Abstract
Pt-nanoparticles-laden graphene (GR) crumples were directly synthesized from a colloidal mixture of aqueous chloroplatinic acid (H2PtCl6) and graphene oxide (GO) sheets via aerosol spray pyrolysis (ASP). Effects of Pt content in the Pt/GR composite and temperature of heating zone on the particle morphology, diffraction pattern, and specific surface area were investigated. The morphology of Pt/GR was the shape of a crumpled sheet of paper and the average size of the composite was around 1.3 μm in diameter. As Pt content increased from 2 to 20 wt%, higher numbers of Pt nanoparticles are observed on the GR at higher Pt content and the specific surface area of the composite also increased from 122 to 146 m2/g. Also, the intensity of the GR peak decreased, but that of the Pt peak increased. As temperature increased from 500°C to 900°C, an increase of the particle size of Pt due to sintering was observed. Electrocatalytic application of the Pt/GR composites was examined through methanol oxidation reaction. The 20 wt% Pt/GR synthesized at 900°C showed higher performance on methanol oxidation than a commercial 20 wt% Pt/carbon black catalyst.
Copyright 2013 American Association for Aerosol Research
1. INTRODUCTION
Graphene (GR) exhibits a unique structure of two-dimensional nanosheet composed of hexagonally arrayed sp2-bonded carbon atoms with one-atomic thickness. GR sheets can be used as substrate materials of electrocatalyst for fuel cells due to their unique electric conductivity and structural characteristics resembling carbon nanotubes and carbon nanofibers (Brodie Citation1859; Croft Citation1960; Stoller et al. Citation2008; Rao et al. Citation2009; Segal Citation2009). GR sheets can be prepared by direct exfoliation of graphite sheets and the chemical reduction of graphene oxide (GO) sheets (Schniepp et al. Citation2006; Stankovich et al. Citation2007; Gao et al. Citation2009; Park and Ruoff Citation2009). GR, chemically synthesized from GO, is also called reduced graphene oxide (rGO).
Recently, GR-supported composites have attracted a great deal of attention for their enhanced material properties such as their electrical conductivity and chemical and electrical properties. Many works have been reported on the preparation of GR-based composites (Stankovich et al. Citation2006; Li and Kaner Citation2008; Si and Samulski Citation2008; Xu et al. Citation2008; Kou et al. Citation2009; Li et al. Citation2009; Williams and Kamat Citation2009; Yoo, Okata et al. Citation2009; Zhang et al. Citation2009). Previous studies were mostly conducted to synthesize the composites using various liquid-phase chemical methods such as chemical reduction with hydrazine, the impregnation–reduction method, the colloidal method, and the polyol method. However, such liquid-phase methods make the preparation of composites time consuming due to many kinds of chemical reaction steps, long reaction time, repeated washing, and long drying time. Besides the long processing time and steps, the as-prepared composites often show severe stacking or agglomeration. Severe stacking of GR sheets leads to a loss of the ultrahigh surface area advantage as a two-dimensional nanomaterial. Recently, a few efforts have been made to prevent GR sheets from severe stacking (Yoo, Kim, et al. 2008; Park et al. Citation2011; Li et al. Citation2012).
GO is known as a thermally unstable material that can easily undergo deoxygenation to be reduced upon thermal annealing (Rodriguez and Jimenez Citation1984; Kim, Cote, et al. Citation2010). During the thermal reduction of GO, some of the partially oxidized graphite turns into the fully oxidized form CO2 as well as the reduced form GR. Recently, it was reported that even a common camera flash is capable of photothermally reducing GO film at ambient conditions (Cote et al. Citation2009; Gilje et al. Citation2010). It was also reported that GR sheets decorated with nanoparticles of noble metals and transition metal oxides can be prepared through such one-step, domino-like redox reactions by flame treatment using GO film containing metal precursor and potassium salts (Kim, Luo, et al. Citation2010).
Such an unstable thermal property of GO was applied to synthesize GR sheets from GO sheets with no chemical reducing agents by spraying the colloidal droplets composed of GO sheets in the gas phase at high temperature (Luo et al. Citation2011). Spraying a dispersion of GO and nanoparticles yielded GR wrapped nanoparticles (Luo et al. 2012), which could turn into hollow GR capsules after removing the nanoparticles (Sohn et al. 2012). Furthermore, if colloidal droplets composed of GO sheets and platinum (Pt) precursor solution are subjected to the same thermal treatment, the Pt precursor and GO can be simultaneously reduced by the thermal decomposition. Then, Pt-nanoparticles-laden GR sheets can be synthesized in a very short time. However, there have been no reports on the synthesis of Pt/GR composite via aerosol process.
