Abstract
Flattening coefficients for liquid oleic acid droplets were determined for droplets containing varyig concentrations of uranine when impacted on surfactant-treated glass slides. Flattening coefficients varied by surfactant and by uranine concentration. The results of this work suggest that interfacial forces between oleic acid droplets and surfactant-coated slides change with surfactant chemistry enough to have significant impacts on results from evaluation of size-selective aerosol sampler performance. The effects of uranine concentration and surfactant type should not be extrapolated from data generated using alternative surfactants when using microscopy to verify the size of monodisperse liquid particles utilized for sampler performance testing.
Copyright 2012 American Association for Aerosol Research
INTRODUCTION
Monodisperse aerosols are used extensively for characterizing the performance of size-selective aerosol sampling equipment, including Federal Reference Method (FRM) ambient PM10 samplers (40 CFR Part 53 Subpart D), stack samplers (Paik and Vincent Citation2002; Astrid et al. Citation2003), and virtual impactors (Haglund et al. Citation2002; Haglund and McFarland Citation2004). Vibrating orifice aerosol generators (VOAGs) are often used to generate monodisperse liquid particles in the 1.5–50 μm range (Berglund and Liu Citation1973). The size of these particles can be predicted within ±2% based on knowledge of the liquid flow rate and excitation frequency imposed on the liquid jet (Berglund and Liu Citation1973), or it can be measured by impacting droplets on a glass slide treated with an oil-phobic film and measuring the droplets using a calibrated microscope (Berglund and Liu Citation1973; Gieseke and Mitchell Citation1973; Olan-Figueroa et al. Citation1982). When testing the performance of an ambient PM10 sampler for use as an FRM sampler, microscopic measurement of droplets is required to verify the droplet size predicted based on VOAG operational parameters [40 CFR 53.42(b)].
When a spherical liquid droplet is impacted onto a slide, it acquires a new shape resulting from the establishment of mechanical equilibrium between surface tension, interfacial forces and gravity. The apparent diameter of the flattened spheroid (D a) will be a function of the original spherical droplet diameter (D s), the mass and surface tension of the droplet, and the interfacial forces between the droplet and the surface of the slide, where the latter variable depends on the chemistry of both the droplet and the surface. A flattening coefficient (F) is defined as the ratio of the apparent diameter of the sessile droplet to the original spherical diameter and, once known, may be used to determine the spherical droplet diameter by measurement of the diameter of a flattened droplet impacted on a slide (EquationEquation (1)).
Oil-phobic surfactants are used to treat glass slides before droplet impaction so that impacted oil droplets maintain a consistent and compact shape. Whitby and Vomela (Citation1967) measured dioctyl phthalate (DOP) particles impacted on glass slides immersed in 0.2% solution of L-1083 (3M Co., St. Paul, MN) fluorocarbon surfactant and found a flattening coefficient of 1.450 for drops in the 50–150 μm range while a coefficient of 1.513 was determined for droplets from 150 to 400 μm in size. Because the surfactant used by Whitby and Vomenla (1967) was no longer available at the time of their experiments, Liu et al. (Citation1982) determined flattening coefficients for DOP and oleic acid droplets impacted onto glass slides treated with an experimental surfactant (L-1428, 3M Co., St. Paul, MN). Liu et al. (Citation1982) found that F was independent of droplet size in the 2—50 μm range and reported values of 1.450 and 1.429 for DOP and oleic acid droplets, respectively. However, the surfactant used by Liu et al. (Citation1982) is no longer commercially available. Cheng et al. (Citation1986) developed a method of measuring droplet spread factors (the inverse of flattening factors) using scanning electron microscope images taken at different beam-to-substrate orientations and analyzing shadow lengths. The authors impacted DOP droplets onto slides coated with a gold-platinum alloy and then L-1429 surfactant (3M Co., St. Paul, MN, USA). The authors reported an average spread factor of 0.582 (equivalent to a flattening coefficient of 1.72), but it is unknown how the alloy application affected droplet spreading.
