Abstract
Variations of mixing state and chemical constituents of Asian dust (AD) particles having different transport pathways were investigated by measuring hygroscopicity and volatility of size-selected (1 μm) dust particles, and their morphology and elemental composition in Gwangju, Korea. Also, hygroscopicity and volatility of possible candidate chemical species that can be included in the dust particles was measured in a laboratory for comparison with field data. A significant amount of dust particles were found to be aged by internally mixing with hygroscopic and volatile species in different ways, depending on their transport pathway. Formation of hygroscopic CaCl2 and/or cloud processing of dust particles with sea-salt species were proposed as being central to the formation of hygroscopic species in the “less polluted AD” (in which the air mass arrived at sampling site without passing over significant industrial areas), and the existence of hygroscopic species in the “highly polluted AD” (in which the air mass passed over major industrial areas) was explained by the interaction of dust particles with anthropogenic pollutants (i.e., Ca(NO3)2 formation by heterogeneous reaction of HNO3 with dust particles and condensation of hygroscopic H2SO4 or (NH4)2SO4 onto the dust particles). Volatile carbonaceous species, which would exist on the surface of the dust particles, were also observed and their fraction significantly increased in the highly polluted AD due to a higher possibility to encounter air masses containing a significant amount of carbonaceous species. Morphological and elemental data identified three types of aged dust particles (reacted dust, cloud-processed dust, and aggregated dust). The production of various aged dust particles depending on their transport pathways has important implications on their different effects on cloud formation, radiation balance, and human health from original dust particles.
Copyright 2012 American Association for Aerosol Research
INTRODUCTION
Dust particles are an important fraction of the global aerosol loading, and influence radiation balance caused by scattering or absorbing incoming solar energy and cloud formation by serving as cloud condensation nuclei (CCN) (Levin et al. Citation1996; Buseck and Pósfai Citation1999; Ramanathan et al. Citation2001; Satheesh and Krishna Moorthy Citation2005; Kelly et al. Citation2007; Lack et al. Citation2009). They also have been known to exacerbate various health problems (Chen and Yang Citation2005; Ichinose et al. Citation2008; Yang et al. Citation2009; Hong et al. Citation2010; Hwang et al. Citation2010; Choi et al. Citation2011). Asian dust (AD) particles, typically originating from the Gobi desert, Loess plateau, Manju region, and Taklamakan desert located in Mongolia and China, are transported into Korea and Japan via a high-speed northwest wind (>∼3 m/s) (Sun et al. Citation2005). During long-range transport, dust particles can be exposed to various aging processes such as the heterogeneous reaction of dust particles with gases (Sullivan et al. Citation2007a; Tobo et al. Citation2010), condensation of gases having low vapor pressure on to the dust particles (Usher et al. Citation2003; Clarke et al. Citation2004), cloud processing of the dust particle with sea-salt species (Andreae et al. Citation1986; Trochkine et al. Citation2003; Murphy et al. Citation2006), coagulation of the dust particles with other types of particles (Chou et al. Citation2003), and interaction with varying amounts of water vapor. Thus, physicochemical properties of transported AD particles can be significantly different from the original dust particles, which mainly consist of mineral soil components (Trochkine et al. Citation2003; Cwiertny et al. Citation2008; Tobo et al. Citation2010). They may age in the atmosphere in different ways depending on the air masses encountered, which again have different chemical constituents.
In terms of dust particle conversions, Krueger et al. (Citation2004) showed that CaCO3 and CaMg(CO3)2 species in dust particles can react with HNO3 to form nitrate salts. More recently, Tobo et al. (Citation2010) identified the conversion of insoluble CaCO3 in dust particles to soluble Ca(NO3)2 over urban and industrial areas, and the formation of CaCl2 under high HCl concentration environment in marine boundary layer. Previously, Trochkine et al. (Citation2003) reported that 40–45% of mineral particles were internally mixed with sulfates during their long-range transport form China to Japan. Andreae et al. (Citation1986) suggested that a mixture of sea-salt, sulfate, and mineral dust (i.e., silicates) particles could be produced by cloud processing. Zhou et al. (Citation1996) also reported that the morphology and composition of dust particles were modified by their interaction with sea salt and anthropogenic pollutants during transport from China to Japan. Zhang et al. (Citation2010) showed that chemical composition of particles was different depending on transport pathway of dust storm.
Previous studies have provided useful insight into aging of dust particles by looking at the morphology and elemental composition of a single particle via microscopy techniques such as transmission electron microscopy (TEM), scanning electron microscope (SEM), and energy dispersive spectroscopy (EDS) (Zhou et al. Citation1996; Zhang and Iwasaka Citation1999; Ro et al. Citation2005; Shi et al. Citation2005; Li and Shao Citation2009), by measuring bulk chemical composition of particles in PM10 using a filter-based technique (Zhang et al. Citation2010), and by determining chemical composition of a single particle with single particle mass spectrometer technique in real time (Sullivan et al. Citation2007a, Citation2007b; Sullivan and Prather Citation2007; Sullivan et al. Citation2009). In the microscopy techniques, statistically, a limited number of particles were typically examined, and detection of nitrogen element that can be used as direct evidence for the formation of nitrate salts in dust particles was limited. In filter-based techniques, after sampling particles for 12–24 h, their bulk chemical composition after a series of sample extraction procedures can be determined by various offline analytical instruments, such as ion chromatography (IC)/liquid chromatography (LC), gas chromatography-mass spectrometry (GC-MS), and inductively coupled plasma-mass spectroscopy (ICP-MS) (Kim et al. Citation2003; Suzuki et al. Citation2010; Zhang et al. Citation2010). However, it should also be noted that it is difficult to accurately reconstruct chemical constituents and the mixing state of single dust particles by only measuring their bulk chemical composition. In addition, these methods cannot be used to capture temporal variations of properties of dust particles experiencing a rapid aging process, nor to identify volatile species in dust particles due to possible evaporative artifacts. The use of single particle mass spectrometry has provided a tremendous amount of information on chemical constituents of single dust particles in real time (Sullivan et al. Citation2007a, Citation2007b; Sullivan and Prather Citation2007; Sullivan et al. Citation2009). However, high vacuum condition and desorption and ionization of particles by a strong laser in the mass spectrometer technique would make it difficult to directly determine hygroscopic and volatile properties of dust particles. Thus, it is thought that direct measurements of the hygroscopicity and volatility of the dust particles will provide an additional understanding of their aging process and effect of the aged dust particles on the cloud formation.