Aerosol spray pyrolysis (ASP) is a very fast and continuous aerosol process to fabricate self-assembled composites from the sprayed droplets of a colloidal mixture of nanomaterials. This method is also very advantageous in producing composites with a high production rate. It also takes a very short reaction time of several seconds and does not require any post heat treatment or purification. As shown in , a formation of Pt-nanoparticles-laden GR crumples via ASP can be described as follows. A colloidal mixture of GO sheets and Pt precursor solution is sprayed by a nebulizer, and the sprayed droplets are carried into the heating zone for the evaporation of the solvent. When confined in evaporating aerosol droplets, isotropic capillary compression can squeeze the GO sheets into GO or GR particles in the shape of a crumpled paper ball (Luo et al. Citation2011), and then the reduction of GO and Pt precursor occurs together in the heating zone. Finally, Pt-nanoparticles-laden GR crumples can be generated like a dried powder.
FIG. 1 Schematic diagram of the formation of Pt/GR composite from colloidal mixture of Pt nanoparticles and GO sheets by aerosol assisted self-assembly. (1) Colloidal of chloroplatinic acid and GO sheets, (2) crumpling of GO by capillary compression, (3) drying of Pt nanoparticles, and (4) thermal reduction of GO and chloroplatinic acid. (Color figure available online.)
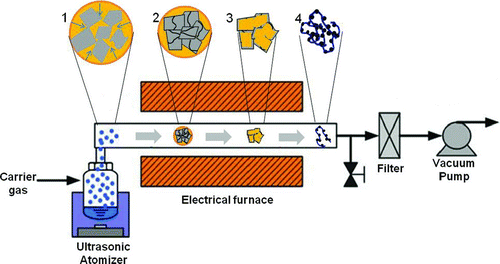
In the present study, we report facile direct synthesis of Pt-nanoparticles-laden GR crumples from sprayed droplets of a colloidal mixture of aqueous chloroplatinic acid (H2PtCl6) and GO sheets via ASP. The effect of Pt content in GR and operating temperature on the specific surface area, morphology, and crystallinity of the Pt/GR was investigated in order to characterize the material properties of Pt/GR composite. We also measured the degree of methanol oxidation by the as-prepared Pt/GR composite using an electrochemical reaction. A cyclic voltammogram (CV) measurement of methanol electro-oxidation was carried out.
2. EXPERIMENTAL
2.1. Synthesis of Pt/GR
GO was synthesized by the oxidation of graphite powder (Bay Carbon, SP-1) using NaNO3, H2SO4, and KMnO4 according to the Hummers’ method, where the micrographic powder was mixed with a strong oxidizing agent, filtered, washed, and dried (Hummers and Offeman Citation1958). Then, GO colloid was prepared with the application sonication until a homogeneous dark yellow dispersion was obtained. To prepare a colloidal aerosol precursor, an aqueous solution of H2PtCl6 (Aldrich) was added into the GO colloid and the mixture was vigorously agitated for homogeneous dispersion.
An experimental apparatus for the synthesis of Pt/GR composite consisted of an ultrasonic atomizer (UN-511, Alfesa Pharm Co.), an electrical tubular furnace, and a filter sampler (). The colloidal aerosol precursor solution was sprayed by a vibrator, which was operated at a frequency of 1.7 MHz, of the ultrasonic nebulizer. Droplets sprayed from the precursor solution were carried into an electrical tubular furnace by argon. The evaporation of water, thermal reduction of GO and Pt precursor, and the self-assembly between GR and Pt were occurred in series in the tubular furnace where the length and diameter of the heating zone were 30 cm and 25 mm, respectively. The composite synthesized by ASP was collected by a Teflon membrane filter under a vacuum.
2.2. Characterization
The morphology of as-prepared composites was observed with a transmission electron microscope (TEM, Philips CM12) and a field emission scanning electron microscopy (FE-SEM; Sirion, FEI). The specific surface area was characterized by the analysis of N2 adsorption–desorption isotherms. Data collection was conducted by a static volume method, using Quadrasorb Quantachrome equipment. The crystallinity was analyzed at room temperature by X-ray diffractometry (XRD, RTP 300 RC, Rigaku) with a CuKα target operated at 30 kV and 40 mA. The mean diameter was measured by the dynamic light scattering method using a particle size analyzer (Mastersizer 2000, Malvern).
