on the specificity of the stains and the quality of the adherent surfaces used. The main disadvantages of many adherent surfaces used as sample media are poor retention efficiencies for the airborne particles, high potential for auto-fluorescence, and cross-reactions with the dyes, leading to weak discrimination power between micro-organisms and debris. All of this leads to low bacterial counting results (Moschandreas et al. Citation1996).
In previous experiments with yeast cells, we found that silicone sealants prepared as thin coatings on microscope slides seem to be appropriate surfaces for the collection of bio-aerosols for fluorescence microscopic analysis. Out of 30 different tested adhesive coatings, “single component room temperature vulcanizing” (RTV) silicones were the most efficient showing collection efficiencies up to 97%. Furthermore, cell losses and background fluorescence were negligible when the coated slides were used within 50 min after preparation and a standardized staining procedure with 4′,6-diamidino-2-phenylindole (DAPI) was applied (Clauß et al. Citation2010). However, these results were gained by testing 1 species of micro-organisms and 1 staining method and little is known how these sealants are reacting with other types of micro-organisms such as molds and bacteria. Therefore, it seemed useful to test the sealants and the staining procedure with bacteria and molds in addition to yeast cells and monodisperse latex particles. Furthermore, it is well established that different groups of micro-organisms differ markedly in the chemical composition and appearance of their cell walls, as well as in surface texture, which can considerably influence the success of staining and may also have an effect on the retention of the micro-organisms by the tested silicone coatings. In regard to staining, a multitude of fluorescent dyes and staining procedures are commonly used depending on the application, each with its own assets and drawbacks (Raymond et al. Citation1994). For example, the combination of DAPI and propidium iodide (PI) allows discrimination between living and dead cells (Kopke et al. Citation2000), whereas acridine orange (AO) allows discrimination between active and non-active cells and also segregation of bacterial cells from non-bacterial substances, background cells, and debris (Lauer et al. Citation1981; Kuwae and Hosokawa Citation1999). A disadvantage is the high mutagenicity of these dyes intercalating in DNA. New fluorescent nuclear stains like SYTO® or SYBR® dyes are less toxic and less mutagenic and therefore easier to handle, which is especially important for field experiments. Most of the dyes commonly used are soluble in water, but some need to be dissolved, for example, in acetone, ethanol, or dimethyl sulfoxide (DMSO). Others are pH sensitive and require acids, bases, or buffer (Haughland Citation2002). In most cases, before or after staining, additional washing steps with different buffers or tensides like sodium dodecyl sulphate (SDS), TweenTM 80, or Triton® X-100 are recommended (Mansour et al. Citation1984; Huber et al. Citation1985; Aodongeril et al. Citation2009). These reagents may improve staining results but can also negatively influence the retention efficiency of the silicone.
This paper compares several dyes and staining procedures for the rapid detection of airborne micro-organisms collected on single component RTV silicones. Particle retention efficiencies will be presented for 2 silicones and for different groups of micro-organisms, and recommendations are given for an optimal staining procedure of microbial cells. Special attention was paid to a rapid and efficient but simple staining procedure for field experiments.
MATERIALS AND METHODS
Selected groups of micro-organisms and latex particles were prepared and aerosolized in a laboratory-based aerosol test tubing system. Samples were taken by impaction from the air stream in the aerosol tube on microscope slides coated with 2 selected silicone sealants. After sampling, the surface of the sealants was stained and treated staining and additional treatments were conducted directly onto the silicone surface. The retention efficiencies of the silicones were calculated by counting the impacted cells/particles under a fluorescence microscope before and after staining or treatment with different reagents.
Cultivation of Defined Micro-Organisms and Preparation of Test Aerosols
Cladosporium herbarum ATCC 11281 and Penicillium expansum ATCC 36200 were chosen because their spores are commonly found in ambient air. The molds were cultivated 5 days at 30°C on dichloran-glycerol (DG18) agar (Oxoid Deutschland GmbH, Wesel, Germany). To harvest the spores, 3.0 g sterile quartz sand was dispersed on each Petri dish. The mixture of sand and spores was well shaken and was removed by washing with sterile distilled water with 0.1% Tween 80. To remove the spores from the sand, the suspension was treated for 2 min in an ultrasonic bath. The supernatant was collected and centrifuged at 1000 rcf (relative centrifugal force) for 10 min at 20°C. The resulting pellet was resuspended and washed 3 times in sterile distilled water.