Since Ca(NO3)2, CaCl2, H2SO4, and (NH4)2SO4 are hygroscopic, and minerals are nonhygroscopic (Sullivan et al. Citation2009), a real-time measurement of the hygroscopicity of size-selected dust particles will help identify the existence of hygroscopic species as a result of aging processes such as heterogeneous reaction, condensation, coagulation, and so on. The measurement of volatile organic compounds in the dust particles, which has been received little attention until now, will provide new insights into the mixing of dust particles with anthropogenic volatile organic compounds. In addition to the origin of dust particles, it is also thought that as the transport pathway of the dust particles vary in each AD event, this could possibly affect the aging process of dust particles (e.g., the difference between dust particles passing over industrial areas of eastern China or having a long residence time over an ocean without passing over an industrialized zone) (Duce et al. Citation1980; Husar et al. Citation2001; Sullivan et al. Citation2007a, Citation2007b; Sullivan and Prather Citation2007). However, limited studies have been conducted on the effect of transport pathway of the dust particles on their hygroscopicity and volatility.
In this study, a tandem measurement technique that combines a differential mobility analyzer (DMA) and a particle size distribution analyzer (PSD) with a heater and a humidifier placed between them was applied to determine the hygroscopicity and volatility of size-selected dust particles in almost real time (the volatility of dust particles was measured for the first time to our best knowledge) (Massling et al. Citation2007; Park et al. Citation2008; Kaaden et al. Citation2009). Organic carbon species can be considered candidates for the measured volatile species in this study, because (NH4)2SO4 or other inorganic species are not volatile at the heater temperature of 100°C in the tandem DMA-PSD system, and water in particles was removed before entering the system. During several AD events having different transport pathways, the hygroscopicity and volatility of the size-selected dust particles were measured to investigate the effect of transport pathway of the AD particles on their hygroscopicity and volatility (i.e., internal mixing via aging processes). The size-selection enabled us to infer the chemical composition of mainly dust particles, minimizing other types of particles. A database for the hygroscopic growth factor (HGF) and the shrinkage factor (SF) of chemical species that can be included in dust particles was also constructed based on a series of laboratory measurements. In addition, morphological and elemental analyses of dust particles were carried out using TEM/EDS.
EXPERIMENTAL
Dust particles passing through the PM10 inlet were dried to 8–10% RH using a series of diffusion driers. The size distribution of the dried particles (20 nm–10 μm) was continuously measured using a scanning mobility particle sizer (SMPS) (DMA: TSI 3081, CPC: TSI 3022A) (20–600 nm) and a PSD (TSI 3603) (0.6–10 μm). The hygroscopicity and volatility of the size-selected dust particles were measured using a tandem DMA-PSD system when AD events occurred (Kaaden et al. Citation2009; Massling et al. Citation2007). The tandem system mainly consists of a DMA, PSD, humidifier, and heated tube as shown in . Dust particles of a certain size (∼1 μm) were selected by the DMA (aerosol flow rate is 1.0 lpm and sheath air flow rate is 2.1 lpm). The selected dust particles were directed to a humidifier or a heated tube (i.e., they are exposed to an elevated RH or high temperature environment), subsequently being routed to the PSD (∼1 lpm) to measure particle size change under the elevated relative humidity (85% RH) or increased temperature (100°C). Due to the large size of the dust particles, a PSD able to measure particles of up to 10 μm in diameter was used in the tandem system. The HGF represents a ratio of the mode diameter (aerodynamic equivalent diameter) at 85% RH compared to dry condition (∼10% RH), while the SF is a ratio of the mode diameter at an increased temperature (100°C) relative to the room temperature (∼20°C) (i.e., relative change of the mode diameter (aerodynamic equivalent diameter) measured with the PSD under increased RH or heater temperature was used here). Since the PSD determined the aerodynamic equivalent diameter of the DMA-selected particles, multiple-charged particles were separated. The volume fractions of volatile species in size-selected particles can be calculated from the SF value, by assuming spherical particles (i.e., the volume fraction of volatile species (evaporated volume/total volume) = 1 – SF3). At the heater temperature of 100°C, organic carbon species including organic acids can be considered candidates for the volatile species because (NH4)2SO4 or other inorganic species are not volatile, and water in particles was removed before entering the tandem measurement system.
To construct a database of the hygroscopicity and volatility of possible candidate chemical species that can be included in dust particles, we produced various particles of a known composition. As shown in , these included 3Al2O3·2SiO2 (Sigma-Aldrich, USA), CaCO3 (Sigma-Aldrich, USA), CaSO4 (Sigma-Aldrich, USA), CaCl2 (Sigma-Aldrich, USA), Ca(NO3)2 (Oriental Chemical, Korea), NaCl (Junsei, Japan), MgCl2 (Sigma-Aldrich, USA), Fe2O3 (Sigma-Aldrich, USA), H2SO4 (Sigma-Aldrich, USA), C2H4O4 (Sigma-Aldrich, USA), C3H4O4 (Sigma-Aldrich, USA), C4H6O4 (Sigma-Aldrich, USA), C24H50 (Sigma-Aldrich, USA), C28H58 (Sigma-Aldrich, USA), and C32H66 (Sigma-Aldrich, USA). Particles were produced from a 0.1% (wt) DI water solution using a constant output atomizer (TSI 3076, USA) for water soluble species and using a furnace reactor (i.e., evaporation-condensation method) for water insoluble species.
TABLE 1 Hygroscopicity and volatility of possible candidate chemical species that can be included in the dust particles
To determine the morphology and elemental composition of single dust particles, particles less than 10 μm in diameter were collected on a TEM grid using a PM10 sampler. These samples were then analyzed by TEM (JEOL JEM-2100F) and EDS (OXFORD INCAx-sight). A number of particles (∼106 particles) having a size of ∼1 μm were examined in order to relate their morphological and elemental properties to the hygroscopicity and volatility of the size-selected dust particles. Morphological and elemental classifications were conducted for the dust particles collected during several AD events having different transport pathways. Due to low vacuum pressure (10−3 Torr) and strong electron beam (200 kV) in the TEM, volatile species will be evaporated. Thus, identification of volatile organic species would be difficult in the TEM.
