Abstract
The presence of actinomycetes in many workplaces and their role in the incidence of various respiratory symptoms remains poorly understood and underestimated. A sampling and culture-independent analysis method to measure airborne actinomycetes has yet to be developed and controlled bioaerosols are needed for laboratory investigations. In this article, the performances of a single-pass bubbling aerosolizer were characterized to evaluate the feasibility of generating actinomycetes from a liquid source and to confirm that viability of aerosolized entities was preserved. Six preparation protocols for liquid Thermoactinomycetes vulgaris cultures were compared in terms of culturable flora and total spores concentrations (culture and epifluorescence microscopy) and size distributions (optical counter and cascade impactor) of the bioaerosols generated. Using the best protocol, the generator's performances were then validated using three species: Thermoactinomyces vulgaris, Thermobifida fusca, and Streptomyces californicus. Bioaerosols contained a mixture of spores and mycelium and their properties were stable throughout generation (120 min) and were satisfactorily reproducible between runs. Depending on the species generated, the culturable concentrations measured were between 104 and 108 CFU.m−3, with corresponding total spore concentrations between 105 and 109 Spores.m−3. These concentrations cover the ranges measured in the workplace. The generator's flexibility should make it possible to produce bioaerosols with other actinomycetes species, and use them in laboratory trials with various objectives and constraints.
Copyright 2013 American Association for Aerosol Research
1. INTRODUCTION
Actinomycetes are gram positive bacteria found in the air of many workplaces. Actinomycetes form filamentous mycelium and small spores (aerodynamic diameters of 0.57 μm for Thermoactinomyces vulgaris, 0.85 μm for Streptomyces albus and 1.28 μm for Micromonospora halophytica—Reponen et al. [Citation1998]), which easily become airborne and can penetrate deep into the human lung.
Workplace exposure to actinomycetes bioaerosols potentially cause disease in humans, including hypersensitivity pneumonitis and chronic bronchitis (Fink Citation1978; Lacey and Crook Citation1988—health effects are further discussed in the online supplemental information at Section S1). Nevertheless, exposure to actinomycetes in workplaces and its contribution to the incidence of various respiratory symptoms remain poorly understood and underestimated.
A robust method to measure airborne actinomycetes, from sampling of the bioaerosol to its culture-independent analysis, has yet to be developed (Section S2 contains more information). To characterize and evaluate such a method, two types of complementary trials must be considered: laboratory tests with controlled experimental bioaerosols, and on-site tests in real conditions with complex and unstable bioaerosols.
Laboratory productions of experimental bioaerosols are aimed toward controllable reproduction of required sizes, biological agents, and concentrations (Griffiths and DeCosemo Citation1994). Methods to generate bioaerosols from dry or wet sources have been developed (Reponen et al. Citation1997) but few studies have comprehensively characterized actinomycete aerosols immediately following their generation (Table S2).
Most aerosolization techniques for actinomycetes use a dry dispersion technique. It consists of releasing microorganisms directly from the culture medium by applying a blowing air to the surface to detach spores and mycelium fragments. Microorganisms are most often generated from colonized gelose, but straw or other contaminated surfaces have been used. Most particles which detach from actinomycete cultures aerosolized under dry conditions, do so in a short time. This creates an initial burst of aerosolized particles, generally followed by a rapid decrease in number concentration; the bioaerosol is only stable over a short period. They are therefore not appropriate when stable, uniform concentrations over time are required (Jung et al. Citation2009). In addition, little has been published on the concentration levels obtained and the reproducibility of generation tests. Most data are available for the swirling-flow disperser, which was developed specifically for actinomycetes because small spores are more difficult to disperse from surfaces than fungal spores (Reponen et al. Citation1997).
To our knowledge, no work has been published describing the generation of actinomycetes bioaerosols from liquid cultures.
Wet dispersion techniques are frequently used in laboratory tests. While it remains the most widely cited technique, bioaerosol production by nebulizers poses some problems. A time-dependent increase in fine particles concentration is observed (Terzieva et al. Citation1996; Stone and Johnson Citation2002), what may be indicative of microorganism injury, such as cell membrane disruption, cell shrinkage, or leakage of intracellular components. Indeed, nebulization is known to produce effects which may be deleterious to microorganism integrity and viability, including: high shear forces, violent impacts between biological particles and the nebulizer surface, constant agitation of the liquid and recirculation of the non-aerosolized suspension which leads microorganisms to be repeatedly subjected to the stress of generation (Griffiths and DeCosemo Citation1994; Griffiths et al. Citation1996; Reponen et al. Citation1997; McCullough et al. 1998; Mainelis et al. Citation2001, Citation2005). Damage to cells appears from the first minutes of nebulization and results in a reduced concentration of culturable microorganisms (Stone and Johnson Citation2002). Repeated nebulization also increases the number of electrical charges carried by the microorganisms (Mainelis et al. Citation2001). The other commonly used wet bioaerosol generation technique is bubbling. Bubbles are formed by passing compressed air through a porous disc which can be submerged in the microbial liquid culture (Reponen et al. Citation1997; Ulevicius et al. Citation1997) or not (Mainelis et al. Citation2005; Simon et al. Citation2011). This method reduces stress and damage to microorganisms compared to nebulization (Reponen et al. Citation1997; Mainelis et al. Citation2001; Rule et al. Citation2009).