Olan-Figueroa et al. (Citation1982) determined flattening coefficients for oleic acid droplets tagged with a fluorescent tracer (sodium fluorescein or uranine). Olan-Figueroa et al. (Citation1982) immersed slides in a 2% solution of a fluorocarbon surfactant distributed as either NyeBar Type CT (W.F. NYE, Inc., New Bedford, MA, USA) or FC-721 (3M Co., St. Paul, MN, USA) and impacted droplets of DOP and oleic acid with sodium fluorescein concentrations ranging from 10 to 20 g/mL. Flattening coefficients were determined by comparing the size of frozen, spherical droplets impacted on the slide to the diameter of the same droplets after they melted. The authors also measured the thickness and base diameter of impacted liquid droplets microscopically, but to do so, they oriented the slide vertically such that gravitational forces acted on the droplet parallel to the slide surface rather than normal to the slide surface, likely altering droplet shape. Olan-Figueroa et al. (Citation1982) found that flattening coefficients were unaffected by particle size (2.7–29.1 μm) but decreased with increasing uranine concentration. Average flattening coefficients for DOP and oleic acid droplets were 1.35 and 1.34, respectively. John and Wall (Citation1983) found similar results for pure DOP droplets impacted on slides treated with FC-721 compared to aerosolized droplet diameters measured using an optical counter.
Since 1982, NyeBar Type CT has become unavailable, and other surfactants have been used. Oil-phobic surfactants are primarily used to prevent oil creep in mechanical components and, as a result, manufacturers of these coatings have little interest in maintaining a specific spread factor during production. Among the most common surfactants used in aerosol research are NyeBar Type Q, which was also recently taken off the market, and its replacement, NyeBar Type L. The flattening coefficient of oleic acid droplets on slides treated with these surfactants is unknown. However, the flattening coefficient can significantly affect the calculated spherical diameter of liquid aerosol particles used to evaluate the performance of size-selective aerosol sampling equipment. The objective of the present work is to quantify the flattening coefficient of fluorescently tagged oleic acid droplets on glass slides treated with NyeBar Type Q and NyeBar Type L.
METHODS
The method used by Olan-Figueroa et al. (Citation1982) to determine flattening coefficients is labor intensive, and it has been observed that droplets tend to disintegrate within a few hours after impaction. Therefore, the time between droplet melting and measurement may affect the calculated flattening coefficient. Furthermore, the effect of the phase change from solid to liquid droplets on the flattening behavior of the droplets is not known, and the effect of orienting the slide vertically on measurements of droplet height was not considered by the authors.
Recent advances in microscopy allow for precise measurement of impacted droplets using confocal microscopy. In this study, a confocal microscope was used to measure lateral (X–Y) and axial (Z) dimensions of impacted fluorescently tagged oleic acid droplets impacted on slides coated with either NyeBar Type Q or NyeBar Type L.
Slide Preparation
Glass slides (VWR VistaVisionTM part number 16004–368; VWR International; Radnor, PA, USA) were cleaned with a 50% isopropanol/water mixture and dried using a Kimwipe (Kimberly–Clark Professional, Roswell, GA, USA). They were then treated with NyeBar Type Q (0.2%; Nye Synthetic Lubricants; Fairhaven, MA, USA) or NyeBar Type L (2.0%; Nye Synthetic Lubricants, Fairhaven, MA, USA) by placing 5 drops of NyeBar on the cleaned side of a slide before covering with a second clean slide. The slides were simultaneously pressed together and drawn away from each other, evenly coating both slides. Treated slides were allowed to air dry before use.
Particle Generation and Impaction
Monodisperse aerosol of fluorescently tagged oleic acid was generated using a Vibrating Orifice Aerosol Generator (VOAG) (Model 3450; TSI Inc., Shoreview, MN, USA). Oleic acid solutions having uranine concentrations of zero, 5%, 10%, and 20% by mass were aerosolized using a 20 μm orifice, a solution flow rate of 0.225 ml/min (including ethanol diluent and oleic acid), and an excitation frequency of 56.5 kHz. Particles were transmitted through a 10 mCi Krypton-85 charge neutralization column before being impacted on glass slides.