2.3. Electrochemical Measurement
Electrochemical measurements were conducted at room temperature with a working Pt/GR electrode using an electrochemical interface instrument (VSP, Bio-Logics). All aqueous solutions were prepared with low resistance deionized water (∼18 MΩ). The CV measurement of methanol electro-oxidation was carried out in the mixture solution of 0.05 M H2SO4 + 1 M CH3OH within the potential range of 0–1.0 V (vs. saturated calomel electrode [SCE]) with a scan rate of 50 mV/s. Prior to the CV measurement, the electrolyte was purged with pure N2 gas for 30 min to remove dissolved oxygen.
3. RESULTS AND DISCUSSION
3.1. Synthesis of Pt/GR
shows FE-SEM and TEM images of the Pt/GR composite prepared with various Pt contents of (a1, a2) 5, (b1, b2) 10, and (c1, c2) 20 wt% while the GO concentration of the colloid was 0.5 wt%, temperature in the heating zone was 900°C, and the carrier gas flow rate was 1 L/min. The morphology of Pt/GR was the shape of a crumpled sheet of paper (Luo et al. Citation2011). The average size of the composite measured by the dynamic light scattering method was around 1.3 μm in diameter. The same morphology and average size of the Pt/GR composite prepared by ASP were observed regardless of variation of experimental conditions. TEM images reveal that Pt nanoparticles of less than 5 nm in diameter are uniformly deposited on the surface of GR crumples. Higher numbers of Pt nanoparticles are observed on the GR at higher Pt content.
FIG. 2 FE-SEM and TEM images of the Pt/GR composite prepared with various Pt contents of (a1, a2) 5, (b1, b2) 10, and (c1, c2) 20 wt% while the GO concentration of the colloid was 0.5 wt%, temperature in the heating zone was 900°C, and the carrier gas flow rate was 1 L/min. (Color figure available online.)
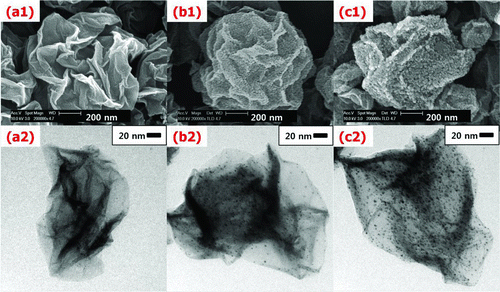
shows the diffraction of patterns of the as-prepared composites, which were synthesized under the same conditions as in . As the Pt content of the composites increased, the intensity of the GR peak decreased but that of the Pt peak increased. This indicates that diffraction pattern of Pt nanoparticles prepared at 900°C by ASP was crystalline phase. Crystallite sizes of Pt/GR composite were less than 4 nm when prepared at various Pt contents in the Pt/GR composite and the temperature of heating zone while GO concentration of the colloid was 0.5 wt% and the carrier gas flow rate was 1 L/min ().
TABLE 1 Crystallite size of the Pt/GR composite prepared at various Pt content in the Pt/GR composite and temperature of heating zone while GO concentration of the colloid is 0.5 wt% and carrier gas flow rate was 1 L/min
FIG. 3 The diffraction of patterns of the Pt/GR composite prepared with various Pt contents of (a) 5, (b) 10, and (c) 20 wt% Pt/GR, which were synthesized under the same conditions as in , and that of (d) 20 wt% Pt/carbon black.
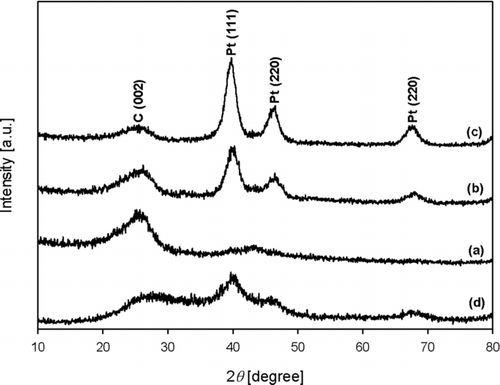
The specific surface area of the composite increased from 122 to 146 m2/g under the conditions of . The increase of the specific surface area is attributed to the increased number of Pt nanoparticles on the surface of GR. It is presumed that the Pt/GR composite has the structure of a stack of around 20 layers of monoatomic GR sheets since a theoretical surface area of unstacked GR is 2630 m2/g (Zhu et al. Citation2010).
