Abstract
Research has shown that cigarette smoke changes chemically and physically after it is released into indoor air, that these changes can increase secondhand smoke (SHS) toxicity, and that acute exposures to even low levels of SHS increase the risk of cardiopulmonary disease. We designed a system to reproduce realistic SHS exposures in the laboratory for use in controlled human exposure studies. We generated cigarette smoke with a smoking machine, diluted it and conducted it through a 6 m3 stainless steel flow reactor at rates equivalent to the upper ranges of normal residential air exchange rates, to create aged cigarette smoke as a model for secondhand cigarette smoke. We observed that approximately 50% of the particle mass deposited within the system and that particle deposition percentage was higher when absorbent materials were placed within the system. The particle size ranges and deposition percentages, coefficients and velocities observed for this smoke aerosol are in good agreement with published values for SHS observed in residences and vehicles. This apparatus also permits the study of the physical and chemical interactions between SHS and indoor surfaces. The apparatus delivers stable aerosol concentrations to a human subject, which will permit accurate analysis of dose-response relationships in studies of the cardiovascular and respiratory effects of SHS exposure.
Copyright 2012 American Association for Aerosol Research
INTRODUCTION
Epidemiological studies have demonstrated a strong causal relationship between secondhand smoke (SHS) exposure and human disease (California Environmental Protection Agency, Office of Environmental Health Hazard Assessment et al. Citation2005; U.S. Department of Health and Human Services Citation2006). However, the mechanisms by which SHS exposure causes diseases other than cancer are still unclear. SHS is a complex and dynamic mixture made up of particles and gases that includes smoke from the burning end of the cigarette (sidestream smoke) and exhaled mainstream smoke (U.S. Department of Health and Human Services Citation1986). Recent evidence, from our laboratory and others, has shown that SHS undergoes numerous chemical and physical changes after it is released into the air. Nicotine and other semivolatile organic compounds in smoke adsorb to surfaces where they can react with oxidant gases present in SHS and ambient air to form new chemical compounds (Singer et al. Citation2002, Citation2003; Destaillats et al. Citation2006; Schick and Glantz Citation2007; Sleiman, Destaillats et al. Citation2010; Sleiman, Gundel et al. Citation2010; Petrick et al. Citation2011).
Subchronic and chronic exposure studies using laboratory animals have demonstrated that, when quantified on the basis of particulate material mass, aged cigarette smoke is more toxic to the respiratory epithelium than fresh smoke (Schick and Glantz Citation2006). Thus, studies that use freshly generated cigarette smoke may not capture the health effects caused by SHS in the real world. We created an aged-cigarette-smoke generation system to reproduce human exposures to secondhand cigarette smoke in the real world as accurately as possible in the laboratory. Controlled exposure studies are an important tool for assessing the acute health effects of exposure to pollutant exposures in humans and the biological mechanisms underlying these health effects. By using human subjects, it is possible to eliminate the doubt and dispute involved when animal exposure data are used to set exposure limits for humans. Previous human exposure studies have used two essential methods to generate SHS: human smokers (in the laboratory, public places or in homes), and fresh smoke from cigarette smoking machines. Both methods have significant drawbacks. Human smokers in the laboratory setting can only smoke so many cigarettes per hour and per day, which limits the range of smoke concentrations and the duration of potential exposures. Exposures in public places are hard to control and accurately monitor. Few researchers have used smoking machines in ways that allow for the normal smoke aging and chemical changes that occur in real environments where people are exposed to SHS. Since aging can increase SHS toxicity (Schick and Glantz Citation2006), we built a novel system to generate aged cigarette smoke for controlled human exposure studies.
The goals of this study were to twofold:
1. | To assess whether the new system accurately reproduces the air exchange rates, particle size profiles, particle deposition decay rates, and deposition velocities that are seen in real indoor environments where smoking is permitted. | ||||
2. | To test the effects of surface complexity on particle deposition within the system. |
We chose five target smoke concentrations: 100, 300, 600, 900, and 1800 μg m−3, to represent the range of concentrations encountered in areas where people are actively smoking (California Environmental Protection Agency, Office of Environmental Health Hazard Assessment et al. Citation2005), plus one higher concentration. The surfaces tested for effects on particle deposition were: stainless steel, 3MM chromatography paper, and 100% cotton terry cloth fabric. These materials were chosen because they are encountered in homes and businesses and offer a range of surface roughness and absorptive capacity.
The deposition constant β and deposition velocity VD are descriptions of particle behavior that have been measured extensively in chambers and indoor spaces. The deposition constant β expresses all factors influencing particle loss except ventilation, including loss from Brownian and turbulent diffusion and gravimetric settling, thermophoretic deposition due to temperature gradients between the surface and the bulk aerosol flow, size and geometry of the test chamber, electrostatic forces, surface roughness, and amount of mixing (Lai Citation2004). Deposition velocity VD is the effective velocity with which particles migrate to a surface, and does not depend on enclosure geometry. We calculated the deposition constant β and deposition velocity VD so we could compare particle behavior in our system to published values in the literature.
MATERIALS AND METHODS
Smoke Aging System
The design and operating criteria for our system included the ability to
1. | Achieve stable particle concentrations between 100 and 2000 μg/m3 | ||||
2. | Age smoke for 60 min | ||||
3. | Continuously and accurately monitor particle concentrations to ensure subject safety |
The system comprised ():
1. | An automated smoking machine | ||||
2. | A dilution system using filtered and conditioned air | ||||
3. | Stainless steel ducting to transport the aerosol | ||||
4. | A 6-m3 stainless steel aging chamber | ||||
5. | Sampling sites at four locations in the system | ||||
6. | An exposure chamber and exposure hood for the human subject | ||||
7. | Air velocity control and data acquisition systems |
Cigarette Smoke Generation and Dilution
Cigarette smoke was generated using a model TE-10z smoking machine (Teague Enterprises Inc., Woodland, CA). The cigarettes were Marlboro Red hard pack (Philip Morris Co., Richmond, VA), purchased by the carton on the open market and stored at –20°C prior to use. They were conditioned according to ISO Method #15592–2:2001(International Organization for Standardization Citation2001) and smoked according to ISO Method #15592–2:2001(International Organization for Standardization Citation2000).