Berglund and Liu (Citation1973) reported a method for determining the diameter of particles generated using vibrating jet atomizers and estimated the uncertainty of the calculated diameters to be less than 2%. The diameter of a spherical drop (D s) produced by a VOAG after the volatile fraction has evaporated is calculated as:
Solutions were mixed to yield a nominal 10 μm aerodynamic diameter droplet of nonvolatile liquid aerosol, which was impacted on slides using a custom slide impactor. (Note: Pure oleic acid droplets were mixed to yield nominal 10.6 μm aerodynamic diameter droplets.) The impactor drew 17 Lpm of particle-laden air through a 6.35 mm diameter orifice, which was 3.7 mm from the slide surface (). Particles were impacted on slides for approximately 0.5 s per slide. This procedure typically produced a circular deposition zone on the glass slide having a diameter of a few millimeters and containing many hundreds of individual droplets. The areal density (droplets per unit surface area) can be adjusted by changing the duration of exposure of the impactor to the particle-laden air.
Droplets for each treatment (4 levels of uranine concentration × 2 categories of Nyebar type) were impacted on 5 slides, for a total of 40 slides. The uranine concentration of the generated aerosol was completely randomized (with the exception of “zero uranine” slides, which were all generated in 1 block). Once the solution concentration was established, droplets were impacted on 1 slide treated with Type L and 1 slide treated with Type Q Nyebar before changing the uranine concentration for the next set of tests. Within each solution concentration block, the order in which the 2 slide treatments were impacted was randomized.
The temperature, relative humidity, and barometric pressure of the ambient environment were recorded during impaction of each slide along with the time of impaction.
Particle Measurement
Within 6 h of impaction, a portion of each slide was imaged using a Keyence VK-9700 Series Color 3D Laser Scanning upright confocal microscope (Keyence Corp., Itasca, IL, USA) with a 50X/0.95 objective. A 408 nm wavelength laser was used for illumination, and droplets were analyzed in reflectance mode. An example of analyzed droplets is shown in . The temperature, relative humidity, and barometric pressure of the ambient environment were recorded during imaging of each slide along with the time of imaging.
FIG. 2 Example of droplet images used for analysis (left) and droplet height profile (right). (Color figure available online.)
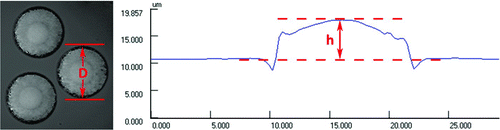
According to the Rayleigh Criterion, the lateral resolution (R L) of measurements made using a confocal microscope can be calculated by:
A pixel size smaller than the Nyquist rate of ½ R L was used to optimize lateral resolution. The axial resolution of a confocal microscope is a complex function related to the wavelength of light used for illumination, pinhole size, numerical aperture, and the refractive index of the medium used for imaging. Furthermore, the effective resolution may be less than the calculated resolution when low signal-to-noise ratios are observed. Based on communication with the manufacturer, the axial resolution of the confocal microscope used was estimated to be 10 nm for the present application.
The apparent area and height (h) of each of ten droplets was then measured using VK-Analyzer software (Keyence Corp., Itasca, IL, USA). The apparent diameter of each droplet (D a) was calculated from the measured apparent area, and the volume of the droplet (V) was calculated using EquationEquation (4), assuming the droplet takes a plano-convex lens shape upon impaction (Gieseke and Mitchell Citation1973):
From this volume calculation, the diameter of a spherical aerosol of equivalent volume was calculated. These spherical diameters were compared with expected droplet diameters (EquationEquation (2)) and were used to calculate flattening coefficients for each droplet (EquationEquation (1)); thus the flattening coefficients reported in this study were determined independently from the operational parameters of the VOAG.
Previous studies have found that flattening coefficients do not vary for DOP droplets in the 50–150 μm range (Whitby and Vomela Citation1967) or oleic acid droplets in the 3–30 μm range (Olan-Figueroa et al. Citation1982). The Bond number (B) is a unitless value that indicates the relative effects of droplet weight versus surface tension forces on the shape of a sessile droplet (EquationEquation (5)):
The shape of droplets with small bond numbers (i.e., ≪≪1) would be dominated by surface tension and would not be expected to vary with size. For oleic acid droplets (density (ρ) = 0.895 g/cm3; surface tension (γ) = 32.5 dyne/cm; CAS Number 112–80-1) up to 100 μm in diameter, the bond number <0.002, indicating that surface tension forces will greatly exceed the forces of gravity and, therefore, flattening coefficients should be relatively constant for droplets less than 100 μm in diameter.