Yeast cells of the genus Saccharomyces cerevisia ATCC 9763 were chosen as test-organisms because they are non-pathogenic, easy to handle, and also easy to label with the non-toxic fluorescence stain Mycoval. Cells were incubated for 72 h at 25°C on Sabouraud 2% glucose agar (Merck KGaA, Darmstadt, Germany), denaturated in 50% Ethanol (p.a.) for 30 min, centrifuged at 2000 g for 1 min and resuspended in a 5% Mycoval solution (Helmut Hund GmbH, Wetzlar, Germany). After 30 min, the stained cells were washed with distilled water 3 times. In a similar experiment, living and unstained cells were used to evaluate the influence of denaturation with ethanol and pre-staining with Mycoval on the retention efficiency.
Spores of the Gram positive bacterium Bacillus subtilis ATCC 6051 are also a common contaminant in ambient air. Bacteria were cultivated on nutrient agar plates (meat extract 3.0 g, tryptone 5.0 g, agar 15.0 g [Oxoid Deutschland GmbH, Wesel, Germany]) supplemented with 10.0 mg MnSO4 x H2O per liter to induce sporulation. After 72 h at 30°C when >99% of the bacilli formed spores, colonies were harvested by a cotton bud and resuspended in cold sterile distilled water. The solution was treated for 2 min in an ultrasonic bath and centrifuged with 3000 g for 15 min at 4°C. The pellet was resuspended and washed 3 times in distilled water to remove remaining vegetative bacteria and cell debris.
Among Gram positive bacteria, Micrococcus luteus (ATCC 4698) is often found in outdoor ambient air, whereas Staphylococcus aureus (ATCC 25923) is often found in highly microbial contaminated air, e.g., animal housing. The Gram negative bacterium Escherichia coli (ATCC 25922) is rarely found in air, but was chosen for our experiments because it is one of the best known and commonly used test micro-organism. Bacterial cells of the 3 strains were cultivated on blood agar base No. 2 (Oxoid Deutschland GmbH, Wesel, Germany) at 36°C for 48 h, and colonies were harvested with a cotton bud and resuspended in distilled water.
Additionally, the influence of the particle size only on the retention efficiency was evaluated by using monodisperse organic latex spheres with defined sizes of 1.0 μm and 3.0 μm (Polysciences Europe GmbH, Eppelheim, Germany).
Silicone Sealants and Adherent Surface Preparation
In prior experiments, the single component “RTV” silicones “Sista Küchensilikon” (Henkel KGaA, Düsseldorf, Germany) and “Special Coating Silicone for Bio-aerosol Sampling 1228” (Grimm Aerosol Technik GmbH & Co. KG, Ainring, Germany) turned out to be most suitable for the collection and retention of airborne organic particles. The first one has an acetic curing system and a pH of approximately 3, whereas the second one has a neutral curing system and a pH of approximately 7. For the experiments, approximately 3 μg of the silicones were applied directly into the 8 mm wells of Diagnostic Slides (Menzel GmbH, Braunschweig, Germany) forming a homogeneous layer of constant thickness with an even surface. Excess material was easily removed by a glass cover slip.
Experimental Setup for the Aerosol Measurements
Suspensions of micro-organisms or particles in distilled water were sprayed in the air stream of a laboratory-based aerosol test tubing system, which was described in detail by Clauß et al. (2010). In general, it is constructed of 125 mm Ø KGB PVC pipes and stainless steel boxes. A medical nebulizer was used for injection of the test aerosol. A fan in one of the stainless steel boxes provides the under-pressure in the tubing system and a constant air speed of 1.35 m/s throughout the experiments. All samples were taken at a room temperature of 22°C ± 2°C. Aerosolized particles were collected from the air-stream in the tube by an isokinetic sampling probe with a Portable Bio-aerosol Spectrometer 1.209 (Grimm Aerosol Technik GmbH & Co. KG, Ainring, Germany). The Bio-aerosol Spectrometer is equipped with a round jet impactor, which allows the collection of particles from an air-stream on a microscope glass slide forming round deposition spots.
The number and sizes of particles in the sample air volume were measured in real time and reasonable sampling times were estimated to avoid particle bounce due to overloading of the collection surface. After that, 3 experiments were performed. First, the deposited test micro-organisms were stained with DAPI to evaluate the retention efficiencies of the silicone sealants for the different bacteria, mold spores, and yeast. Second, the micro-organisms were treated with other different dyes to evaluate the performance to visualize and distinguish airborne micro-organisms from background debris. In addition, cells were treated with different reagents to test the ability to improve the staining results. Third, Mycoval®-labeled yeast cells were treated with the same different reagents to check the influence of these reagents to the retention efficiencies of the silicones.