The dilution system was designed to produce final particle concentrations between 100 and 2000 μg m3–1, without the use of inline fans or other devices that might change the temperature or chemical composition of the aerosol. Primary dilution was done using an air amplifier (model CDF-200h, Vaccon Co. Medway, MA) that admitted a fraction of the total smoking machine exhaust into the exposure system. Secondary dilution was effected by injection of the partially diluted smoke from the air amplifier into a stream of 200 L min−1 conditioned and filtered ambient outside air, generated by the UCSF Human Exposure Chamber HVAC system (Aris et al. Citation1993). It took an average of 3 min for the smoke to move from the cigarette, through the smoking machine, primary dilution, and secondary dilution to site 1. All input air, except the makeup air for the smoking machine, was HEPA and charcoal filtered.
Aging Chamber
The diluted smoke aerosol was aged in a custom-built 6-m3 stainless steel chamber. To promote mixing, three 1.40 m × 1.52 m stainless steel baffles were installed in a staggered configuration chamber () and two interior mixing fans (1/4 DC Fan Motor, Fasco Inc., distributed as Grainger # 2M167) were installed through shaft seals in the roof. The total interior surface area of the aging chamber is 35.4 m2, the volume is 6.0 m3, and the surface-to-volume ratio is 5.9 m−1.
Sampling Sites
Smoke samples were collected at four points in the system to test the effects of aging and surface deposition on smoke composition:
1. | Within the duct, just after secondary dilution (pre-aging) | ||||
2. | Within the duct, just after the aging chamber (post-aging) | ||||
3. | Within the duct, just before the exposure chamber (pre-subject) | ||||
4. | Within the exposure hood (subject). () |
Particle samples collected at sites 1, 2, and 3 employed custom-built sampling segments with three parallel stainless steel sampling nozzles with fittings for 6.4 mm (¼″) Teflon tubing (two # NS-8, 6.4 mm ID, and one #NS-16, 12.7 mm ID; Apex Instruments Inc., Fuquay-Varina, NC, USA). Particle samples at site 4, within the exposure hood, were collected through a 4″ segment of ¼″ Teflon tubing.
Air Velocity Control and Data Acquisition
Air flow through the ducting and aging chamber was controlled with a manual ball valve upstream of the secondary dilution site (). Air velocity was monitored via two air velocity transmitters (#641-b, Dwyer Instruments, Michigan City, IN, USA) located in the ducting; one just before the aging chamber and the other just before the human exposure chamber where the subject would sit (). A data acquisition system (Model CFP-1808, National Instruments, Austin, TX) was used to collect data from the air velocity transmitters and particle monitors and a custom LabView (Version 8.5, National Instruments, Austin, TX, USA) software program was developed to monitor, record, and process data for the system.
Exposure Hood and Human Exposure Chamber
After exiting the aging chamber, smoke was transported to the human exposure chamber where the exposure hood was located. The human exposure chamber is a 2.5 m × 2.5 m × 2.4 m adapted walk-in refrigerator with stainless steel interior (model W00327–3R; Nor-Lake, Hudson, WI, USA), and computer-controlled temperature, humidity, and ventilation. Air exchange rate in the HEC was 0.85 air changes per minute, the temperature was 22 ± 4°C and the relative humidity was 50 ± 10%. No human exposure experiments took place during the experiments discussed in this article. However, sample site 4 (subject) was within the breathing zone of the human exposure interface for this system: an adapted pressurized air purifying respirator hood (3M Airmate # BE-10–3, 3M, Inc., St. Paul, MN, USA) connected with an 1″ (25 mm) Teflon tube to the terminus of the diluted smoke duct.
Surface Material Preparation
Three separate aging chamber conditions were tested: stainless steel, stainless steel plus 3M chromatography paper and stainless steel plus 100% cotton terry cloth. The 3M chromatography paper and 100% cotton terry cloth specimens were cut to fit over the front and back side of all three aging chamber baffles, measuring approximately 1.4 m by 1.5 m per side, giving a surface area of 12.77 m2, which represents 38.8% of the total internal chamber surface area. They were mounted using stainless steel spring clips. An additional 200 cm2 of clear plastic adhesive packing tape was used to fasten sheets of paper together. Before usage, the terry cloth fabric was washed three times in a domestic washing machine using an unscented, enzyme-free laundry detergent (Country Save powdered laundry detergent, Arlington, WA, USA) in hot water with two rinses per cycle, and then washed once more with no detergent.
EXPERIMENTS
Leak Testing of Aging Chamber
Leak tests were performed on the aging chamber by sealing the outlet, pressurizing the chamber to 0.25 inches water-gauge (operating pressure), and measuring how much air input it took to maintain 0.25 inches pressure.
Laser Photometric Measurement of Particle Concentration
Particle concentration was measured continuously by laser photometers at site 1 (TSI SidepackTM AM510, TSI Inc., Shoreview, MN, USA) and site 3 (TSI Dusttrak IITM, model 8530,TSI Inc., Shoreview, MN, USA).