The systematic uncertainty in calculated flattening coefficients was estimated by propagating errors associated with primary measurements through data reduction equations in accordance with the International Organization for Standardization (ISO) Guide to the Expression of Uncertainty in Measurement (ANSI/ASME Citation1998).
RESULTS
The expected geometric diameters of all droplets produced were calculated based on VOAG parameters and solution compositions (EquationEquation (2)). Calculated spherical diameters based on measurements of flattened droplets are shown in along with measured flattening coefficients.
TABLE 1 Measured diameters and flattening coefficients of droplets (mean ±95% confidence interval)Footnote a
No significant differences were detected between the measured droplet sizes and the expected droplet size except for slides treated with Nyebar Type Q, which had a higher than expected droplet size for “zero uranine” droplets and a lower than expected droplet size for 5% uranine solutions. For a given uranine content, no differences were detected in droplet size by surfactant except at a uranine concentration of 5%.
Measured flattening coefficients are shown in . Average flattening coefficients measured by Olan-Figueroa et al. (Citation1982) are also included in to provide context for current measurements.
FIG. 3 Flattening coefficients (with 95% confidence intervals) by surfactant and uranine concentration. (Color figure available online.)
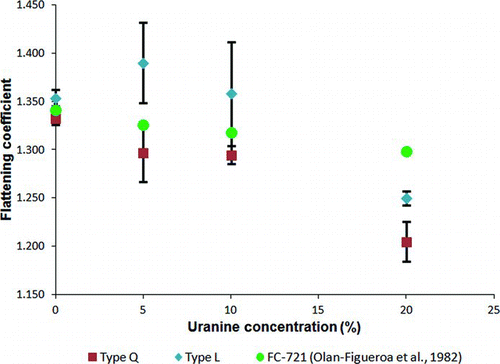
Although tests were conducted in a narrow range of environmental conditions, none of the environmental factors measured during particle impaction or measurement (i.e., temperature, relative humidity, water vapor pressure, and barometric pressure) significantly impacted observed flattening coefficients.
Similar to the results of Olan-Figueroa et al. (Citation1982), flattening coefficients for Nyebar Type Q varied in a linear fashion with uranine concentrations. Results for Nyebar Type L, however, were nonlinear when oleic acid droplets containing no uranine were considered. Flattening coefficients for both surfactants were significantly different than those reported by Olan-Figueroa et al. (Citation1982) for some droplets of similar composition, indicating a significant change in the interfacial forces occurring between the droplet and surfactant molecules as surfactant chemistry has changed.
The average flattening coefficients for droplets containing 10% uranine were 1.358 and 1.295 for Nyebar Type Q and Type L, respectively. The 4.6% difference in flattening coefficients between surfactant treatments would correspond to a 4.6% change is calculated spherical particle diameters when applying EquationEquation (1) to verify particle size during monodisperse particle generation. Such variation could have significant implications for sampler performance evaluation in regions of a sampler collection efficiency curve where sampler performance is highly dependent on particle size, such as near the cut point of a size-selective aerosol sampler. Furthermore, 40 CFR 53 Subpart D requires that candidate FRM PM10 samplers be challenged with liquid particles having that are within ±0.5 μm of the target particle size for droplet sizes ≤11 μm and within ±1.0 μm of the target particle size for droplet sizes ≥13 μm. For many of these particle sizes, a 4.6% error in calculated particle diameter would cause appropriately sized particles to be erroneously excluded from evaluation.
Based on the propagation of errors in primary measurements through data reduction equations (ANSI/ASME Citation1998), the average systematic uncertainty associated with measurements of flattening coefficients was 1.5%. Uncertainty in lateral measurements (i.e., apparent droplet diameter) accounted for >99% of the total uncertainty in calculated flattening coefficients.