Ambient measurements were conducted for four AD events occurring in the spring and winter in 2010 in urban Gwangju, Korea. For comparison, measurements were also conducted before and after the AD events. The current sampling site is located ∼7.6 km north of the downtown area and is influenced by various local sources, including traffic from a nearby highway (∼1.5 km from the site), combustion systems from residential and commercial areas (∼0.6 km from the site), and biomass burning from agricultural areas (∼0.8 km from the site). Dust particles were sampled through a PM10 inlet placed on the roof of a building (about 16 m above ground level). In addition, hourly PM10, O3, NO2, CO, and SO2 measurements including meteorological data (temperature, RH, wind direction, wind speed) were obtained at a nearby sampling site. The origin and pathway of air masses during the AD event periods were determined using an air mass backward trajectory analysis (http://www.arl.noaa.gov/ready/hysplit4.html). For this test, a 48 h air mass backward trajectory analysis was conducted, ending at heights of 100, 500, and 1000 m above ground level at the sampling site.
RESULTS AND DISCUSSION
Classification of AD Events
The AD events summarized in can be classified into weak, regular, and strong, based on the level of PM10. Here, AD2 was found to be the strongest AD event (the average PM10 was 1,239 μg/m3) among those examined. A northwestern wind with a high speed (3–5 m/s) was typically dominant when the AD events were observed in Korea. The high surface wind speed is an important factor for uplifting and carrying dust particles into air, which is followed by their long-range transport. During all AD events, the RH was typically lower than in non-events, suggesting that the dust-laden air masses had a low RH. A lower concentration of pollutant gases was also observed in AD events compared to non-events or local pollution events, probably due to the cleaning effect (i.e., a cold front with a high wind speed preceding the dust-laden air masses typically swept local pollutants away) (Zhang et al. Citation2010). A local pollution event can be defined as a particle event having a high PM2.5 and PM10, high concentration of CO, NOx, and SO2, and low wind speed (i.e., stagnant conditions) (Xie et al. Citation2005). It was found that as shown in , the three measured parameters (i.e., PM10, wind speed, and SO2) were able to clearly distinguish AD events (averages of PM10, wind speed, and SO2 are 539.2 μg/m3, 4.0 m/s, and 3.8 ppb, respectively) from non-events (averages of PM10, wind speed, and SO2 are 54.4 μg/m3, 1.8 m/s, and 3.0 ppb, respectively) and local pollution events (averages of PM10, wind speed, and SO2 are 76.6 μg/m3, 1.4 m/s, and 12.9 ppb, respectively). The SO2 was an important parameter to discriminate local pollution events from non-events.
TABLE 2 Average values of PM10, meteorological, and gas data during the periods of AD events
FIG. 2 Comparison of hourly average PM10, wind speed, and SO2 among AD events, non-events, and local pollution events.
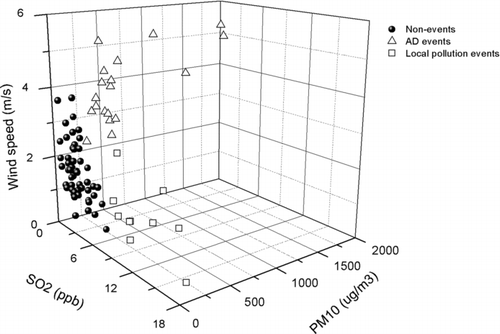
To determine the origin and transport pathway of the AD particles, air mass backward trajectory analyses during the AD events were conducted, as shown in . It was observed that all the AD particles tested here originated from the Gobi desert area (40–50oN, 90–110oE), though their transport pathway was different during each AD event. Depending on the transport pathway, we classified the AD events into “less polluted AD” and “highly polluted AD” events. In the less polluted AD events (AD1 and AD3), the air-mass originating from the Gobi desert arrived at the current sampling site without passing over major industrial areas (shaded area in ), whereas in the highly polluted AD events (AD2 and AD4) the air mass passed over a heavily industrialized zone before arriving at the sampling site, as shown in (Zhang et al. Citation2010). Also, in the highly polluted AD events, the air mass at an altitude of 100 m was directly transported to the sampling site from industrial areas (not from the Gobi desert). This lower air mass moved slow relative to the upper air mass and contained a significant amount of anthropogenic pollutants that were emitted from the industrial areas, and which were then mixed with the dust particles (Zhang et al. Citation2010). It was found that NO2, CO, and SO2 (i.e., anthropogenic air pollutants) concentrations in the highly polluted AD events were relatively higher than those in less polluted AD events in the same months, as indicated in . Variations of the hygroscopic and volatile properties of the dust particles in each type of AD event will be discussed in a subsequent section.
FIG. 3 Air mass backward trajectories during (a) less polluted AD events (AD1 and AD3) and (b) highly polluted AD events (AD2 and AD4) at heights of 100 m (triangle), 500 m (square), and 1000 m (circle) m above the sampling site (the shaded area represents major industrial areas near the air mass pathways).
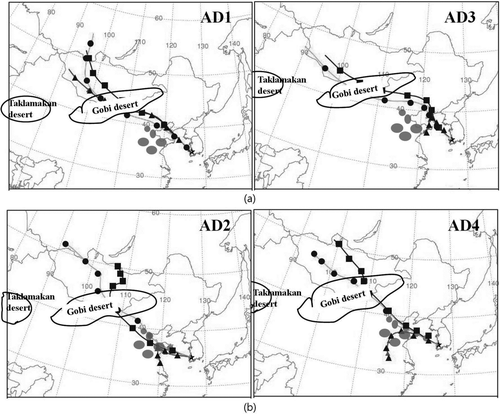
Number Size Distribution of AD Particles
Average number size distributions with their standard deviations during the current AD event and non-event are compared in . The data show that the number of 1 μm particles significantly increased by a factor of 2–5 during the AD event, with no significant change of submicrometer particles. The number distribution data suggest that the 1 μm particles during the AD event could mainly consist of long-range transported dust particles less affected by locally-produced particles near the sampling site. Thus, the 1 μm particles were selected by the first DMA in the tandem DMA-PSD system to obtain their hygroscopicity and volatility. Due to limitation of the DMA, we were not able to select particles larger than 1 μm. However, based on comparison of number size distributions between AD and non-events, the biggest number difference occurred around this particle size range. The surface area distribution with an assumption of spherical particles is shown in . The surface area of ∼2 μm particles also significantly increased by a factor of 2–5 during the AD events, suggesting that the dust particles provided a sufficient surface area so that other gases or particles can be mixed with them via condensation, reaction, or coagulation (Usher et al. Citation2003; Chou et al. Citation2003; Clarke et al. Citation2004; Sullivan et al. Citation2007a; Tobo et al. Citation2010).