Gravimetric Measurement of Particle Concentration
The target particle concentrations were 100, 300, 600, 900, and 1800 μg m3–1. Because of initial problems with control of particle concentration, a total of six separate experiments for the stainless and paper aging chamber conditions and five experiments for the cloth aging chamber condition were necessary to reach all of the target concentrations. EPA PM2.5 filter membranes (47 mm diameter, #7592–104, Whatman Inc., NJ, USA) were pre-and postweighed on a Mettler MT-5 analytical microbalance (calibrated manually by certified Mettler technicians on a quarterly basis). Two filter membranes were placed in series in clean filter cassettes (#EW-06621–40, Cole-Parmer, Vernon Hills, IL, USA). Cassettes were then wrapped in aluminum foil to shield filters from light, and labeled. Samples were collected at 10 L min−1 until a minimum of 100 μg particulates per filter were collected. All samples were collected after the particle concentration had reached steady state throughout the system, defined as a steady particle concentration for a minimum of 15 min at site 3.
Background Particle Measurement
Before smoke was admitted to the system, we measured particle concentration gravimetrically and with the TSI SidepackTM AM510.
Particle Count and Size Distribution
Smoke particle counts and size distributions for a single experiment were obtained using an scanning mobility particle sizer (SMPS), (TSI Inc., St. Paul, MN, USA), employing a differential scanning mobility particle analyzer (Model 3071) with a condensation nuclei counter (model 3025). Sampling was conducted at all four sites. For these tests, sampling flow rates were set to yield distributions over the range of 16.5–604 nm, with a total sampling time of 90 s for each scan.
DATA ANALYSIS
SMPS
We used the count median diameter and geometric standard deviation (GSD) values generated by the SMPS to calculate the mass median and mean diameters, using the Hatch Choate equations. We used the particle number generated by the SMPS and the calculated mass mean diameter to calculate μg m3–1 using the following equation:
Particulate Matter Deposition Fraction
Barths’ number calculations were performed for the sampling rates and flow rates used. Calculated errors relative to the isokinetic condition were <50% for 5 μm particles (Clark Citation1998). Particle deposition fraction was calculated by gravimetrically measuring particle loss across the chamber. The total aerosol volume passing through the filter membranes was calculated as the average sampling flow rate (L min−1), as measured by a soap bubble spirometer (Gilian PN 800268, Sensidyne Corp., Clear Wakr, FL, USA), multiplied by the recorded duration of the experiment (min). The mass of particulate matter (μg) on the filter membranes was calculated as the difference between the final and initial filter weights. The average concentration (C 1 for pre-aging and C 2 for post-aging) throughout the duration of the test was calculated as the mass of particulate matter collected, divided by the volume passing through the filter. The mass fraction (%) of particulate matter deposited on surfaces between paired sampling sites, M dep, was found by computing:
We assumed that the total volume remained unchanged.
Deposition Decay Rate Loss Coefficient and Deposition Velocity
To determine the deposition coefficient, β, and deposition velocity, , a mass balance was developed describing flow in and out of the aging chamber. For this analysis, volumetric flow rate through the chamber was approximated to be the average recorded flow rate at the chamber inlet over the duration of the experiment, as recorded by LabView. Based on leak test findings and carbon monoxide measurements, the system was modeled with equal flow rates in and out of the aging chamber.
Because of the presence of the baffles and mixing fans, the aging chamber was assumed to be a well-mixed reactor with uniform smoke concentration C. Within the chamber, the most significant process that occurred was particle deposition to surfaces, which follows a first order rate of decay. Particle coagulation and secondary organic aerosol formation were also occurring, but neither would have significantly changed the overall particle concentration. Coagulation is a second order process that is most important at much higher particle concentrations. Secondary organic aerosol formation yields ultrafine particles with very low mass. The overall mass balance was written as:
The chamber volume was constant and thus it does not need to be shown within the derivative dC/dt. The volume flow rates (m3 s−1) into and out of the chamber were assumed equal, Q in = Q out (henceforth referred to as “Q”). Integration was performed to obtain the deposition rate in terms of the decay rate loss coefficient, β.
The system particle concentration was controlled to remain at steady state during the course of each experiment and the aerosol in the chamber was well mixed, so, C = C
out, and,
Solving for β yielded:
From this, the deposition velocity was determined:
Statistical Analyses
Statistical analyses were performed using Sigmaplot 10.0 software.
RESULTS
Leak Testing of Aging Chamber
Aging chamber leakage rate was 1.5–2.3% h–1.
Background Particle Concentration
Before smoke was admitted into the smoke aging system, the particle concentration was below the level of detection by gravimetric or laser photometric methods.
Effects of Aging on Particle Concentration
Particle concentration measurements at site 1 fluctuated with the burn cycle of the cigarette (). The 10-min cycle from ignition through extinguishing and reloading is reflected in the cyclical variation in particle concentration at site 1, located just after the secondary dilution. Smoke concentrations at site 3, located after the aging chamber and immediately upstream of the site for human exposures, did not show these cyclical variations. The aging chamber acted as a mixing chamber, averaging the cyclic input concentration into a uniform output concentration () by mixing the small inlet flow into a large volume.
FIG. 2 Particle concentration (mg m−3) at sites 1 and 3. Data from the AM510 and DustTrak instruments were adjusted to gravimetric equivalents (by multiplying by a factor of 0.27) to show particle deposition within the system. Mean concentration at site 1 was 1.98 mg m–3. Plateau concentration at site 3 was 1.14 mg m–3. Passage through the aging chamber reduced particle concentration fluctuation.