Dyes and Staining Procedures
Sixteen different dyes and stains were tested on silicone sealants regarding an optimal staining performance (). The staining procedures were kept as simple as possible to allow the use in field experiments in combination with a portable fluorescence microscope.
TABLE 1 Manufactures (M), excitation and emission wavelengths, filter sets used (F), as well as preparation of stock solutions (SS) and working solutions (WS) for 16 different fluorescence and non-fluorescence dyes
Due to the high hydrophobicity of the silicone surfaces, only a small amount of 10 μL of the working solutions applied with a pipet directly onto the deposition spot was sufficient for each experiment. Within 1 min, micro-organisms were well stained with most of the tested fluorescent dyes. To obtain optimal results, a staining time of 5 min was maintained in each case. After that time, the remaining stains were washed away and rinsed with distilled water. For the classical methylene blue staining, the Gram staining (crystal violet, Gram's iodine, and Safranin), and the spore staining by Wirtz-Conklin staining method (Schaeffer and Fulton Citation1933; malachite green), common standard protocols were conducted as described, e.g., in Horobin and Kiernan (Citation2002). Besides the staining with only 1 dye, useful combinations of 2 dyes were also tested. Staining with HI and Syto®9 as well as the classical Gram staining were tested for the discrimination between Gram positive and Gram negative bacteria. The combination of DAPI and PI was used to discriminate between living and dead cells. To obtain dead cells, bacteria were treated with 70% EtOH for 30 min.
Additional Chemical Treatment
Several buffers or solvents are commonly used to dissolve or dilute the dyes; tensids are used to improve staining results (). The influence of the most often used reagents for this purpose on the retention efficiency of the silicone sealants was tested. Fluorescent labeled yeast cells were deposited on silicone coated microscope slides and treated with 1 mL of different reagents for 10 min. After this procedure, the microscope slides were rinsed with distilled water. This procedure war repeated 3 times per reagent.
TABLE 2 Reagents tested on the silicone sealants
Fluorescence Microscopy and Image Analysis
The deposition spots had typical diameters of 1 mm. For all experiments, each time a minimum of 300 cells was counted in 1 defined area per slide in the center of the deposition spot for statistical validation. For counting bacteria cells the area was 100 × 130 μm (1000x magnification), and 1.0 × 1.3 mm (100x magnification) for counting mold spores and yeast cells. Microscopic images of collected particles adhered to the silicone surfaces were taken with a CCD camera (ProgRes®CF, Jenoptik, Jena, Germany) attached to a “Primostar iLED” (Carl Zeiss Jena GmbH, Jena, Germany) or a “H-600 AFL” (Helmut Hund GmbH, Wetzlar, Germany) fluorescence microscope. The number of adhered cells was counted before and after staining or chemical treatment, and the ratios were calculated by forming the quotient of cell counts before and after stain/treat. To evaluate the performance of the staining methods and the suitability of the silicones for this purpose, 4 ranks were established: “excellent,” “good,” “fair,” and “poor” performance. Here, the staining intensity for micro-organisms, as well as auto-fluorescence of the silicones and cross-reactions with the dyes were taken into account. The experiment for the evaluation of the retention efficiencies of the silicones for the different micro-organisms with a DAPI staining procedure was repeated 5 times. The evaluation of the staining performance of other dyes and the additional reagents as well as the investigation of the influence of the different reagents to the retention efficiencies of the silicones was repeated 3 times per dye and reagent.
Statistical Analysis
Statistical tests were conducted to compare the retention performances of the 2 silicones (Wilcoxon signed rank test) and to see if particle size possibly has an influence on the observed retention (Mann-Whitney U-test, particles of >3 μm and ≤3 μm diameter). The Bonferroni-Holm method (Holm Citation1979) was applied to correct for multiple testing with a global alpha of 0.05.