The effect of temperature on particle morphology and the diffraction pattern of the composite were examined. As the reaction zone temperature increased from 500°C to 900°C at 20 wt% Pt, 0.5 wt% GO concentration of the colloid, and a 1 L/min carrier gas flow rate, an increase of the particle size of Pt due to sintering between particles was observed by FE-SEM and TEM analysis (). The diffraction patterns of the composites exhibit that the intensity of Pt was increasing because the crystalline phase of Pt was enhanced at higher temperature (). This indicates that Pt nanoparticles prepared at lower temperature have less crystallinity. Meanwhile, the intensity of GR was hard to see because the intensity of Pt was comparatively higher than that of GR. From the above results, it was found that ASP is an effective strategy to synthesize Pt-nanoparticle-laden GR sheets in the absence of reducing
agents.
3.2. Evaluation of Electrocatalytic Activity
The Pt/GR composite was explored for the oxidation of methanol as a fuel cell electrocatalyst. The cyclic voltammetry measurement of methanol oxidation reaction (MOR) was carried out with Pt/GR composite prepared at different temperatures. A commercial 20 wt% Pt/carbon black catalyst (Sigma Aldrich) was used as a reference for comparison. shows the current-potential curves of MOR between Pt/carbon black and Pt/GR composite prepared at different temperatures while the Pt/GR ratio was 0.2, GO concentration of the colloid was 0.5 wt%, and carrier gas flow rate was 1 L/min. The amount of metals was 1 mg for both catalysts per apparent area of electrode. The onset potential of methanol oxidation was 0.57, 0.56, and 0.60 V versus reference platinum electrode for Pt/carbon black, Pt/GR at 700 and 900°C, respectively. The electrocatalytic property of the composite prepared at 500°C was hard to be measured since Pt nanoparticles prepared at lower temperature have less crystallinity. The highest methanol oxidation was obtained from Pt/GR that was prepared at 900°C. The crystallinity and the morphology of Pt-nanoparticles-laden GR crumples may have a synergic effect for enhancing the methanol oxidation. The current density of Pt/GR prepared at 900°C was higher than the Pt/carbon black by about 3.82 mA/cm2. The difference in the catalytic activity of MOR between Pt/carbon black and Pt/GR is obviously due to the carbon substrate materials. The reverse anodic peak for MOR is attributed to the removal of the incompletely oxidized carbonaceous species formed in the forward scan (Mancharan and Goodenough Citation1992; Jang et al. Citation2010). Hence, the ratio of the forward peak current density (I f) to the reverse anodic peak current density (I b), I f/I b, can inform the catalyst tolerance to carbonaceous species accumulation. A low I f/I b ratio indicates poor oxidation of methanol to carbon dioxide during the anodic scan and excessive accumulation of carbonaceous residues in the catalyst surfaces (Yoo, Okata et al. Citation2009). The I f/I b ratio was estimated to be 1.30 and 1.96 for Pt/carbon black and Pt/GR at 900°C, respectively. It is, thus, found that a large amount of intermediate carbonaceous species for Pt/GR at 900°C was oxidized to carbon dioxide compared with that of Pt/carbon black. Therefore, this result reveals that Pt/GR composite prepared via ASP is a promising material for a Pt fuel cell catalyst.
FIG. 6 The current-potential curves of MOR between Pt/carbon black and Pt/GR composite prepared at different temperatures (a) 500°C, (b) 700°C, (c) 900°C, and (d) 20 wt% Pt/carbon black in the mixture solution of 0.05 M H2SO4 + 1 M CH3OH within the potential range of 0–1.0 V (vs. SCE) with a scan rate of 50 mV/s.
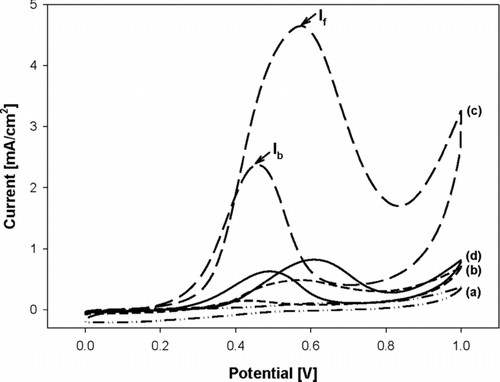
4. CONCLUSIONS
Pt-nanoparticles-laden GR crumples were successfully synthesized from sprayed droplets of aqueous colloidal solution of H2PtCl6 and GO via ASP. The morphology of Pt/GR composite was crumpled and Pt nanoparticles of less than 5 nm were well dispersed on the surface of the GR crumples. The number of Pt nanoparticles and specific surface area were proportional to the Pt content in the composite. The operating temperature could control the crystalline phase of Pt nanoparticles in the composite from amorphous to crystalline. The crystalline Pt/GR composite showed better catalytic performance for methanol oxidation than commercial Pt/carbon black electrocatalyst. Finally, ASP was found as a facile one-step process to synthesize Pt/GR composite without using any chemical reducing agent, and Pt/GR composite prepared by ASP is a prospective Pt fuel cell
catalyst.