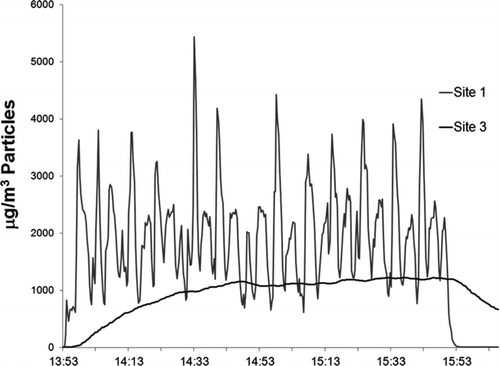
Smoke Particle Size Distribution
SMPS analysis, during a single experiment, demonstrated that the cigarette smoke particle size increased as the smoke aged. The geometric mean particle diameter at site 1 was 93 nm and the count median diameter was 94 nm. Particle size then increased gradually as the smoke passed through the aging system. At site 4, the particle mean and median particle sizes were ∼20% larger than at site 1. All particle distributions were slightly positively skewed (0.174–0.348). Testing, with one-way ANOVA, showed that the differences in particle size were statistically significant with F = 1567 and p < .001. Calculated mass concentrations show 50% deposition averaged over the three post-aging sites (sites 2, 3, and 4; )—in agreement with the deposition percentages measured gravimetrically. The loss of particles to deposition appears to have been greatest in the mid-range of particles sizes. See the supplemental information for histograms. The SMPS experiment was conducted with the terry cloth deposition substrate in the aging chamber.
TABLE 1 SMPS particle count, particle size and mass calculations
Particle Deposition Fraction and Surface Type
Particle deposition fractions measured in 20 experiments ranged from 23 to 78% (). The median deposition fraction for stainless steel was 43.0%, for 3MM paper 47.8% and for cloth 55.7%. Two-way ANOVA analysis of the effects of post-aging sampling location and deposition surface mounted in the smoke aging chamber revealed statistically significant differences between the three deposition surfaces (p = .010 and F = 5.261), but no difference between samples collected at sites 2 and 3 and no interaction between deposition surface and sampling location. Pairwise comparisons using the Holm–Sidak t-test showed significant differences between stainless and cloth surfaces (p = .0124) and cloth and paper surfaces (p = .005), but no significant difference between stainless and paper surfaces (p = .748) ().
FIG. 3 Particle concentration in the aged-cigarette-smoke generation system: results from 20 separate experiments testing the effects of aging, input particle concentration, and the addition of paper and cloth to the aging chamber, on particle deposition. Substantial fractions of the total particulate material in the smoke aerosol deposit between site 1 (before the aging chamber) and sites 2 and 3 (after the aging chamber). This result is consistent across particle input values and surface types.
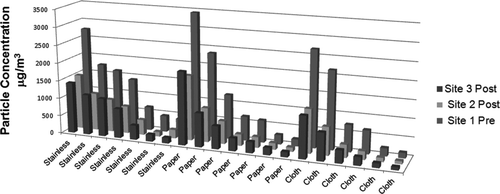
FIG. 4 Particle deposition fraction for three surfaces: median, interquartile range, maximum, minimum, and outlying values are shown. Particle deposition is significantly different between cloth surfaces and paper or stainless steel (control) surfaces.
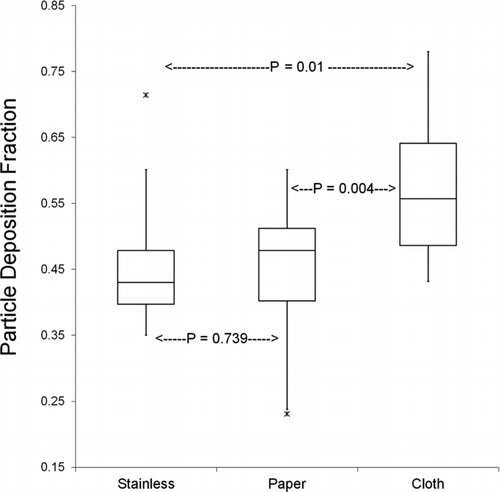
Particle deposition fraction was not dependent on particle concentration or flow rate. Linear regression analyses indicated that there was no statistically significant relationship between input smoke concentration or air flow rate through the system and the fraction of particulate matter deposited.
Deposition Decay Rate Loss Coefficient and Deposition Velocity
The deposition decay rate loss coefficients (β) and deposition velocities (VD ) are shown in . Although air velocity transmitters were functional and used in all 20 experiments, data logging via the LabView software program was only available for 12 experiments. Because of the high dependence of β and VD on volumetric flow rate, only trials that included continuous air velocity measurements were analyzed. The average flow rate was 89 L min−1 with standard deviation of 16 L min−1. These values translate to an average aging or residence time of 67 min. Velocity measurements at the chamber inlet (site 1) were chosen to represent the chamber flow rate assuming no leakage through the chamber. Two-way ANOVA analysis of the effects of surfaces mounted in the smoke aging chamber and post-aging sampling location on β and VD values calculated for sites 2 and 3 revealed a significant effect for surface (p = .008 and F = 6.287, but no effect of sampling location (p = .606, F = 0.276) and no interaction between surface and sampling location. Pairwise comparisons using the Holm–Sidak test showed significant differences between stainless and cloth surfaces (p = .009) and cloth and paper surfaces (p = .003), but no significant difference between stainless and paper surfaces (p = .538) ().
TABLE 2 Calculated values of deposition decay rate loss coefficient, β, and deposition velocity, VD , through aging chamber
DISCUSSION
Our primary findings are:
1. | Passage through the aging chamber reduced cyclic variation in smoke concentration | ||||
2. | Mean particle size increased slightly as the smoke aged | ||||
3. | Approximately 50% of the particle mass deposited within the aging chamber | ||||
4. | Particle deposition increased significantly when 100% cotton terry cloth was placed in the aging chamber, but not when 3M chromatography paper was placed in the chamber. |
Reduced Variation in Particle Concentration
Personal exposure to SHS normally includes significant variations in particle concentration due to changes in position of concentrated smoke plumes and changes in the number of cigarettes burning at a given time. However, it is preferable to use constant particle concentrations in human exposure studies. Constant concentrations permit direct correlation between exposure concentration and physiological effects without uncertainty over whether the peak concentration or the average concentration is driving the effects.