Field Sampling
To evaluate the suitability of the different methods for sampling in the field under practical conditions, airborne particles were collected at different locations where high concentrations of airborne micro-organisms could be expected (laying hen houses; animal house with cows, pigs sheep, and goats; piggery) and analyzed for micro-organisms. Before each sampling, a new microscope slide (a total of 5 slides per sampling site) was prepared with fresh silicone. The coated slides were immediately attached to the impactor of the Bio-aerosol-Spectrometer and the sampling was started. Due to the high particle concentrations in animal houses, the sampling time was limited to 10–30 s in order to prevent overloading of the slide. The slides were removed from the spectrometer after sampling and stained immediately each with 10 μL of the respective working solutions, which were applied with a pipet directly onto the deposition spots. After staining for 5 min, the remaining stains were washed off with distilled water. The waste water was collected and disposed according to applicable safety regulations. After drying, the slides were investigated onsite with the Primostar iLED fluorescence microscope.
RESULTS
Most of the dyes and staining procedures showed good or excellent staining results on both silicone sealants. It was possible to distinguish collected airborne bacteria from background debris. The average losses of adhered micro-organisms due to the staining procedures and additional treatments depended on the properties of silicone sealants, the curing time, the particle size, and the kind of treatment. However, particle losses were in all experiments nearly always less than 5%.
presents the performance of different staining procedures for airborne bacteria adhering to the silicone sealants “Sista Küchensilikon” and “Grimm Silicone 1228.” In regard to the efficiency, there were great differences between the staining methods but only slight differences between the silicones. Most of the procedures conducted showed good or even excellent staining results. The classical non-fluorescence staining methods like Gram staining or spore staining according to Wirtz (Schaeffer and Fulton Citation1933) were also feasible directly on the silicone surfaces and suitable for distinguishing collected airborne bacteria from the background. However, staining with fluorescence dyes obtained better results.
TABLE 3 Performances (++ excellent, + good, ○ fair, − poor) of different staining procedures for airborne bacteria adhered on “Sista Küchensilikon” and “Grimm Silicone 1228” silicone sealant
Outstanding results were obtained with the stains SYBR® Green and also with SYBR® Safe when “Grimm Silicone 1228” was used as an adhesive surface. Images of aerosolized bacteria collected in our laboratory test tube system and airborne particles collected in different animal houses, which were stained after sampling with SYBR® Green or SYBR® Safe, are presented in . The images show that both single airborne bacteria and bacteria within larger airborne particles could be well distinguished from background debris. In addition, auto-fluorescence of the silicone or cross-reactions with the dyes could not be observed.
The combination of staining with HI and Syto®9 as well as the classical Gram staining for the discrimination between Gram positive and Gram negative bacteria worked well in the laboratory experiments with selected strains. With both methods, Gram positive bacteria could be distinguished from Gram negative bacteria. For bacterial cells collected in field experiments, the staining results were often not clear. The same was found for the discrimination between living and dead (with DAPI and PI) and active and non-active cells (with AO) respectively.
compares the average losses of collected test aerosol particles, which adhered on the silicone sealants due to the described staining procedure for DAPI. The curing time showed to have an influence on the retention efficiency of the silicone: Particle losses ranged below 5% in most cases when the stain was applied immediately after sampling, and were even below 1% when the stain was applied after 5 min. In addition, also the particle size had a significant influence on retention efficiency (<3 μm vs. >3 μm, Mann-Whitney U-test, 2-sided, p = 0.002 (0 min), p = 0.003 (5 min), and p = 0.001 (10 min)). Higher losses were found for latex particles with 1 μm diameter than for latex particles with 3 μm diameter and also for the smaller bacteria and bacterial spores than for mold spores and yeast cells. However, the standard deviations were relatively high.
FIG. 1 Visualization of airborne bacteria collected and stained on RTV silicone (“Grimm Silicone 1228”). A: Cells of Bacillus subtilis and Staphylococcus xylosus aerosolized in a laboratory test system and stained with SYBR® Green or B: SYBR® Safe. C, E: Airborne particles stained with SYBR® Green collected in an alternative keeping system for laying hens. D: Aggregate of coccid bacteria collected in a stable for mixed farm animals stained with SYBR® Safe. F: Airborne particle stained with SYBR® Safe collected in a pig confinement system. (Color figure available online.)
