Abstract
Copyright 2012 American Association for Aerosol Research
INTRODUCTION
The measurement of electrical mobilities is an important source of empirical information about fine nanometer aerosol particles. The mobilities are converted to particle diameters by means of a mathematical model of the relationship between electrical mobility and particle size. The international standard ISO 15900 recognizes the Stokes-Cunningham-Knudsen-Weber-Millikan model (briefly the plain Millikan model)
The plain Millikan model is commonly accepted when considering sufficiently large particles. Complications come up in case of fine nanometer particles. Effect of the finite size of gas molecules will be discussed in next sections. Additionally, the concept of size is a subject of discussions because a fine nanometer particle does not have solid surface and its geometric size is a conventional parameter in the similar way as the diameters of atoms and molecules are conventional quantities in crystallography. Mason (Citation1984) recommended the mass diameter (6m/(πρ))1/3, which is a diameter of an imaginary equivalent sphere of the same mass m and of the density of ion or particle matter ρ. The density of ionic matter exceeds the density of condensed bulk matter about 1.9 times in case of the simple cubic lattice and about 1.35 times in case of the closest packing of equal rigid spheres. In the following, the diameter of a particle is expected to be defined as the mass
diameter.
Tammet (Citation1995) analyzed phenomena that are important when considering the sub-3 nm particles. Later, Li and Wang (Citation2003) performed careful kinetic calculations and proposed an updated model of mobility-size relationship. An alternative theoretical model was advanced by Shandakov et al. (Citation2005). However, Wang (Citation2009) pointed out that the key problem of transfer of the scattering law still has no theoretical solution and some parameters can be estimated only by fitting a model to the empirical data. The kinetic theory based theoretical models lead to complicated computations and to difficulties in interpretation. These models are mentioned in introductions of aerosol research papers but not used when processing the real measurements. Poor success of theoretical models in the practice of aerosol research motivates a search for simplified algorithms that should well approximate the measurements and the kinetic theory based models, and support simple interpretation when compared with the classic Millikan model. The new simple general model of size-mobility relation is proposed with the aim of developing convenient algorithms for processing measurements and effective graphic comparison of different specific models. The model is limited to singly charged spherical particles and does not allow taking into account the chemical composition of particles. Fitting the general model to the specific model by Tammet (Citation1995) is considered below as a technical example of the implementation of the new model.
THE CONSTANT-UPDATED MILLIKAN MODEL
The drag on a fine particle depends on the particle-molecule collision diameter. In studies of fine nanometer particles, the finite size of molecules cannot be ignored, and the Millikan equation is often updated, replacing the particle diameter with the sum of diameters of the particle and the gas molecule d + dg . The diameter of the gas molecule is expected to be constant and the model
The constant-updated Millikan model fails within the limit of molecular sizes. For example, the mobility of N2 + ions in nitrogen at 300 K and standard pressure is 1.86 ± 0.18 cm2V−1s−1 (Mason and McDaniel Citation1988). The diameter of a nitrogen molecule estimated according to the Loschmidt method or according to the diffusion coefficient is about 0.37 nm. EquationEquation (2) issues at d = dg = 0.37 nm the mobility of 3.8 cm2V−1s−1. The main reason for inconsistency is the neglecting of the polarization interaction between charged particles and gas molecules.
Fernandez de la Mora et al. (Citation2003) analyzed the measurements of particles of a diameter less than 10 nm and found a good agreement between measurements and the constant-updated Millikan model at dg = 0.53 nm, which remarkably exceeds the geometric diameter of gas molecules. Afterwards, Ku and Fernandez de la Mora (Citation2009) and Larriba et al. (Citation2011) compared the diameters and mobilities of particles down to the molecular sizes. Larriba et al. (Citation2011) concluded that the measurements in the diameter range of 1.5–2.7 nm are in excellent agreement with the constant-updated Millikan model at dg = 0.3 nm, which is less than the diameter of a nitrogen molecule. Quantitative estimating of the uncertainty of fine nanometer particle measurements is difficult, and the uncertainty of the above-mentioned results remained unknown.