Particle Size
The particle size distributions of cigarette smoke aerosol in our system are in good agreement with the literature on SHS, aged sidestream smoke, and mainstream cigarette smoke. The mass median diameter (MMD) values reported in the literature for SHS range from approximately 200 to 500 nm and the GSD values from approximately 1 to 3 (Klepeis et al. Citation2003; Nazaroff and Klepeis Citation2003; Liu and Zhu Citation2010). We found MMDs ranging from 227 nm, in the freshly diluted smoke at site 1, to 298 nm, at the terminus of the aging system. Our GSDs ranged from 1.66 to 1.79. The parallel increase in count median and MMDs from site 1 (prior to aging) to sites 2, 3, and 4 (after aging) may reflect particle size increase through coagulation.
Deposition Fraction, Deposition Decay Rate Loss Coefficient and Deposition Velocity
Relatively few studies report particulate material deposition fractions, but a study by Philip Morris Co., using a 30 m3 chamber with 0.75 air changes per hour (ACH), reported deposition of 34% of sidestream cigarette smoke particulate matter when there were no furnishings, and 64% deposition when the room was fully furnished (Voncken et al. Citation1994). The median deposition percentages we observed were 43% for stainless steel, 48% for 3MM chromatography paper, and 56% for 100% cotton terry cloth.
The total particulate material deposition fractions, decay rate loss coefficients (β), and deposition velocities (VD ) measured in this study agree well with those reported in the literature. The surface-to-volume ratio in our system was 5.9 m−1 and the deposition decay rate-loss coefficients be ranged from 0.69 to 3.49 h−1. Using a 20 m3 experimental chamber with 0.03–0.1 ACH and a surface-to-volume ratio of 2.9 m−1, Klepeis et al. reported values for β ranging from 0.05 to 0.15 h−1 for smoke from cigarettes and cigars smoked by machine (Klepeis et al. Citation2003). Using a 30 m3 office with 3.6 ACH and a surface-to-volume ratio of 2 m−1, Ning et al. reported β values from 1.25 to 3.5 h−1 for cigarette smoke from human smokers (Ning et al. Citation2006).
Because the surface-to-volume ratio in this system (5.9 m−1) is higher than in residences and commercial spaces, deposition data from studies conducted in smaller chambers and in motor vehicles also provide appropriate comparisons. Ingebrethsen et al. found β values from 0.07 to 0.25 h−1 for sidestream smoke in a 0.45 m3 stainless steel cylinder with 0.60 ACH and a surface-volume ratio of 8 m−1 (Ingebrethsen and Sears Citation1989). Daher et al. found a β of ∼2 for cigarette smoke in a 1.5-m3 teflon-lined chamber with 1.5 ACH and a surface-volume ratio of 6 m−1 (Daher et al. Citation2010). Liu and Zhu found values ranging from 11 to 20 for cigarette smoke in a 2-m3 truck compartment with from 5.3 to 7.8 ACH and a surface-volume ratio of 8.1 m−1 (Liu and Zhu Citation2010). Our findings fit well in these ranges. Our findings for deposition velocity, VD , range from 3.22 × 10−5 to 1.26 × 10−4 ms−1 and are within the ranges found by Lai (Citation2004), for indoor spaces, indicating that the present aging system is representative of actual environments where smoking takes place.
Most previous studies of particle deposition delivered a high-concentration pulse into a closed system and measured loss of particles over time. Although these studies provide insight into general aerosol behavior and particle deposition, our system—a flow cell with continuous particle input and ventilation—is more representative of the residential and commercial buildings in which SHS exposure occurs. It will be useful in future studies testing chemical deposition and the effects of aging and accumulation on deposited smoke compounds.
Correlation Between Surface Type and Deposition
Significantly more particles were lost from the aerosol when terry cloth was added to the stainless steel aging chamber than when 3MM chromatography paper was added or when no additional materials were added. Hot-rolled 304 stainless steel is a smooth, impermeable surface with low chemical reactivity. The 3MM chromatography paper and cotton terry cloth are both permeable and absorb water from the air. The 3MM paper is a relatively smooth, uncoated paper with a cellulose content of ∼98% and a water content of ∼2% at 50% RH and 22°C. The paper was 0.034 mm thick and weighed ∼570 g/cm3. Terry cloth is a highly absorbent, pile loop textile with a cellulose content of ∼94% and a water content of approximately 5% (Destaillats et al. Citation2006). The terry cloth was 4.0 mm thick and weighed ∼2.2 gc m3–1.
Our findings are in accord with many other reports in the literature that have indicated that surface type has a substantial effect on deposition of particulate matter. In part, this depends on the friction velocity related to the surface roughness, but other factors may influence deposition as well. Abadie et al. (Citation2001) determined deposition velocities for glazing, linoleum, carpet, and smooth and rough wallpaper and found very high velocities for carpet, and low values for glazing and linoleum. This was attributed to an increase in the thickness of the particle concentration boundary layer adjacent to the rougher surfaces, which in turn decreased resistance to deposition and enhanced Brownian and turbulent diffusion.
Utility of SHS Generation System for Controlled Human Exposure Studies
This is the first system for controlled human exposure to cigarette smoke that permits control of the age of the smoke aerosol. Smoke age is a critical variable for two reasons. Most important is that the SHS that people are exposed to in the real world is aged. Ventilation rates in homes are quite low, ranging from 0.37–0.88 ACH on average (Repace et al. Citation2006; Yamamoto et al. Citation2010). This means that whenever someone smokes indoors, it takes anywhere from 15 min to 2.7 h for the smoky air to be replaced with fresh air. Ventilation rates in public spaces are supposed to be higher, but published data measuring ventilation rates in public spaces are lacking. Depending on how many people are smoking in a room and how often they smoke, real SHS exposures will consist of either a mixture of fresh and aged smoke or exclusively aged smoke.