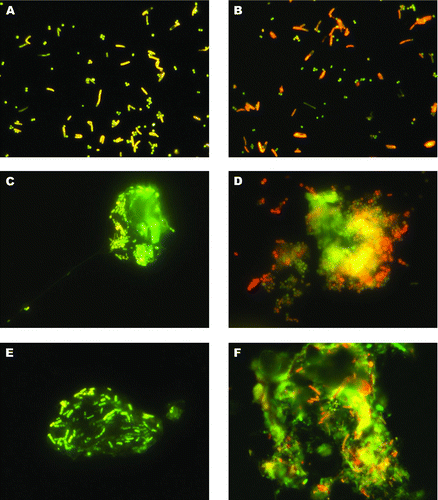
FIG. 2 Average losses of different particles adhered on 2 silicone sealants (“Sista Küchensilikon” and “Grimm Silicone 1228”) due to staining with DAPI. Staining was conducted 1 min (▪), 5 min (
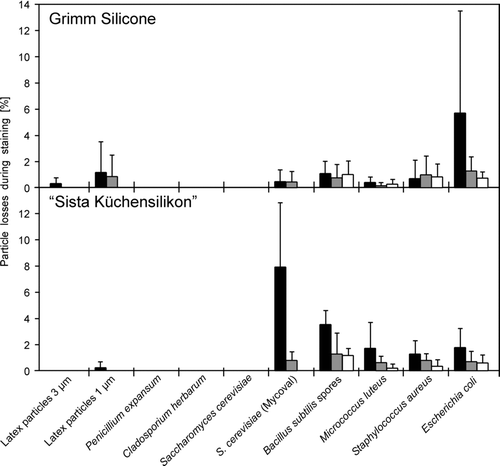
FIG. 3 Average losses of fluorescent labeled (Mycoval) yeast cells deposited on silicone sealants (“Sista Küchensilikon” (▪) and “Grimm Silicone 1228” (□)) and treated with 1 mL of different reagents for 10 min (n = 3, error bars represent standard deviations). The reagents were applied immediately onto the silicone surface after sampling.
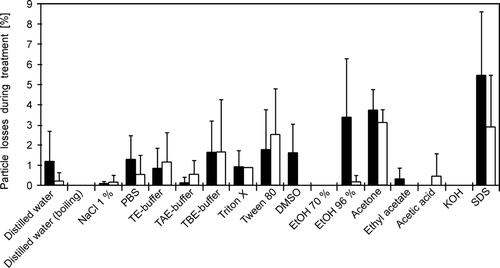
The influence of an additional treatment with different reagents on the retention efficiency of silicone surfaces for fluorescence labeled yeast cells was investigated. Labeled yeast cells were chosen because they showed the highest particle losses due to staining with DAPI in prior experiments. presents the average losses of yeast cells that were treated with 1 mL of different reagents for 10 min. The reagents were applied immediately after sampling onto the silicone surface. For both silicones, average particle losses were again less than 5% with only 1 exception (possibly a technical application failure). The standard deviations were also relatively high. Regarding retention efficiency, there were significant differences between the 2 silicones (Wilcoxon signed-rank test: z = 8.74, p = 0.001).
The effect of several additional treatments with different reagents on the staining results was also investigated. Clear positive effects could not be observed but some negative effects occurred. For example, treatment with TE buffer, TAE buffer, TBE buffer, and boiling water led to a destaining of Mycoval labeled cells. Washing with acids and bases had sometimes negative effects on the fluorescence brightness of the pH sensitive dyes. Treatment with ethyl acetate led to shrinking of the silicone surfaces to approximately 80% of their original size. Shrinking of the silicone surface could also be observed when coated microscope slides were stored in the refrigerator at 4°C for a few hours. Therefore, a simple washing step with distilled water and storage at room temperature is recommended after staining.
DISCUSSION
Key technology of the presented method for the collection and rapid detection of airborne micro-organisms was the combination of an adherent 1 component RTV silicone surface with high retention efficiency and the staining of the collected particles with high performance fluorescence dyes. Therefore, the choice of the right products is of great importance for an efficient and reliable staining.
A multitude of silicones with different chemical properties and curing systems is commercially available. Most suitable for our purpose was 1 component “RTV” silicones, which cure by reaction with atmospheric moisture. In this group, the “Sista Küchensilikon” had the highest retention efficiencies in previous experiments (Clauß et al. Citation2010). It has an acetic curing system and releases small amounts of acetic acid during the curing process (25 μg/mg; personal communication, Analyze Inc., Chandler, AZ, USA, May 2010). This led to a low pH of 3 of the surface, which may be disadvantageous for the purpose to sample living micro-organisms and the use of pH sensitive fluorescence dyes. Silicone sealants with neutral curing systems and a pH of 7 are certainly more suitable. Here, the “Grimm Silicone 1228” in these experiments showed similar retention efficiencies and even lower auto-fluorescence or cross-reactions with the tested dyes than “Sista Küchensilikon.” However, it has to be taken into account that the “Grimm Silicone 1228” releases small amounts of methanol during the curing process, which may also have an influence on the viability of certain micro-organisms.