Hygroscopicity and Volatility of AD Particles
The hygroscopicity and volatility of possible candidate chemical species that can be included in the dust particles were measured using the tandem technique, as shown in . In the table, only dicarboxylic acids (i.e., C2H2O4 and C4H6O4) and n-alkane species (e.g., C24H50) were found to be volatile at the heater temperature (100°C), and Ca(NO3)2, CaCl2, H2SO4, (NH4)2SO4, and sea-salts species (e.g., NaCl and MgCl2) were significantly hygroscopic at 85% RH. Our HGF data were consistent with values available in the previous literature (Chrzan et al. Citation1989; Gibson et al. Citation2006), thereby confirming the accuracy of this tandem measurement system to determine the HGF of particles.
TABLE 3 Relative fraction of dust particles having hygroscopic and volatile species
During the AD event periods, we continuously measured the hygroscopicity and volatility of 1 μm dust particles every 3 min. We found that the fraction of dust particles having hygroscopic and volatile species varied depending on the type of AD event (as defined previously), as shown in . In total, 26% of the 1 μm dust particles were found to have hygroscopic species in the highly polluted AD (i.e., 26% of measurements had HGFs greater than 1.00 ± 0.02) and 84% in the less polluted AD. Since the air mass was well mixed (i.e., quite constant wind direction) during AD events, the variation of HGF or SF would not be caused by the rapid change of air mass. Our data suggested that some of the dust particles (∼1 um) were pure minerals and others were aged. The dust particles could be aged in a different ways, thereby increasing their hygroscopicity both in the highly polluted and less polluted AD, as will be discussed later. In terms of volatility, 65% of the dust particles had volatile species (i.e., SF < 1.00 ± 0.03) in the highly polluted AD and 18% in the less polluted AD. The higher fraction of the dust particles having volatile species in the highly polluted AD occurred because the dust particles had a higher possibility to encounter air masses containing a significant amount of volatile carbonaceous species during transport in the polluted AD, as opposed to the transport pathway in the less polluted AD. In addition, the intensity of hygroscopicity and volatility that can be described by HGF and SF (i.e., volume fraction of volatile species), respectively, also varied depending on the type of AD event, as summarized in . All HGFs of 1 μm particles were smaller than 1.25, and no distinct groups of particles having different hygroscopicity under 85% RH were identified in our tandem data, suggesting that pure sea-salt particles, which were highly hygroscopic (HGF∼1.8), were not significantly externally-mixed with the 1 μm dust particles.
As shown in , the HGF in the less polluted AD was somewhat higher than that in the highly polluted AD. The dust particles could be aged in both, thereby leading to an increase in their hygroscopicity. Note that the residence time of dust particles over the open ocean (i.e., Yellow Sea) in the less polluted AD event was longer (∼13 h) than in the highly polluted AD (∼8 h), thus there was a higher possibility that the dust particles contained hygroscopic species originating from the ocean (Trochkine et al. Citation2003; Tobo et al. Citation2009; Tobo et al. Citation2010). Indeed, the heterogeneous reaction of dust particles with gaseous HCl over the ocean could be a possible pathway for the formation of hygroscopic CaCl2 (the existence of both Ca and Cl was also identified in our TEM/EDS data). Moreover, Na, Cl, and Mg were also observed in dust particles in the TEM/EDS data. Satellite data (Moderate Resolution Imaging Spectroradiometer (MODIS)) showed that AD air mass overlapped with clouds during transportation over ocean in the less polluted AD (http://modis.gsfc.nasa.gov/), suggesting that the cloud processing of dust particles with sea-salt components could be another important pathway for the existence of hygroscopic species in the dust particles (Andreae et al. Citation1986), leading to the higher HGF in the less polluted AD than the highly-polluted AD. A simple calculation indicated that the Brownian coagulation time of 1 μm dust particles with ∼2 μm sea-salt particles was about 2.6 weeks (i.e., much longer coagulation time compared to the lifetime of dust particles) (the coagulation time is defined as the time for the initial number concentrations of the dust particles to decrease by half, and the initial number concentrations of the dust particles and sea-salt particles are assumed to be 1,000 and 100 #/cm3, respectively) (Hinds Citation1999; Baron and Willeke Citation2001). However, the possibility of direct coagulation of dust particles and sea-salt particles cannot be completely ruled out (Zhang et al. Citation2003; Yamashita et al. Citation2005).
In the highly polluted AD, the dust particles were also aged by anthropogenic air pollutants, increasing their hygroscopicity. However, the intensity of hygroscopicity and the fraction of particles having hygroscopic species were smaller than those in less polluted AD. In this case, HNO3 produced from a polluted area could react with dust particles (i.e., CaCO3) to form hygroscopic Ca(NO3)2, contributing to the observed HGF. The reacted CaCO3 was identified in our TEM/EDS data, as will be discussed later. Although it was reported that the reaction probability of H2SO4 or SO2 in the dust particles (e.g., CaCO3) compared to HNO3 was relatively low (Underwood et al. Citation2001; Li et al. Citation2006; Song et al. Citation2007), the existence of S was observed in the TEM/EDS data, suggesting that hygroscopic S-containing species could be included in the dust particles. In a previous report, during the ACE-Asia field experience, Sullivan et al. (Citation2007a) found that the uptake of secondary acids onto dust particles depended on the mineralogical composition of the dust particles (i.e., nitrate species were accumulated onto Ca-rich particles such as CaCO3, whereas sulfate species accumulated onto Al2SiO5 particles). However, the addition of S was also observed in the Ca-rich particles with a residual left after evaporation of hygroscopic species, as seen in their TEM images. Zhang et al. (Citation2008) reported that H2SO4 molecules easily condensed onto aerosol particle surfaces (their sticking coefficient is very high) and were stabilized by water (i.e., irreversible condensation) independent of the morphology and chemical composition of the particles (the condensation rate only depends on surface area). As shown in , the 1–2 μm dust particles provided a much higher surface area compared to the fine particles (i.e., urban particles), such that the H2SO4 molecules could readily condense onto them. Also, the secondary sulfate or nitrate could be condensed on to the dust particles, contributing to the observed hygroscopic growth (Zhang and Iwasaka Citation1999; Li et al. Citation2006; Bauer et al. Citation2007; Tobo et al. Citation2010; Li et al. Citation2011). If CaSO4 existed in the dust particles as a result of the heterogeneous reaction of dust particles with SO2, their contribution to hygroscopic growth should be small because the HGF of CaSO4 was found to be low, as shown in . In addition, the reaction probability for the formation of CaSO4 is known to be low (Sullivan et al. Citation2009). Thus, we believe that the condensation of H2SO4 molecules or (NH4)2SO4 onto the dust particles could be an important pathway for the dust particles to have hygroscopic S-containing species. Also, secondary organic acids can be mixed with the dust particles to contribute the hygroscopic growth of the dust particles although their HGFs were typically low. This kind of internal mixing (i.e., aging) of the dust particles is very important for explaining the mass transfer process in atmospheric aerosols that consequently affect their optical properties (direct climate forcing) and CCN activity (indirect climate forcing) (Wang Citation2005; Karydis et al. Citation2011).