The second reason smoke age is important is that the chemical composition of SHS changes over time and can become more toxic (Schick and Glantz Citation2006). The physical and chemical changes that occur as SHS ages are complex. Particulate material mass can be lost by direct mechanical deposition of particles to surfaces and by evaporation of volatile and semivolatile organic compounds from the particles. Particles can also collide and coagulate. Vapor phase compounds can be lost by adsorbtion to surfaces. Chemical reactions, in the aerosol phase and within the deposited and adsorbed phase on surfaces, can yield new chemicals which will partition to either the vapor or particle phase based on their vapor pressure. A significant percentage of the organic compounds in SHS have vapor pressures in the semivolatile range (10−2–10−8 kPa) (Rogge et al. Citation1994; Daisey Citation1999). This means that they are oily, waxy, or tar-like and more likely to deposit and adsorb onto interior surfaces than to stay in the air and be removed by ventilation (Wechsler and Nazaroff Citation2008). With observed particle deposition fractions ranging from 23 to 78%, our data suggest that deposition and adsorbtion are very important processes in SHS aging.
The role of evaporation is less clear. Ingebrethsen et al. reported decreases in sidestream smoke particle size during the first 1–50 min after emission of the smoke plume into the air (Ingebrethsen and Sears Citation1989) and attributed them to evaporation of volatile and semivolatile compounds from the particles. However, we observed no decreases in particle size in the smoke in our system. Evaporation is certainly occurring and increased evaporation and adsorbtion in the boundary layer nearest the surfaces may contribute to the increased particle loss with the terry cloth surfaces in the aging chamber. However, since we only observed increases in particle size and loss of total particulate mass, coagulation, and deposition appear to be the dominant mechanisms affecting particles in our system.
Implications for Thirdhand Smoke Pollution in Public Places
Thirdhand smoke is a new term used to describe the combination of SHS compounds that are adsorbed to surfaces, the compounds that are re-emitted back into the gas phase and the new chemical compounds that are formed by reaction of SHS compounds to yield secondary pollutants. Each cigarette emits 5–17 mg of particulate material into the environment (Daisey et al. Citation1991; Leaderer and Hammond Citation1991; Klepeis et al. Citation2003; Repace et al. Citation2006). Most residences and public spaces contain an abundance of complex, absorbent surfaces. Sensory perception and research both demonstrate that when people smoke repeatedly in a room, SHS chemicals build up on the furnishings and surfaces and are released back into the air (Singer et al. Citation2002). Depending on the vapor pressure and octanol-air partition coefficient of the compound, exposure to material that desorbs from smoke-polluted surfaces can make a significant contribution to total SHS exposure (Singer et al. Citation2004; Wechsler and Nazaroff Citation2008). However, when SHS is actively being generated, the SHS, not the emissions from adsorbed materials on surfaces, is the primary source of particles and smoke compounds in the air (Singer et al. Citation2002). Chemical reactions occurring in adsorbed nicotine and adsorbed SHS on surfaces can produce products including the tobacco specific-nitrosamines NNA and NNK, formaldehyde, N-methyl formamide, myosmine, ethyl pyridyl ketone, nicotinaldehyde, cotinine, and ultrafine particles (Destaillats et al. Citation2006; Petrick et al. Citation2010; Sleiman, Destaillats et al. Citation2010; Sleiman, Gundel et al. Citation2010; Petrick et al. Citation2011).
Limitations
In this study, we measured only the loss of particle mass from the aerosol, not the accumulation of particulate material on the inner surfaces of the smoke-aging system. If there are reactions occurring in the smoke aerosol or in adsorbed material that convert particle phase compounds into gas phase compounds with higher vapor pressures than the parent compounds, then measuring total particle deposition will overestimate the mass deposited within the chamber. The aged-smoke generation system will be useful for analyzing deposited material directly in future experiments.
CONCLUSIONS
The aged-cigarette-smoke generation system we describe can generate particle concentrations in the ranges observed in public spaces where smoking occurs (California Environmental Protection Agency, Office of Environmental Health Hazard Assessment et al. Citation2005). The ability to continuously monitor particle concentrations supports safe exposures of human subjects. The particle size ranges, deposition percentages, deposition coefficients, and deposition velocities observed for the smoke aerosol are all in good agreement with published values for cigarette smoke observed in residences and vehicles. The system is also able to deliver uniform concentrations to the subject, which will permit accurate analysis of dose-response relationships in acute and subchronic exposures of human subjects. This system can be used to accurately reproduce human exposures to secondhand cigarette smoke in a controlled laboratory environment. In future experiments, it may permit analysis of the dose-response relationship between SHS exposure and decrements in cardiovascular and respiratory health. The system could also be adapted, by running only clean air through it, to determine whether respiratory exposure to the compounds that desorb from SHS-contaminated surfaces will cause detectable changes in biomarkers of SHS exposure in human subjects.