A multitude of dyes for transmitted-light microscopy and fluorescent dyes for fluorescence microscopy are available. For the purpose to visualize and distinguish bacterial cells from background debris and for the rapid detection of airborne micro-organisms, fluorescence microscopy methods provided best results. With the described simple fluorescence staining method, an expert observer may distinguish between bacterial cells, yeast cells, and mold spores only on the basis of morphological characteristics as mentioned also by Fisar et al. (Citation1990). Most of the fluorescence dyes showed good or even excellent staining results. Only staining with nile red showed poor results and for FITC strong cross-reactions with the silicone surface and intense background staining could be observed. A slight green background was also visible when particles were stained with AO. Here the background staining disappeared within 10 s under the fluorescence microscope probably due to photo fading whereas bacterial cells remained stained for several minutes. The discrimination between active and non-active cells worked well with defined strains and bacterial cells inactivated with EtOH in the laboratory. In field experiments, bacteria were often found as somewhere between green and red and the results were often not clear. Staining with the classical fluorescence dyes DAPI or PI led to excellent staining results, but the same non-distinctive results regarding the discrimination between living and dead cells were found here as for the staining with AO. An additional disadvantage is that they are intercalating agents for DNA and therefore strong animal mutagens. In this regard also, HI or AO is problematic. The combinations of HI and Syto®9 and the classical Gram staining for the discrimination between Gram positive and Gram negative bacteria worked also well in the laboratory experiments with selected strains. But again in field experiments the results were often not clear. Bacterial cells appeared somewhere between green and orange and even cluster of the same bacteria were stained in different colors. Regarding the discrimination between bacterial cells and background debris for the enumeration of total bacterial count, outstanding staining results were obtained with SYBR® Green which is additionally much less mutagenic than DAPI or PI (Singer et al. Citation1999). SYBR® Green is an ultrasensitive stain for staining double stranded DNA and oligonucleotides, which is used mainly for post electrophoresis staining and real time PCR (Wittwer et al. Citation1997) and has also been used for the identification of viral, bacterial, and fungal pathogens. Similar staining results were obtained with SYBR® Safe when “Grimm Silicone 1228” was used as adherent surface. The staining with SYBR® Green was indeed slightly more intense but SYBR® Safe has the great advantage that it is non-toxic and non-mutagen and it is classified as non-hazardous although it bonds to nucleic acids (Haughland Citation2002). This is especially useful in field experiments when handling and disposing toxic chemicals may be difficult. It is stable at −20°C and when stored at 4°C in distilled water the working solution can be used for several weeks. However, SYBR® Safe is pH sensitive, which explains the “fair” staining results on “Sista Küchensilikon.”
Additional treatment and washing of the stained micro-organisms with different reagents were conducted to improve the staining results. Clear visible positive effects could not be observed but some negative effects occurred, which were mainly destaining (dependent on the pH sensitivity of the stains) or shrinking of the silicone surface. In most cases, we found that a simple washing step with distilled water after the staining was sufficient to obtain excellent results. This is useful for outdoor experiments when waste disposal of hazardous chemicals is a problem. However, it can be conducted when additional treatment or washing is preferred for some reasons. At least for almost all experiments the influence on the retention efficiency of the adhered particles was very low.
The combination of “Grimm Silicone 1228” as adherent surface for the collection of airborne particles, staining with SYBR® Green or SYBR® Safe, and rinsing with distilled water provided the best performance for the detection of collected airborne micro-organisms from air samples. Single airborne bacteria as well as bacterial cells within particles could be distinguished from background debris as seen in . This discrimination is of great interest, as we found out that only few bacteria were existent as single cells in the air. More often, large aggregates were found as seen in image D. In the majority of cases, several micro-organisms of different sizes and shapes were embedded in extracellular material (C, E) or found within larger particles of various compositions (F). This was found to be true not only for particles collected in animal housings but also for particles collected from ambient air contaminated with bacteria. Nevertheless, in most cases it was possible to detect and count single cells even when they were located within larger particles. Nevertheless, covering of fluorescing micro-organisms by larger dust particles remains a problem. For numeration of collected micro-organisms, it has to be kept in mind also that during impaction larger particles may alter their shape to some degree. In this regard, shows an aggregate of coccoid bacteria that seems to be deformed or even broke-up probably due to impaction. Therefore, it cannot be excluded that particle fractions that contained bacteria were blown away during sampling, although particle velocity during impaction is approximately 40 m/s relatively low due to impactor design and flow-rate. The same may occur for larger aggregates during staining and washing. However, images of the same particles taken with transmitted-light microscopy before staining and washing and with fluorescence microscopy afterwards indicated that even bacterial cells that lay onto other bacteria without a direct contact to the silicone surface were not washed away.