FIG. 6 TEM images and EDS spectra of dust particles: (a) (Si-rich (Al2SiO5), (b) Ca-rich (CaCO3), and (c) Fe-rich (Fe2O3) particles (the Cu peak might come from the copper TEM grid although we conducted background subtraction in the EDS spectra).
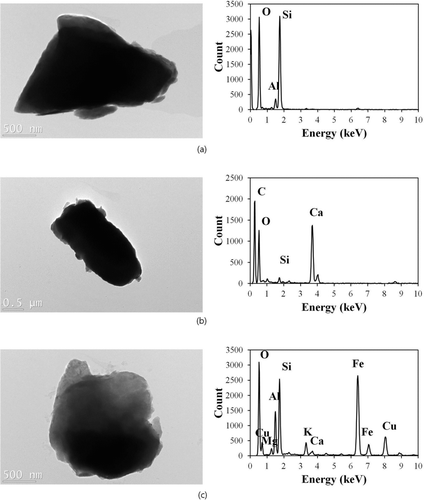
In addition to the atmospheric aging of dust particles by inorganic species, it was also reported that volatile dicarboxylic acids could be internally mixed with dust particles via photochemical chemistry and in-cloud processing (Sullivan and Prather Citation2007). confirms that some dust particles had volatile species. Note that no inorganic species that can be considered a viable candidate species for dust particles () were volatile at the heater temperature (100°C) of the tandem system (i.e., only volatile organic carbon species can be considered candidates for the evaporated species in this study, because inorganic species are not volatile at the heater temperature, and water in particles was removed via a series of diffusion driers before entering the tandem measurement system. When the volume fractions of volatile species in the dust particles were compared between the less polluted and highly polluted AD, the fraction in the highly polluted AD was found to be significantly higher (74% higher overall). This suggests that the AD particles passing over industrial zones included more volatile organic species. The volume fraction of volatile species in the 1 μm particles were also measured after AD events (i.e., non-events). It was observed that there was almost no volatile species in the 1 μm particles. As shown in , a clear difference was observed in volatility between the highly polluted and less polluted AD. This difference was thought to be due to the fact that there was only a limited source responsible for the addition of volatile organic species onto the dust particles in the transport pathway of the less polluted AD. We raised possibility that organic acids from the polluted area could react with dust particles or condense onto the dust particles (i.e., organic coating), and/or organic particles could potentially coagulate with the dust particles (Usher et al. Citation2003; Sullivan and Prather Citation2007). In this case, we are not able to rule out the possibility of coagulation between the dust particles and fine/ultrafine organic particles because the coagulation time between the 1 μm dust particles (∼1,000 #/cm3) and 0.1 μm urban particles (∼10,000 #/cm3) was about 3 h (Hinds Citation1999; Baron and Willeke Citation2001). Our TEM/EDS data also confirmed that different types of small particles were aggregated to the large dust particles, as will be discussed in the next section.
Morphological and Elemental Properties of AD Particles
Data for the hygroscopicity and volatility of size-selected (1 μm) dust particles suggest that a significant amount of dust particles was found to be aged by internally mixing with various hygroscopic and volatile species. As such, possible pathways for the formation of hygroscopic and volatile species could be proposed (i.e., formation of Ca(NO3)2, and CaCl2 by heterogeneous reaction, condensation of H2SO4 and (NH4)2SO4 onto dust particles, and cloud processing of dust particles with sea-salt species for the formation of hygroscopic species; heterogeneous reaction and condensation of organic acids with dust particles, and coagulation of dust particles with organic particles for the formation of volatile species). Additional morphological and elemental analyses were then carried out using TEM/EDS to support our hypotheses. For this task, 106 particles having a size of ∼1 μm collected during AD events were analyzed. A group of dust particles consisting mainly of mineral components (Si-rich (Al2SiO5), Ca-rich (CaCO3), and Fe-rich (Fe2O3) particles) (i.e., not aged dust particles) were identified as shown in . Those particles were collected during the less polluted AD. No evidence for aging (i.e., no inclusion of a significant amount of other inorganic and/or carbonaceous species, and no residuals after evaporation of hygroscopic or volatile species in their TEM images) was observed in such particles. TEM images for laboratory-generated pure mineral particles showed a similar result that residuals after evaporation were not observed at the current TEM condition. As such, these particles are deemed responsible for the nonhygroscopic and nonvolatile dust particles discussed in our previous section () (Wise et al. Citation2005, Citation2007).
We also identified a group of aged dust particles collected in both less polluted and highly polluted AD (). Under a high vacuum and strong electron beam, there was evidence of evaporation in some aged dust particles for hygroscopic or volatile species in the TEM images. Also, the aged dust particles contained a significant amount of other elements such as S, Na, Mg, K, and Cl—in addition to mineral elements. The aged dust particles could be further classified as reacted dust, cloud-processed dust, and aggregated dust. The reacted dust particles had major elements of S, Ca+O without C (possibly Ca(NO3)2), or Ca+Cl (possibly CaCl2), in addition to mineral components, and had evidence of evaporation of hygroscopic or volatile species (i.e., residuals after their evaporation in the TEM), as shown in . This type of particles represents aged dust particles as a result of reaction (e.g., CaNO3 or CaCl2 formation) or condensation (e.g., H2SO4 or (NH4)SO2)).
The cloud-processed dust particles had major elements of Na, Mg, and Cl (i.e., sea-salt species), in addition to minerals, and had evidence of evaporation of hygroscopic species in their TEM images (). In the previous section, we posited that the inclusion of sea-salt species in the dust particles could be caused by cloud processing. The existence of multiple sea-salt elements and their inhomogeneous distribution in the dust particles with evidence of evaporation supports the claim that the cloud processing could be responsible for the formation of such hygroscopic species.