Our research suggests that even when room ventilation is in the upper ranges observed in residential spaces, approximately half of the particulate mass from every cigarette deposits on room surfaces. This confirms research showing that, when smoking is permitted indoors, nonsmokers are exposed to the chemicals in cigarette smoke even when no one is actively smoking (Matt et al. Citation2004, Citation2008, Citation2011). Furthermore, our finding that particle deposition increased when complex, absorbent materials were placed in the system suggests that the complex and absorbent materials that are typical in indoor environments may serve as a sink for the accumulation of smoke toxins.
uast_a_708947_sup_27285867.zip
Download Zip (591.9 KB)Acknowledgments
S.F. Schick, K.F. Farraro, J. Fang, S. Nasir, H. Wong, and J. Balmes were supported by a grant from the Flight Attendants Medical Research Institute. D. Lucas was funded by the NIEHS (Award Number P42ES004705). This project would not have been possible without significant intellectual and practical contributions from the members of the Biological and Agricultural Engineering Shop at the University of California, Davis, particularly from Thomas W. Bell. Suzaynn F. Schick and Kathryn F. Farraro are joint first authors.
[Supplementary materials are available for this article. Go to the publisher's online edition of Aerosol Science and Technology to view the free supplementary files.]
REFERENCES
- Abadie , M. , Limam , K. and Allard , F. 2001 . Indoor Particle Pollution: Effect of Wall Textures on Particle Deposition . Build. Environ. , 36 : 821 – 827 .
- Aris , R. M. , Christian , D. , Hearne , P. Q. , Kerr , K. , Finkbeiner , W. E. and Balmes , J. R. 1993 . Ozone-Induced Airway Inflammation in Human Subjects as Determined by Airway Lavage and Biopsy . Am. Rev. Respir Dis. , 148 ( 5 ) : 1363 – 1372 .
- California Environmental Protection Agency, Office of Environmental Health Hazard Assessment, and Office of Air Resources Board . 2005 . Proposed Identification of Environmental Tobacco Smoke as a Toxic Air Contaminant , Oakland California : California Environmental Protection Agency .
- Clark , A. G . 1998 . Industrieal Air Pollution Monitoring: Gaseous and Particulate Emissions , New York : Springer .
- Daher , N. , Saleh , R. , Jaroodi , E. , Sheheitli , H. , Badr , T. , Sepetdjian , E. , Al Rashdi , M. , Saliba , N. and Shihadeh , A. 2010 . Comparison of Carcinogen, Carbon Monoxide, and Ultrafine Particle Emissions from Narghile Waterpipe and Cigarette Smoking: Sidestream Smoke Measurements and Assessment of Second-Hand Smoke Emission Factors . Atmos. Environ. , 44 ( 1 ) : 8 – 14 .
- Daisey , J. M. 1999 . Tracers for Assessing Exposure to Environmental Tobacco Smoke: What are They Tracing? . Environ. Health Perspect , 107 ( Suppl 2 ) : 319 – 327 .
- Daisey , J. M. , Mahanama , K. R. and Hodgson , A. T. 1991 . Toxic Volatile Organic Compounds in Simulated Environmental Tobacco Smoke . J. Expo. Anal. Env. Epid. , 8 ( 3 ) : 313 – 334 .
- Destaillats , H. , Singer , B. C. , Lee , S. K. and Gundel , L. A. 2006 . Effect of Ozone on Nicotine Desorption from Model Surfaces: Evidence for Heterogeneous Chemistry . Environ. Sci. Technol. , 40 ( 6 ) : 1799 – 1805 .
- Ingebrethsen , B. J. and Sears , S. B. 1989 . Particle Evaporation of Sidestream Tobacco Smoke in a Stirred Tank . J. Colloidal & Inter. Sci. , 131 ( 2 ) : 526 – 536 .
- International Organization for Standardization . 2000 . Routine Analytical Cigarette-Smoking Machine – Definitions and Standard Conditions , Geneva , , Switzerland : International Organization for Standardization .
- International Organization for Standardization . 2001 . “ Fine-Cut Tobacco and Smoking Articles Made from it – Methods of Sampling, Conditioning and Analysis ” . In Part 2: Atmosphere for Conditioning and Testing , Geneva, Switzerland : International Organization for Standardization . ISO Method #15592–2:2001
- Klepeis , N. E. , Apte , M. G. , Gundel , L. A. , Sextro , R. G. and Nazaroff , W. 2003 . Determining Size-Specific Emission Factors for Environmental Tobacco Smoke . Aerosol Sci. Technol. , 37 ( 10 ) : 780 – 790 .
- Lai , A. C. 2004 . Particle Deposition Indoors: A Review . Indoor Air , 12 ( 4 ) : 211 – 214 .
- Leaderer , P. P. and Hammond , S. K. 1991 . Evaluation of Vapor Phase Nicotine and Respirable Suspended Particle Mass as Markers for Environmental Tobacco Smoke . Environ. Sci. Technol. , 25 : 770 – 777 .
- Lipowicz , P. J. 1988 . Determination of Cigarette Smoke Particle Density from Mass and Mobility Measurements in a MIllikan Cell . J. Aerosol Sci. , 19 ( 5 ) : 587 – 589 .
- Liu , S. and Zhu , Y. 2010 . A Case Study of Exposure to Ultrafine Particles from Secondhand Tobacco Smoke in an Automobile . Indoor Air , 20 ( 5 ) : 412 – 423 .
- Matt , G. E. , Quintana , P. J. , Hovell , M. F. , Bernert , J. T. , Song , S. , Novianti , N. , Juarez , T. , Floro , J. , Gehrman , C. , Garcia , M. and Larson , S. 2004 . Households Contaminated by Environmental Tobacco Smoke: Sources of Infant Exposures . Tob. Control. , 13 ( 1 ) : 29 – 37 .
- Matt , G. E. , Quintana , P. J. , Hovell , M. F. , Chatfield , D. , Ma , D. S. , Romero , R. and Uribe , A. 2008 . Residual Tobacco Smoke Pollution in used Cars for Sale: Air, Dust, and Surfaces . Nicotine Tob. Res. , 10 ( 9 ) : 1467 – 1475 .