THE FUNCTION-UPDATED MILLIKAN MODEL
The quantity that replaces d in the plain Millikan model can be called the effective Millikan diameter d M. The parameter d M – d is actually an empiric parameter that may differ from the geometric diameter of gas molecules. It will be called the diameter extension and denoted Δ = d M – d. Comparison of the experimental results by Fernandez de la Mora et al. (Citation2003) and Larriba et al. (Citation2011) enables speculation that the diameter extension may vary with the particle size as predicted by the kinetic theory based models. Dependence of the diameter extension on the particle size according to the measurements is illustrated in the supplemental information. The conclusion by Larriba et al. (Citation2011) about the constant value of Δ may tolerate the fitting of the variations into the limits of uncertainty.
The proposal of the present study is to consider the diameter extension as a variable quantity that depends on the particle diameter and, to a smaller extent, on the temperature of the bath gas Δ = f Δ (d, T). The function-updated Millikan model is written
If a table of measured mobilities of nanometer particles of well-known mass diameter d down to the molecular sizes is available, then the values of the diameter extension can be tabulated as
Δ ≈ f Δ (d, T), which can be suggested as a tool for processing the nanometer aerosol measurements.
NUMERICAL EXPERIMENTS
The function f Δ(d, T) is different for different gases. The experiments below expect the air as the bath gas: Δ = f Δair (d, T). A study of the function f Δair(d, T) requires a reference data table, where mobilities of nanometer particles of different size in the air are presented at different temperatures. Usage of measurements presented with reduced mobilities is limited to datasets wherein a correct inversion of applied reduction procedure is possible; see explanations in Tammet (Citation1998). The numerical experiments are carried out using a provisional reference table generated artificially using the model (Tammet Citation1995). Thus, the derived approximation of the function f Δair(d, T) should be considered as an example of implementation of the method, and adjustment of the approximation is required in future.
The provisional reference table consists of mobilities for 29 diameters from 0.7 to 3.5 nm with a step of 0.1 nm at 11 values of temperature from −50°C to +50°C with a step of 10 K. Some changes were made in the published procedure (Tammet Citation1995): two technical errors were corrected and the approximations of gas viscosity and free path were replaced with approximations acquired from ISO 15900. Parameters of the bath gas were limited with parameters of the air. The density of particle matter was expected to be a constant of 2 g cm−3 and it was excluded from the list of explicit arguments of the mobility. The Pascal code of the modified algorithm and additional explanations are presented in the supplemental information.
shows the dependence of the estimated Δ on the diameter at three values of the temperature. It is compiled according to EquationEquation (4) where the values of d and Z were taken from the reference table. The asymptotic value of Δ in the model (Tammet Citation1995) is 0.6 nm composed of 0.37 nm collision diameter of gas molecules and of 0.23 nm of extra distance caused by other effects. The value of Δ decreases in the range of sub-3 nm particles. This could partially explain differences in the estimates of the gas molecule diameter in experimental studies carried out with nanometer particles of different sizes.
FIG. 1 Diameter extension required to fit the updated Millikan model with the provisional reference table at p = 1000 mb. (Color figure available online.)
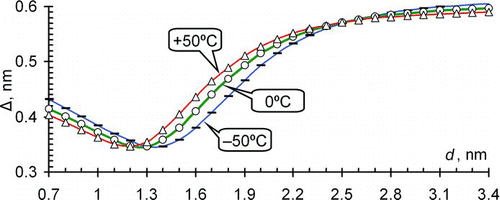
The curves in can be approximated with a simple expression
FIG. 2 Relative deviation of the approximate mobility from the reference data at p = 1000 mb. (Color figure available online.)
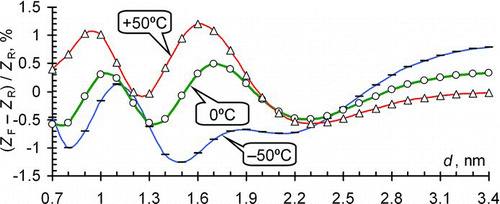
Neglecting of air pressure in EquationEquation (5) appeared to be justified. The curves in remained nearly invariant when varying the air pressure between 800 and 1200 mb. The maximum effect of pressure of about 0.012% occurs at a diameter of 1.2 nm.
Additional diagrams in the supplemental information show that the approximation (5) supports the inverted transformation from the measured mobility to the particle size well: the absolute deviation of the size between the proposed model and the reference data remains in an interval of −0.01 ⃛ + 0.01 nm in the size range of 0.7 … 2.8 nm, and the absolute relative deviation does not exceed 1% for all particles of diameter above 0.7 nm.