Acknowledgments
This work was supported by the General Research Project of the Korea Institute of Geoscience and Mineral Resources funded by the Ministry of Knowledge Economy. Jiaxing Huang thanks the Alfred P. Sloan Foundation for a Sloan Fellowship and the Initiative for Sustainability and Energy at Northwestern (ISEN) for an Early Career Investigator Award.
REFERENCES
- Brodie , B. C. 1859 . On the Atomic Weight of Graphite . Philos. Trans. R. Soc. London , 149 : 249 – 259 .
- Cote , L. J. , Cruz-Silva , R. and Huang , J. 2009 . Flash Reduction and Patterning of Graphite Oxide and Its Polymer Composite . J. Am. Chem. Soc. , 131 : 11027 – 11032 .
- Croft , R. C. 1960 . Lamellar Compounds of Graphite . Quart. Rev. , 14 : 1 – 44 .
- Gao , W. , Alemany , L. B. , Ci , L. and Ajayan , P. M. 2009 . New Insights into the Structure and Reduction of Graphite Oxide . Nature Chem. , 1 : 403 – 408 .
- Gilje , S. , Dubin , S. , Badakhshan , A. , Farrar , J. , Danczyk , S. A. and Kaner , R. B. 2010 . Photothermal Deoxygenation of Graphene Oxide for Patterning and Distributed Ignition Applications . Adv. Mater. , 22 : 419 – 423 .
- Hummers , W. S. and Offeman , R. E. 1958 . Preparation of Graphitic Oxide . J. Am. Chem. Soc. , 80 : 1339
- Jang , H. D. , Chang , H. , Cho , K. , Kim , F. , Sohn , K. and Huang , J. 2010 . Co-Assembly of Nanoparticles in Evaporating Aerosol Droplets: Preparation of Nanoporous Pt/TiO2 Composite Particles . Aerosol Sci. Technol. , 44 : 1140 – 1145 .
- Kim , F. , Cote , L. J. and Huang , J. 2010 . Graphene Oxide: Surface Activity and Two-Dimensional Assembly . Adv. Mater. , 22 : 1954 – 1958 .
- Kim , F. , Luo , R. , Cruz-Silva , R. , Cote , L. J. , Sohn , K. and Huang , J. 2010 . Self-Propagating Domino-Like Reactions in Oxidized Graphite . Adv. Funct. Mater. , 20 : 2867 – 2873 .
- Kou , R. , Shao , Y. , Wang , D. , Engelhard , M. H. , Kwak , J. H. Wang , J. 2009 . Enhanced Activity and Stability of Pt Catalysts on Functionalized Graphene Sheets for Electrocatalytic Oxygen Reduction . Electrochem. Comm. , 11 : 954 – 957 .
- Li , D. and Kaner , R. B. 2008 . Graphene-Based Materials . Science , 320 : 1170 – 1171 .
- Li , Y. , Li , Y. , Zhu , E. , McLouth , T. , Chiu , C.-Y. Huang , X. 2012 . Stabilization of High-Performance Oxygen Reduction Reaction Pt Electrocatalyst Supported on Reduced Graphene Oxide/Carbon Black Composite . J. Am. Chem. Soc. , 134 : 12326 – 12329 .
- Li , Y. , Tang , L. and Li , J. 2009 . Preparation and Electrochemical Performance for Methanol Oxidation of Pt/Graphene Nanocomposites . Elecrochem. Comm. , 11 : 846 – 849 .
- Luo , J. , Jang , H. D. , Sun , T. , Xiao , L. , He , Z. Katsoulidis , A. 2011 . Compression and Aggregation-Resistant Particles of Crumpled Soft Sheets . ACS Nano , 5 : 8943 – 8949 .