- Matt , G. E. , Quintana , P. J. , Zakarian , J. M. , Fortmann , A. L. , Chatfield , D. A. , Hoh , E. , Uribe , A. M. and Hovell , M. F. 2011 . When Smokers Move Out and Non-Smokers Move in: Residential Thirdhand Smoke Pollution and Exposure . Tob. Control. , 20 ( 1 )
- Nazaroff , W. and Klepeis , N. E. 2003 . “ Environmental Tobacco Smoke Particles ” . In Indoor Environment: Airborne Particles and Settled Dust , Edited by: Morawska , L. and Salthammer , T. Weinheim : Wiley-VCH .
- Ning , Z. , Cheung , C. S. , Fu , J. , Liu , M. A. and Schnell , M. A. 2006 . Experimental Study of Environmental Tobacco Smoke Particles Under Actual Indoor Environment . Sci. Total Environ. , 367 ( 2–3 ) : 822 – 830 .
- Petrick , L. , Destaillats , H. , Zouev , I. , Sabach , S. and Dubowski , Y. 2010 . Sorption, Desorption, and Surface Oxidative Fate of Nicotine . Phys. Chem. Chem. Phys. , 12 ( 35 ) : 10356 – 10364 .
- Petrick , L. M. , Svidovsky , A. and Dubowski , Y. 2011 . Thirdhand Smoke: Heterogeneous Oxidation of Nicotine and Secondary Aerosol Formation in the Indoor Environment . Environ. Sci. Technol. , 45 ( 1 ) : 328 – 333 .
- Repace , J. L. , Hyde , J. N. and Brugge , D. 2006 . Air Pollution in Boston Bars Before and After a Smoking Ban . BMC Public Health , 6 : 266
- Rogge , W. F. , Hildemann , L. M. , Mazurek , M. A. and Cass , G. R. 1994 . Sources of Fine Organic Aerosol. 6. Cigarette Smoke in the Urban Atmosphere . Environ. Sci. Technol. , 28 : 1375 – 1388 .
- Schick , S. and Glantz , S. A. 2006 . Sidestream Cigarette Smoke Toxicity Increases with Aging and Exposure Duration . Tob. Control. , 15 ( 6 ) : 424 – 429 .
- Schick , S. F. and Glantz , S. 2007 . Concentrations of the Carcinogen 4-(methylnitrosamino)-1-(3-pyridyl)-1-Butanone in Sidestream Cigarette Smoke Increase After Release into Indoor Air: Results from Unpublished Tobacco Industry Research . Cancer Epidemiol Biomarkers Prev. , 16 ( 8 ) : 1547 – 1553 .
- Singer , B. C. , Hodgson , A. T. , Guevarra , K. S. , Hawley , E. L. and Nazaroff , W. W. 2002 . Gas-Phase Organics in Environmental Tobacco Smoke. 1. Effects of Smoking Rate, Ventilation, and Furnishing Level on Emission Factors . Environ. Sci. Technol. , 36 ( 5 ) : 846 – 853 .
- Singer , B. C. , Hodgson , T. , A. and Nazaroff , W. W. 2003 . Gas-Phase Organics in Environmental Tobacco Smoke: 2. Exposure-Relevant Emission Factors and Indirect Exposures from Habitual Smoking . Atmospheric Environ. , 37 ( 39–40 ) : 5551 – 5561 .
- Singer , B. C. , Revzan , K. L. , Hotchi , T. , Hodgson , A. T. and Brown , N. J. 2004 . Sorbtion of Organic Gases in a Furnished Room . Atmospheric Environ. , 38 : 2483 – 2494 .
- Sleiman , M. , Destaillats , H. , Smith , J. D. , Liu , C.-L. , Ahmed , M. , Wilson , K. R. and Gundel , L. A. 2010 . Secondary Organic Aerosol Formation from Ozone-Initiated Reactions with Nicotine and Secondhand Tobacco Smoke . Atmospheric Environ. , In Press
- Sleiman , M. , Gundel , L. A. , Pankow , J. F. , Jacob , P. , Singer , B. C. and Destaillats , H. 2010 . Formation of Carcinogens Indoors by Surface-Mediated Reactions of Nicotine with Nitrous acid, Leading to Potential Thirdhand Smoke Hazards . Proc. Natl. Acad. Sci. USA , 107 ( 15 ) : 6576 – 6581 .
- U.S. Department of Health and Human Services . 1986 . “ The Health Consequences of Involuntary Smoking: A Report of the Surgeon General, US Dept of Health and Human Services ” . 332 New York : Public Health Service, Centers for Disease Control .
- United States Department of Health and Human Services . 2006 . The Health Consequences of Involuntary Exposure to Tobacco Smoke. United States Surgeon General reports. United States Surgeon General , 709 Washington , DC : United States Centers for Disease Control and Prevention .
- Voncken , P. , Stinn , W. , Haussmann , H. J. and Anskeit , E. 1994 . “ Influence of Aging and Surface Contact on the Composition of Cigarette Sidestream Smoke. Models for Environmental Tobacco Smoke, ” . In Toxic and carcInogenic Effects of Solid Particles in the Respiratory Tract , Edited by: Mohr , U. , Dungworth , D. L. , Mauderley , J. L. and Oberdorster , G. 637 – 641 . Washington , D.C. : International Life Sciences .
- Wechsler , C. J. and Nazaroff , W. 2008 . Semiolatile Organic Compounds in Indoor Environments . Atmospheric Environ. , 42 : 9018 – 9040 .
- Yamamoto , N. , Shendell , D. G. , Winer , A. M. and Zhang , J. 2010 . Residential air Exchange Rates in Three Major US Metropolitan Areas: Results from the Relationship Among Indoor, Outdoor, and Personal Air Study 1999–2001 . Indoor Air , 20 ( 1 ) : 85 – 90 .