SUMMARY AND CONCLUSIONS
Flattening coefficients for oleic acid droplets containing varying concentrations of uranine when droplets were impacted on glass slides treated with Nyebar Type Q and Type L surfactants were determined using confocal microscopy. Flattening coefficients varied by surfactant and by uranine concentration and were significantly different than values reported by Olan-Figueroa et al. (Citation1982) for Nyebar FC-721. The results of this work suggest that the interfacial forces between oleic acid droplets and surfactant-coated slides can vary significantly with changes in surfactant chemistry. These changes are great enough to have significant impacts on results from evaluation of size-selective aerosol sampler performance, particularly for particle sizes in regions of a sampler collection efficiency curve where sampler performance is highly dependent on particle size, such as near the cut point of a size-selective aerosol sampler. Therefore, when employing microscopy to verify aerosol particle sizes for precise work, the effect of uranine concentration and surfactant-dependent interfacial forces must be appropriately considered and should not be extrapolated from data generated using alternative surfactants.
Acknowledgments
The use of the Texas A&M University Polymer Technology Center Keyence VK-9700 confocal microscope is acknowledged. The use of the Microscopy and Imaging Center facility at Texas A&M University is acknowledged. The Olympus FV1000 confocal microscope acquisition (for preliminary studies not reported here) was supported by the Office of the Vice President for Research at Texas A&M University.
Notes
aNo statistical differences were detected between means in the same column followed by the same letter (α = 0.05).
bMeans followed by an * were not statistically different (α = 0.05) than the expected diameter calculated using EquationEquation (2).
REFERENCES
- American National Standards Institute/American Society of Mechanical Engineers (ANSI/ASME) . 1998 . Test Uncertainty, Performance Test Code 19.1—1998 , New York : ASME .
- Astrid , J. C. , Kuhlbusch , T. A. J. , Fissan , H. , Bröker , G. and Geueke , K. J. 2003 . Development of a PM10/PM2.5 Cascade Impactor and In-Stack Measurements . Aerosol Sci. Technol. , 37 : 694 – 702 .
- Berglund , R. N. and Liu , B. Y. H. 1973 . Generation of Monodisperse Aerosol Standards . Env. Sci. Technol. , 7 ( 2 ) : 147 – 153 .
- Cheng , Y. S. , Chen , B. T. and Yeh , H. C. 1986 . Size Measurement of Liquid Aerosols . J. Aerosol Sci. , 17 ( 5 ) : 803 – 809 .
- Gieseke , J. A. and Mitchell , R. I. 1973 . Size Measurements of Collected Droplets . J. Chem. Eng. Data , 10 : 350 – 353 .
- Haglund , J. S. , Chandra , S. and McFarland , A. R. 2002 . Evaluation of a High Volume Aerosol Concentrator . Aerosol Sci. Technol. , 36 : 690 – 696 .
- Haglund , J. S. and McFarland , A. R. 2004 . A Circumferential Slot Virtual Impactor . Aerosol Sci. Technol. , 38 : 664 – 674 .
- John , W. and Wall , S. M. 1983 . Aerosol Testing Techniques for Size-Selective Samplers . J. Aerosol Sci. , 14 ( 6 ) : 713 – 727 .
- Liu , B. Y. , Pui , D. Y. H. and Wang , X. Q. 1982 . Drop Size Measurement of Liquid Aerosols . Atmos. Environ. , 16 ( 13 ) : 563 – 567 .
- Olan-Figueroa , E. , McFarland , A. R. and Ortiz , C. A. 1982 . Flattening Coefficients for DOP and Oleic Acid Droplets Deposited on Treated Glass Slides . Am. Ind. Hyg. J. , 43 : 395 – 399 .
- Paik , S. and Vincent , J. H. 2002 . Aspiration Efficiency for Thin-Walled Nozzles Facing the Wind for Very High Velocity Ratios . Aerosol Sci. , 33 : 705 – 720 .
- Whitby , K. T. and Vomela , R. A. 1967 . Response of Single Particle Counters to Non-Ideal Particles . Env. Sci. Technol. , 1 : 801 – 814 .