CONCLUSIONS
The classic Millikan equation turns out to be inaccurate when studying the finest charged nanometer particles or air ions. The alternative kinetic theory-based models, such as by Tammet (Citation1995), Li and Wang (Citation2003), and Shandakov et al. (Citation2005), did not have great success in aerosol measurements, and the Millikan model is authorized in the international standard ISO 15900.
The Millikan equation is often updated by replacing the particle diameter with an effective diameter d M interpreted as the sum of the diameters of the particle and the gas molecule d M = d + dg , where dg is expected to be a constant of the bath gas. Such a model can be called the constant-updated Millikan model. The quantity dg is an empiric parameter estimated by fitting its value to the measurements (Fernandez de la Mora et al. Citation2003). The results are different in different studies and seem to depend on the diameters of the measured particles.
The proposal of the present study is to consider the diameter extension Δ = d M – d as a variable that depends on the particle diameter and, to a smaller extent, on the temperature. The corresponding general model is called the function-updated Millikan model. Numerical experiments with provisional reference data show that the function Δ= f Δ(d, T) can be well approximated with a simple mathematical expression. Improved specific models for processing of aerosol measurements could be developed on the basis of the general model using enhanced reference data. However, the necessity to recalculate the size distributions after each improvement of the size-mobility model advocates the publishing of mobility distributions in case of precise measurements.
The function-updated Millikan model enables to use the common computational procedures with a minor modification, and enables interpretation in common terms explained in the standard ISO 15900.
uast_a_700740_sup_26739572.zip
Download Zip (246.8 KB)Acknowledgments
This research was supported by the Estonian Science Foundation through grant 8342 and the Estonian Research Council Targeted Financing Project SF0180043s08. The author would like to thank Professor Peter H. McMurry for fruitful discussions.
[Supplementary materials are available for this article. Go to the publisher's online edition of Aerosol Science and Technology to view the free supplementary files.]
REFERENCES
- Fernandez de la Mora , J. , de Juan , L. , Liedtke , K. and Schmidt-Ott , A. 2003 . Mass and Size Determination of Nanometer Particles by Means of Mobility Analysis and Focused Impaction . J. Aerosol Sci. , 34 : 79 – 98 .
- Kim , J. H. , Mulholland , G. W. , Kukuck , S. R. and Pui , D. Y. H. 2005 . Slip Correction Measurements of Certified PSL Nanoparticles Using a Nanometer Differential Mobility Analyzer (Nano-DMA) for Knudsen Number from 0.5 to 83 . J. Res. Natl. Inst. Stand. Technol. , 110 : 31 – 54 .
- Ku , B. K. and Fernandez de la Mora , J. 2009 . Relation between Electrical Mobility, Mass, and Size for Nanodrops 1–6.5 nm in Diameter in Air . Aerosol Sci. Technol. , 43 : 241 – 249 .
- Larriba , C. , Hogan , C. J. Jr. , Attoui , M. , Borrajo , R. , Fernandez Garcia , J. and Fernandez de la Mora , J. 2011 . The Mobility–Volume Relationship Below 3.0 nm Examined by Tandem Mobility–Mass Measurement . Aerosol Sci. Technol. , 45 : 453 – 467 .
- Li , Z. and Wang , H. 2003 . Drag Force, Diffusion Coefficient, and Electric Mobility of Small Particles. II. Application . Phys. Rev. , 68 : E061207
- Mason , E. A. 1984 . “ Ion Mobility: Its Role in Plasma Chromatography ” . In Plasma Chromatography , Edited by: Carr , T. W. 43 – 93 . New York and London : Plenum Press .
- Mason , E. A. and McDaniel , E. W. 1988 . Transport Properties of Ions in Gases , New York : John Wiley & Sons .
- Shandakov , S. D. , Nasibulin , A. G. and Kauppinen , E. I. 2005 . Phenomenological Description of Mobility of nm- and Sub-nm-Sized Charged Aerosol Particles in Electric Field . J. Aerosol Sci. , 36 : 1125 – 1143 .
- Tammet , H. 1995 . Size and Mobility of Nanometer Particles, Clusters and Ions . J. Aerosol Sci. , 26 : 459 – 475 .
- Tammet , H. 1998 . Reduction of Air Ion Mobility to Standard Conditions . J. Geophys. Res. Atmospheres. , 103 : 13933 – 13937 .
- Wang , H. 2009 . Transport Properties of Small Spherical Particles . Ann. N.Y. Acad. Sci. , 1161 : 484 – 493 .