The aggregated dust particles included aged dust particles aggregated with small particles (). These particles displayed no residual in their TEM images after evaporation of hygroscopic or volatile species. The fraction of the aggregated dust particles was somewhat higher in the highly polluted AD. This raises the possibility that in addition to heterogeneous reactions or condensation processes, coagulation could play a role in the modification of the mixing state of dust particles, especially those encountering air masses in which small fine particles are abundant. Indeed, the cloud processing of dust particles with sea-salt species—rather than their direct coagulation—could also lead to aggregated particles, such as those grouped into the cloud-processed dust particles. Accurate quantification of each type of aged dust particles during specific AD events was not shown here due to the limited number of particles examined by the TEM/EDS to draw significant conclusions. This is the limitation of the TEM/EDS method to obtain a statistically significant amount of data. However, the TEM/EDS data still provided useful information on identification of various types of dust particles.
CONCLUSIONS
Measurements of hygroscopicity and volatility of the size-selected dust particles with morphological and elemental data showed that a significant amount of dust particles were aged with increasing hygroscopicity and volatility, and that the type of aged dust particles differed depending on their transport pathway. The volatile fraction in the dust particles was found to be significantly higher in the highly polluted AD, suggesting that the AD particles passing over industrial zones included more volatile organic species. Also, we found that the dust particles could be aged in both types of AD, leading to an increase in their hygroscopicity. The HGF in the less polluted AD was somewhat higher than that in the highly polluted AD because the cloud processing of dust particles with sea-salt components played an important role in the existence of hygroscopic species in the dust particles in the less polluted AD. The varying aging process has important implications for different roles of dust particles in CCN activation and radiation balance, and their effects on human health. Our data suggested that optical properties of such aged dust particles should be significantly different from original dust particles, which is fundamental to the assessment of the radiative forcing of dust aerosols, and that the coating or mixing of dust particles by hygroscopic species with increasing their solubility could cause a significant enhancement of the dust contribution to the global CCN concentration. Also, the presence of volatile carbonaceous species in the dust particles will provide additional insights into the mixing structure of the dust particles affecting human health.
Acknowledgments
This work was supported by the National Research Foundation of Korea grant funded by the Korea government (MEST) (No.2011–0015548) and (NRF-C1ABA001–2011-0021063). The authors would like to thank the ARL (http://www.arl.noaa.gov/ready/hysplit4.html) for providing air mass backward trajectory and the NASA for MODIS data (http://modis.gsfc.nasa.gov/).
REFERENCES
- Andreae , M. O. , Charlson , R. J. , Bruynseels , F. , Storms , H. , Van Grieken , R. and Maenhaut , W. 1986 . Internal Mixture of Sea Salt, Silicates, and Excess Sulfate in Marine Aerosols . Science , 232 : 1620 – 1623 .
- Baron , P. A. and Willeke , K. 2001 . Aerosol Measurement - Principles, Techniques, and Applications. New York. : John Wiley & Sons, .
- Bauer , S. E. , Mishchenko , M. I. , Lacis , A. A. , Zhang , S. , Perlwitz , J. and Metzger , S. M. 2007 . Do Sulfate and Nitrate Coatings on Mineral Dust have Important Effects on Radiative Properties and Climate Modeling? . J. Geophys. Res. D: Atmos. , 112 ( D06307 ) : 1 – 9 . doi: 10.1029/2005JD006977, 2007
- Buseck , P. R. and Pósfai , M. 1999 . Airborne Minerals and Related Aerosol Particles: Effects on Climate and the Environment . Proc. Natl. Acad. Sci. , 96 ( 7 ) : 3372 – 3379 .
- Chen , Y. S. and Yang , C. Y. 2005 . Effects of Asian Dust Storm Events on Daily Hospital Admissions for Cardio Vascular Disease in Taipei, Taiwan . J. Toxicol. Env. Heal. A , 68 ( 17–18 ) : 1457 – 1464 .
- Choi , H. , Shin , D. W. , Kim , W. , Doh , S. J. , Lee , S. H. and Noh , M. 2011 . Asian Dust Storm Particles Induce a Broad Toxicological Transcriptional Program in Human Epidermal Keratinocytes . Toxicol. Lett. , 200 ( 1–2 ) : 92 – 99 .
- Chou , C. C. K. , Chen , T. K. , Huang , S. H. and Liu , S. C. 2003 . Radiative Absorption Capability of Asian Dust with Black Carbon Contamination . Geophys. Res. Lett. , 30 ( 12 ) : 18 – 21 .
- Chrzan , K. , Pohl , Z. and Kowalak , T. 1989 . Hygroscopic Properties of Pollutants on HV Insulators . IEEE T Dielect. El In. , 24 ( 1 ) : 107 – 112 .
- Clarke , A. D. , Shinozuka , Y. , Kapustin , V. N. , Howell , S. , Huebert , B. Doherty , S. 2004 . Size Distributions and Mixtures of Dust and Black Carbon Aerosol in Asian Outflow: Physiochemistry and Optical Properties . J. Geophys. Res. D: Atmos. , 109 ( 15 ) : 1 – 20 . D15S09
- Cwiertny , D. M. , Young , M. A. and Grassian , V. H. 2008 . Chemistry and Photochemistry of Mineral Dust Aerosol . Annu. Rev. Phys. Chem. , 59 : 27 – 51 .
- Duce , R. A. , Unni , C. K. , Ray , B. J. , Prospero , J. M. and Merrill , J. T. 1980 . Long-Range Atmospheric Transport of Soil Dust from Asia to the Tropical North Pacific: Temporal Variability . Science , 209 ( 4464 ) : 1522 – 1524 .
- Gibson , E. R. , Hudson , P. K. and Grassian , V. H. 2006 . Aerosol Chemistry and Climate: Laboratory Studies of the Carbonate Component of Mineral Dust and Its Reaction Products . Geophys. Res. Lett. , 33 ( 13 )
- Hinds , W. C. 1999 . Aerosol Technology - Properties, Behavior, and Measurement of Airborne Particles , New York : John Wiley & Sons .
- Hong , Y. C. , Pan , X. C. , Kim , S. Y. , Park , K. , Park , E. J. Jin , X. 2010 . Asian Dust Storm and Pulmonary Function of School Children in Seoul . Sci. Total. Environ. , 408 ( 4 ) : 754 – 759 .
- Husar , R. B. , Tratt , D. M. , Schichtel , B. A. , Falke , S. R. , Li , F. Jaffe , D. 2001 . Asian Dust Events of April 1998 . J. Geophys. Res. D: Atmos. , 106 ( D16 ) : 18317 – 18330 .
- Hwang , Y. J. , Jeung , Y. S. , Seo , M. H. , Yoon , J. Y. , Kim , D. Y. Park , J. W. 2010 . Asian Dust and Titanium Dioxide Particlesinduced Inflammation and Oxidative DNA Damage in C57BL/6 Mice . Inhal. Toxicol. , 22 ( 13 ) : 1127 – 1133 .