CONCLUSIONS AND OUTLOOK
In the present investigation, a combination of an adhesive surface for the efficient collection of airborne particles and a high performance dye for rapid staining and counting of micro-organisms is presented. The combination of “Grimm Silicone 1228” silicone, SYBR® Green or SYBR® Safe fluorescent dyes, and a washing step with distilled water after staining provided the best results. Most of the other dyes tested also showed good or even excellent staining results on both silicone sealants. The losses of already adhered particles due to the staining procedures or additional washing steps and treatments with 21 different reagents were generally very low and almost anytime less than 5% in all experiments. The retention efficiencies of the silicone sealants depended mainly on the kind of the silicone, the time between impaction of the particles and application of the staining solutions, the particle size, and the kind of treatment. The presented method was also suitable to visualize the appearance of collected airborne particles under a fluorescence microscope within minutes. Single microbial cells or such embedded in larger particles could be distinguished from background debris and thereby rapidly detected and counted. The method described can also be used in field measurements of micro-organisms with a portable fluorescence microscope. For this purpose, the use of SYBR® Safe is recommended because of its good performance and for safety reasons because it is classified as non-hazardous.
The successful detection and counting of airborne bacterial cells with the described method is only the first step to an evenly fast and simple but more specific technology than the rather unspecific dyes. Future sampling of micro-organisms from the air on silicone sealants should be combined with techniques such as “direct fluorescent antibody” (DFA) techniques or “fluorescent in situ hybridization” (FISH). These methods can identify single groups or even species of micro-organisms in environmental samples (Bohlool and Schmidt Citation1980; Parkes and Taylor Citation1985; Chi and Li Citation2005; Renton et al. Citation2008). However, DFA and FISH still suffer from cross-reactions or interferences with background auto-fluorescence that considerably reduce accuracy and reliability (Xi et al. Citation2003; She et al. Citation2007). Future studies will show if these problems can be solved or reduced by employing combination of above described silicone sealants and staining procedures.
Acknowledgments
This research project was funded by the Federal Ministry of Economics and Technology based on a decision of the German Federal Parliament.
REFERENCES
- Aodongeril , Yoshihara , K , Wang , X. , Wang , H. , Momotani , Y. Mori , Y. 2009 . Fluorescent Viability Assay of Mycobacterium Avium Subsp. Paratuberculosis by Carboxyfluorescein Diacetate Succinimidyl Ester and Carboxyfluorescein Diacetate . Int. J. Appl. Res. Vet. Med. , 7 ( 4 ) : 214 – 220 .
- Bohlool , B. B. and Schmidt , E. L. 1980 . “ The Immunofluorescence Approach in Microbial Ecology ” . In Advances in Microbial Ecology , Edited by: Alexander , M. Vol. 4 , 203 – 241 . New York : Plenum .
- Chi , M.-C. and Li , C.-S. 2005 . Fluorochrome and Fluorescent In Situ Hybridization to Monitor Bioaerosols in Swine Buildings . Aerosol Sci. Technol. , 39 : 1101 – 1110 .
- Chi , M.-C. and Li , C.-S. 2006 . Analysis of Bioaerosols from Chicken Houses by Culture and Non-Culture Method . Aerosol Sci. Technol. , 40 : 1071 – 1079 .
- Clauß , M. , Springorum , A. C. and Hartung , J. 2010 . Effective Collection of Airborne Micro-Organisms by Direct Impaction on Silicone Sealants: Comparison of Different Adherent Surfaces . Aerosol Sci. Technol. , 44 ( 11 ) : 993 – 1004 .
- Fisar , Z. , Hysek , J. and Binek , B. 1990 . Quantification of Airborne Microorganisms and Investigation of their Interactions with Non-Living Particles . Int. J. Biometeorol. , 34 : 189 – 193 .
- Haughland , R. P. 2002 . Handbook of Fluorescent Probes and Research Products , 9th ed , Edited by: Gregory , Jay . Eugene, OR : Molecular Probes Inc .