- Luo , J. , Zhao , X. , Wu , J. , Jang , H. D. , Kung , H. H. and Huang , J. 2012 . Crumpled Graphene-Encapsulated Si Nanoparticles for Lithium Ion Battery Anode . J. Phys. Chem. Lett. , 3 : 1824 – 1829 .
- Mancharan , R. and Goodenough , J. B. 1992 . Methanol Oxidation in Acid on Ordered NiTi . J. Mater. Chem. , 2 : 875 – 887 .
- Park , S. and Ruoff , R. S. 2009 . Chemical Methods for the Production of Graphenes . Nature Nanotech. , 4 : 217 – 224 .
- Park , S. , Shao , Y. , Wan , H. , Rieke , P. C. , Viswanathan , V. V. Towne , S. A. 2011 . Design of Graphene Sheets-Supported Pt Catalyst Layer in PEM Fuel Cells . Electrochem. Commun. , 13 : 258 – 261 .
- Rao , C. N. R. , Sood , A. K. , Subrahmanyam , K. S. and Govindaraj , A. 2009 . Some Novel Attributes of Graphene . Angew. Chem. Int. Ed. , 48 : 7752 – 7777 .
- Rodriguez , A. M. and Jimenez , P. V. 1984 . Thermal Decomposition of the Graphite Oxidation Products . Thermochim. Acta , 78 : 113 – 122 .
- Schniepp , H. C. , Li , J. L. , McAllister , M. J. , Sai , H. , Herrera-Alonso , M. Adamson , D. H. 2006 . Functionalized Single Graphene Sheets Derived from Splitting Graphite Oxide . J. Phys. Chem. B , 110 : 8535 – 8539 .
- Segal , M. 2009 . Selling Graphene by the Ton . Nature Nanotech. , 4 : 612 – 614 .
- Si , Y. and Samulski , E. T. 2008 . Exfoliated Graphene Separated by Platinum Nanoparticles . Chem. Mater. , 20 : 6792 – 6797 .
- Sohn , K. , Na , Y. J. , Chang , H. , Roh , K. M. , Jang , H. D. and Huang , J. 2012 . Capillary Molding Route to Oil Absorbing Graphene Capsules . Chem. Comm. , 48 : 5968 – 5970 .
- Stankovich , S. , Dikin , D. A. , Dommett , G. H. B. , Kohlhaas , K. M. , Zimney , E. J. Stach , E. A. 2006 . Graphene-Based Composite Materials . Nature , 442 : 282 – 286 .
- Stankovich , S. , Dikin , D. A. , Piner , R. D. , Kohlhaas , K. A. , Kleinhammes , A. Jia , Y. 2007 . Synthesis of Graphene-Based Nanosheets via Chemical Reduction of Exfoliated Graphite Oxide . Carbon , 45 : 1558 – 1565 .
- Stoller , M. D. , Park , S. , Zhu , Y. , An , J. and Ruoff , R. S. 2008 . Graphene-Based Ultracapacitors . Nano Lett. , 8 : 3498 – 3502 .
- Williams , G. and Kamat , P. V. 2009 . Graphene-Semiconductor Nanocomposites: Excited-State Interactions between ZnO Nanoparticles and Graphene Oxide . Langmuir , 25 : 13869 – 13873 .
- Xu , C. , Wang , X. and Zhu , J. 2008 . Graphene-Metal Particle Nanocomposites . J. Phys. Chem. , 112 : 19841 – 19845 .
- Yoo , E. , Kim , J. , Hosono , E. , Zhou , H.-S. , Kudo , T. and Honma , I. 2009 . Large Reversible Li Storage of Graphene Nanosheet Families for Use in Rechargeable Lithium Ion Batteries . Nano Lett. , 8 : 2277 – 2282 .
- Yoo , E. , Okata , T. , Akita , T. , Kohyama , M. , Nakamura , J. and Honma , I. 2009 . Enhanced Electrocatalytic Activity of Pt Subnanoclusters on Graphene Nanosheet Surface . Nano Lett. , 9 : 2255 – 2259 .
- Zhang , Y. , Li , H. , Pan , L. , Lu , T. and Sun , Z. 2009 . Capacitive Behavior of Graphene-ZnO Composite Film for Supercapacitors . J. Eletroanalytic Chem. , 634 : 68 – 71 .
- Zhu , Y. , Murali , S. , Cai , W. , Li , X. , Suk , J. W. Potts , J. R. 2010 . Graphene and Graphene Oxide: Synthesis, Properties, and Applications . Adv. Mater. , 22 : 3906 – 3924 .