- Ichinose , T. , Yoshida , S. , Hiyoshi , K. , Sadakane , K. , Takano , H. Nishikawa , M. 2008 . The Effects of Microbial Materials Adhered to Asian Sand Dust on Allergic Lung Inflammation . Arch. Environ. Con. Tox. , 55 ( 3 ) : 348 – 357 .
- Kaaden , N. , Massling , A. , Schladitz , A. , Müller , T. , Kandler , K. Schütz , L. 2009 . State of Mixing, Shape Factor, Number Size Distribution, and Hygroscopic Growth of the Saharan Anthropogenic and Mineral Dust Aerosol at Tinfou, Morocco . Tellus, Series B: Chem. Phys. Meteorol. , 61 ( 1 ) : 51 – 63 .
- Karydis , V. A. , Kumar , P. , D., B. , Sokolik , I. N. and Nenes , A. 2011 . On the Effect of Dust Particles on Global Cloud Condensation Nuclei and Cloud Droplet Number . J. Geophys. Res. , 116 ( D23204 ) doi: 10.1029/2011JD016283
- Kelly , J. T. , Chuang , C. C. and Wexler , A. S. 2007 . Influence of Dust Composition on Cloud Droplet Formation . Atmos. Envion. , 41 ( 14 ) : 2904 – 2916 .
- Kim , K. H. , Choi , G. H. , Kang , C. H. , Lee , J. H. , Kim , J. Y. Youn , Y. H. 2003 . The Chemical Composition of Fine and Coarse Particles in Relation with the Asian Dust Events . Atmos. Envion. , 37 ( 6 ) : 753 – 765 .
- Krueger , B. J. , Grassian , V. H. , Cowin , J. P. and Laskin , A. 2004 . Heterogeneous Chemistry of Individual Mineral Dust Particles from Different Dust Source Regions: The Importance of Particle Mineralogy . Atmos. Envion. , 38 ( 36 ) : 6253 – 6261 .
- Lack , D. A. , Quinn , P. K. , Massoli , P. , Bates , T. S. , Coffman , D. Covert , D. S. 2009 . Relative Humidity Dependence of Light Absorption by Mineral Dust After Long-Range Atmospheric Transport from the Sahara . Geophys. Res. Lett. , 36 ( L24805 ) doi: 10.1029/2009GL041002
- Levin , Z. , Ganor , E. and Gladstein , V. 1996 . The Effects of Desert Particles Coated with Sulfate on Rain Formation in the Eastern Mediterranean . J. Appl. Meteorol. , 35 ( 9 ) : 1511 – 1523 .
- Li , L. , Chen , Z. M. , Zhang , Y. H. , Zhu , T. , Li , J. L. and Ding , J. 2006 . Kinetics and Mechanism of Heterogeneous Oxidation of Sulfur Dioxide by Ozone on Surface of Calcium Carbonate . Atmos. Chem. Phys. , 6 ( 9 ) : 2453 – 2464 .
- Li , W. J. and Shao , L. Y. 2009 . Observation of Nitrate Coatings on Atmospheric Mineral Dust Particles . Atmos. Chem. Phys. , 9 ( 6 ) : 1863 – 1871 .
- Li , W. J. , Zhang , D. Z. , Shao , L. Y. , Zhou , S. Z. and Wang , W. X. 2011 . Individual Particle Analysis of Aerosols Collected Under Haze and Non-Haze Conditions at a High-Elevation Mountain Site in the North China Plain . Atmos. Chem. Phys. , 11 ( 22 ) : 11733 – 11744 .
- Massling , A. , Leinert , S. , Wiedensohler , A. and Covert , D. 2007 . Hygroscopic Growth of Sub-Micrometer and One-Micrometer Aerosol Particles Measured During ACE-Asia . Atmos. Chem. Phys. , 7 ( 12 ) : 3249 – 3259 .
- Murphy , D. M. , Cziczo , D. J. , Froyd , K. D. , Hudson , P. K. , Matthew , B. M. Middlebrook , A. M. 2006 . Single-Peptide Mass Spectrometry of Tropospheric Aerosol Particles . J. Geophys. Res. D: Atmos. , 111 ( 23 )
- Park , K. , Dutcher , D. , Emery , M. , Pagels , J. , Sakurai , H. Scheckman , J. 2008 . Tandem Measurements of Aerosol Properties - A Review of Mobility Techniques with Extensions . Aerosol Sci. Tech. , 42 ( 10 ) : 801 – 816 .
- Ramanathan , V. , Crutzen , P. J. , Kiehl , J. T. and Rosenfeld , D. 2001 . Atmosphere: Aerosols, Climate, and the Hydrological Cycle . Science , 294 ( 5549 ) : 2119 – 2124 .
- Ro , C. U. , Hwang , H. , Kim , H. , Chun , Y. and Van Grieken , R. 2005 . Single-Particle Characterization of Four “Asian Dust” Samples Collected in Korea, Using Low-Z Particle Electron Probe X-Ray Microanalysis . Environ. Sci. Technol. , 39 ( 6 ) : 1409 – 1419 .
- Satheesh , S. K. and Krishna Moorthy , K. 2005 . Radiative Effects of Natural Aerosols: A Review . Atmos. Envion. , 39 ( 11 ) : 2089 – 2110 .
- Shi , Z. , Shao , L. , Jones , T. P. and Lu , S. 2005 . Microscopy and Mineralogy of Airborne Particles Collected During Severe Dust Storm Episodes in Beijing, China . J. Geophys. Res. D: Atmos. , 110 ( 1 ) : 1 – 10 .
- Song , C. H. , Kim , C. M. , Lee , Y. J. , Carmichael , G. R. , Lee , B. K. and Lee , D. S. 2007 . An Evaluation of Reaction Probabilities of Sulfate and Nitrate Precursors onto East Asian Dust Particles . J. Geophys. Res. D: Atmos. , 112 ( 18 )
- Sullivan , R. C. , Guazzotti , S. A. , Sodeman , D. A. and Prather , K. A. 2007a . Direct Observations of the Atmospheric Processing of Asian Mineral Dust . Atmos. Chem. Phys. , 7 ( 5 ) : 1213 – 1236 .
- Sullivan , R. C. , Guazzotti , S. A. , Sodeman , D. A. , Tang , Y. , Carmichael , G. R. and Prather , K. A. 2007b . Mineral Dust is a Sink for Chlorine in the Marine Boundary Layer . Atmos. Envion. , 41 ( 34 ) : 7166 – 7179 .