- Holm , S. 1979 . A Simple Sequentially Rejective Multiple Test Procedure . Scand. J. Stat. , 6 : 65 – 70 .
- Horobin , R. W. and Kiernan , J. A. 2002 . Conn's Biological Stains: A Handbook of Dyes, Stains, and Fluorochromes for Use in Biology and Medicine . 189 : 274 10th Ed., Taylor & Francis, London, New York
- Huber , H. , Huber , G. and Stetter , K. O. 1985 . A Modified DAPI Fluorescence Staining Procedure Suitable for the Visualization of Lithotrophic Bacteria . Syst. Appl. Microbiol. , 6 : 105 – 106 .
- Kopke , C. , Cristovao , A. , Prata , A. M. , Silva Pereira , C. , Figueiredo Marques , J. J. and San Romao , M. V. 2000 . Microbiological Control of Wine: The Application of Epifluorescence Microscopy Method as a Rapid Technique . Food Microbiol. , 17 : 257 – 260 .
- Kuwae , T. and Hosokawa , Y. 1999 . Determination of Abundance and Biovolume of Bacteria in Sediments by Dual Staining with 4′,6-diamidino-2-phenylindole and Acridine Orange: Relationship to Dispersion Treatment and Sediment Characteristics . Appl. Environ. Microbiol. , 65 ( 8 ) : 3407 – 3412 .
- Lauer , B. A. , Reller , L. B. and Mirrett , S. 1981 . Comparison of Acridine Orange and Gram Stains for Detection of Microorganisms in Cerebrospinal Fluid and Other Clinical Specimens . J. Clin. Microbiol. , 14 ( 2 ) : 201 – 205 .
- Mansour , J. D. , Schram , J. L. and Schulte , T. H. 1984 . Fluorescent Staining of Intracellular and Extracellular Bacteria in Blood . J. Clin. Microbiol. , 19 ( 4 ) : 453 – 456 .
- Moschandreas , D. J. , Cha , D. K. and Quian , J. 1996 . Measurement of Indoor Bioaerosol Levels by a Direct Counting Method . J. Environ. Eng. , 122 ( 5 ) : 374 – 378 .
- Parkes , R. J. and Taylor , J. 1985 . Characterization of Microbial Populations in Polluted Marine Sediments . J. Appl. Bacteriol. Sym. Suppl. , 59 ( 14 ) : 155 – 173 .
- Raymond , L. , Kepner , L. and Prattk , J. R. 1994 . Use of Fluorochromes for Direct Enumeration of Total Bacteria in Environmental Samples: Past and Present . Microbiol. Rev. , 58 ( 4 ) : 603 – 615 .
- Renton , A. , Filatova , E. , Ison , C. , Meheus , A. , Dmitriev , G. Akovbian , V. 2008 . Performance of Direct Fluorescent Antibody Tests for Routine Diagnosis of Chlamydia trachomatis in Russian Sexually Transmitted Disease Clinics . Int. J. STD AIDS , 19 : 851 – 855 .
- Schaeffer , A. B. and Fulton , M. D. 1933 . A Simplified Method of Staining Endospores . Science , 77 : 194
- She , R. C. , Billetdeaux , E. , Phansalkar , A. P. and Petti , C. A. 2007 . Limited Applicability of Direct Fluorescent-Antibody Testing for Bordetella sp. and Legionella sp. Specimens for the Clinical Microbiology Laboratory . J. Clin. Microbiol. , 45 ( 7 ) : 2212 – 2214 .
- Singer , V. L. , Timothy , E. L. and Yue , S. 1999 . Comparison of SYBR® Green I Nucleic Acid Gel Stain Mutagenicity and Ethidium Bromide Mutagenicity in the Salmonella/Mammalian Microsome Reverse Mutation Assay (Ames test) . Mutat. Res. , 439 : 37 – 47 .
- Wittwer , C. T. , Herrmann , M. G. , Moss , A. A. and Rasmussen , R. P. 1997 . Continuous Fluorescence Monitoring of Rapid Cycle DNA Amplification . Biotechniques , 22 ( 1 ) : 130 – 134 .
- Xi , C. , Balberg , M. , Boppart , S. A. and Raskin , L. 2003 . Use of DNA and Peptide Nucleic Acid Molecular Beacons for Detection and Quantification of rRNA in Solution and in Whole Cells . Appl. Environ. Microbiol. , 69 ( 9 ) : 5673 – 5678 .