- Sullivan , R. C. , Moore , M. J. K. , Petters , M. D. , Kreidenweis , S. M. , Roberts , G. C. and Prather , K. A. 2009 . Effect of Chemical Mixing State on the Hygroscopicity and Cloud Nucleation Properties of Calcium Mineral Dust Particles . Atmos. Chem. Phys. , 9 ( 10 ) : 3303 – 3316 .
- Sullivan , R. C. and Prather , K. A. 2007 . Investigations of the Diurnal Cycle and Mixing State of Oxalic Acid in Individual Particles in Asian Aerosol Outflow . Environ. Sci. Technol. , 41 ( 23 ) : 8062 – 8069 .
- Sun , Y. , Zhuang , G. , Wang , Y. , Zhao , X. , Li , J. Wang , Z. 2005 . Chemical Composition of Dust Storms in Beijing and Implications for the Mixing of Mineral Aerosol with Pollution Aerosol on the Pathway . J. Geophys. Res. D: Atmos. , 110 ( D24209 ) : 1 – 11 . doi: 10.1029/2005JD006054
- Suzuki , I. , Igarashi , Y. , Dokiya , Y. and Akagi , T. 2010 . Two Extreme Types of Mixing of Dust with Urban Aerosols Observed in Kosa Particles: ‘After’ Mixing and ‘On-the-Way’ Mixing . Atmos. Envion. , 44 ( 6 ) : 858 – 866 .
- Tobo , Y. , Zhang , D. , Matsukia , A. and Iwasaka , Y. 2010 . Asian Dust Particles Converted into Aqueous Droplets Under Remote Marine Atmospheric Conditions . Proc. Natl. Acad. Sci. , 107 ( 42 ) : 17905 – 17910 .
- Tobo , Y. , Zhang , D. , Nakata , N. , Yamada , M. , Ogata , H. Hara , K. 2009 . Hygroscopic Mineral Dust Particles as Influenced by Chlorine Chemistry in the Marine Atmosphere . Geophys. Res. Lett. , 36 ( L05817 ) doi: 10.1029/2008GL036883
- Trochkine , D. , Iwasaka , Y. , Matsuki , A. , Yamada , M. , Kim , Y. S. Nagatani , T. 2003 . Mineral Aerosol Particles Collected in Dunhuang, China, and their Comparison with Chemically Modified Particles Collected over Japan . J. Geophy. Res.-Atmos. , 108 ( D23 )
- Underwood , G. M. , Song , C. H. , Phadnis , M. , Carmichael , G. R. and Grassian , V. H. 2001 . Heterogeneous Reactions of NO2 and HNO3 on Oxides and Mineral Dust: A Combined Laboratory and Modeling Study . J. Geophys. Res. D: Atmos. , 106 ( D16 ) : 18055 – 18066 .
- Usher , C. R. , Michel , A. E. and Grassian , V. H. 2003 . Reactions on Mineral Dust . Chem. Rev. , 103 ( 12 ) : 4883 – 4939 .
- Wang , C. 2005 . A Modeling Study of the Response of Tropical Deep Convection to the Increase of Cloud Condensation Nuclei Concentration: 1. Dynamics and Microphysics . J. Geophys. Res. D: Atmos. , 110 ( 21 ) : 1 – 16 .
- Wise , M. E. , Biskos , G. , Martin , S. T. , Russell , L. M. and Buseck , P. R. 2005 . Phase Transitions of Single Salt Particles Studied using a Transmission Electron Microscope with an Environmental Cell . Aerosol Sci. Tech. , 39 ( 9 ) : 849 – 856 .
- Wise , M. E. , Semeniuk , T. A. , Bruintjes , R. , Martin , S. T. , Russell , L. M. and Buseck , P. R. 2007 . Hygroscopic Behavior of NaCl-Bearing Natural Aerosol Particles Using Environmental Transmission Electron Microscopy . J. Geophys. Res. D: Atmos. , 112 ( D10224 ) doi: 10.1029/2006JD007678
- Xie , S. , Yu , T. , Zhang , Y. , Zeng , L. , Qi , L. and Tang , X. 2005 . Characteristics of PM10, SO2, NOx and O3 in Ambient Air During the Dust Storm Period in Beijing . Sci. Total Environ. , 345 ( 1–3 ) : 153 – 164 .
- Yamashita , K. , Hayashi , M. , Irie , M. , Yamamoto , K. , Saga , K. Ashida , M. 2005 . Amount and State of Mineral Particles in the Upper Mixed Layer and the Lower Free Troposphere over Mt. Raizan, Southwestern Japan: Unmanned Airplane Measurements in the Spring of 2003 . J. Meteorol. Soc. JPN. , 83 ( 3 ) : 121 – 136 .
- Yang , C. Y. , Cheng , M. H. and Chen , C. C. 2009 . Effects of Asian Dust Storm Events on Hospital Admissions for Congestive Heart Failure in Taipei, Taiwan . J. Toxicol. Env. Heal. A , 72 ( 5 ) : 324 – 328 .
- Zhang , D. and Iwasaka , Y. 1999 . Nitrate and Sulfate in Individual Asian Dust-Storm Particles in Beijing, China in Spring of 1995 and 1996 . Atmos. Envion. , 33 ( 19 ) : 3213 – 3223 .
- Zhang , D. , Iwasaka , Y. , Shi , G. , Zang , J. , Matsuki , A. and Trochkine , D. 2003 . Mixture State and Size of Asian Dust Particles Collected at Southwestern Japan in Spring 2000 . J. Geophys. Res. D: Atmos. , 108 ( 24 ) ACH 9-1-ACH 9-12
- Zhang , R. , Khalizov , A. F. , Pagels , J. , Zhang , D. , Xue , H. and McMurry , P. H. 2008 . Variability in Morphology, Hygroscopicity, and Optical Properties of Soot Aerosols During Atmospheric Processing . Proc. Natl. Acad. Sci. , 105 ( 30 ) : 10291 – 10296 .
- Zhang , W. , Zhuang , G. , Huang , K. , Li , J. , Zhang , R. Wang , Q. 2010 . Mixing and Transformation of Asian Dust with Pollution in the Two Dust Storms over the Northern China in 2006 . Atmos. Envion. , 44 ( 28 ) : 3394 – 3403 .
- Zhou , M. , Okada , K. , Qian , F. , Wu , P. M. , Su , L. Casareto , B. E. 1996 . Characteristics of Dust-Storm Particles and their Long-Range Transport from China to Japan–Case Studies in April 1993 . Atmos. Res. , 40 ( 1 ) : 19 – 31 .