Considering drawbacks of dry dispersers and nebulizers and because viability is an important aspect of bioaerosol, we considered that a bubbling technique should be better adapted in order to generate actinomycetes. We hypothesized that a liquid sparging aerosolizer (LSA) bubbling generator (Mainelis et al. Citation2005; Simon et al. Citation2011) was a promising choice for aerosolizing repeatable particle size distribution of actinomycete bioaerosol from liquid cultures. The LSA has advantages for the dispersion of microorganisms, such as gentle aerosolization, no recirculation of the liquid culture and satisfactory stability and reproducibility of the bioaerosol properties. We also believed that the flexibility of the LSA constitutes an additional advantage. Several operating conditions (airflows, height of liquid film, composition or concentration of the liquid culture, porous disc characteristics, etc.) can easily be modified, allowing bioaerosols with controlled, adjustable properties to be generated.
Because no data is available with actinomycetes when generated using LSA, the main objectives of this article are as follows:
1. | To evaluate the feasibility of generating actinomycetes bioaerosols from a liquid source. | ||||
2. | To confirm that viability of actinomycetes is preserved when generated by LSA. | ||||
3. | To assess the performances of LSA when generating actinomycetes (values and reproducibility of culturable and total bacteria concentrations; stability of parameters over 120 min). |
2. MATERIALS AND METHODS
2.1. Description of the Setup for Bioaerosol Generation and Characterization
The test rig includes a bubbling-type generator and a chamber to condition and sample bioaerosols. Actinomycetes are dispersed by bubbling compressed air through a film of liquid bacterial culture. The functions of the generator and sampling chamber, as well as all the material used, were described in a previous article (Simon et al. Citation2011—brief description and figure are available in Section S4).
2.2. Preparation of Liquid Actinomycetes Cultures
Based on the preparation protocols described in the literature (Kawamoto et al. Citation1982; Gazenko et al. Citation1998; Hoskisson et al. Citation2000; AFNOR Citation2006), four protocols were tested to prepare the liquid actinomycetes cultures to be generated. The main steps of each protocol are summarized in .
FIG. 1 Summary description of the four protocols to prepare liquid actinomycetes cultures. (Color figure available online.)
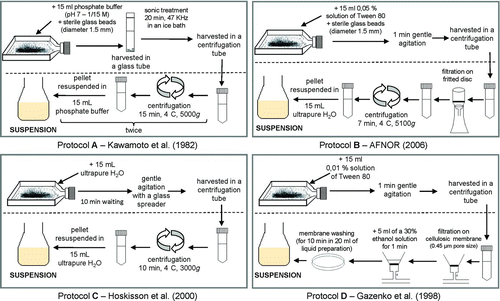
All methods include a phase to recover actinomycetes from culture flasks (BD FalconTM flasks—upper part of diagram), and a second phase to purify the biological harvested materials (lower part of diagram). The recovery step is performed after culture of the desired species on 90 mL Trypticase Soy Agar (TSA) gelose for 15 days at constant temperature (28°C for S. californicus and 50°C for T. vulgaris and T. fusca). To prevent drying of the agar plates, T. vulgaris and T. fusca, were grown in high relative humidity conditions (RH > 99%). The dehydrated medium (Bacto Laboratories Pty Ltd., Liverpool, NSW, Australia; cat number 211043, BBLTM) contained the following: 15.0 g pancreatic casein digest, 5.0 g papaic soybean meal digest, 5.0 g sodium chloride, and 15.0 g agar (per liter of water). After culture, each protocol uses a harvesting step to recover biological particles and transfer them to a centrifuge tube. Harvesting can be done with various liquids (water, phosphate buffer, Tween80) using a range of methods (manual agitation or scraper, with or without glass beads). To eliminate some elements (glass beads, gelose fragments, Tween80, phosphate buffer, ethanol), a purification phase is used involving washing the biological particles by centrifugation and/or filtering them on porous materials.
A second version of protocol A, named protocol A', was tested. Purification steps differ by the solution used to resuspend the centrifugation pellet (ultrapure sterile water in protocol A' instead of phosphate buffered solution). Similarly, a modified version of protocol B, named protocol B', was investigated, with different frit disc properties for filtration. Protocol B uses a glass frit (Duran®) of porosity 3 (16–40 μm), while protocol B' uses a frit of porosity 4 (10–16 μm).
The volume of the culture (ready for generation) depends mainly on the number of culture flasks from which actinomycetes were harvested and on the final volume of liquid used. Microorganisms recovered from six culture flasks were suspended in between 50 and 100 mL water, depending on tests. Liquid cultures were prepared on the day of generation.
2.3. Characterization of the Liquid Cultures and Bioaerosols Generated
The thermophilic actinomycete species Thermoactinomyces vulgaris (ATCCTM 43649) was used as the test organism to compare potential effects of different liquid culture preparations had on aerosol generation potential.
2.3.1. Comparison of Liquid Culture Preparation Protocols
We first compared six protocols: A, A', B, B', C, and D. For each protocol, a liquid culture was prepared (six culture flasks were harvested and suspended in 100 mL sterile ultrapure water), and the culturable and total actinomycetes concentrations were measured. Each liquid culture was then aerosolized. The culturable and total actinomycetes airborne concentrations, as well as the size distributions were measured simultaneously by parallel sampling from different probes in the sampling chamber. Bioaerosols were continuously generated in the same operating conditions: bubbling airflow QG = 4.0 L.min−1; entraining airflow QE = 20 L.min−1 at RH = 50 ± 2%; height of the liquid film H liq = 8 mm. For each protocol, measurements were performed in triplicate using three distinct liquid cultures, prepared, characterized, and generated on three different days.
2.3.2. Concentration Ranges and Reproducibility of the Bioaerosols Generated
To determine the airborne total spore and culturable actinomycetes concentrations ranges and reproducibility, liquid cultures were prepared using protocol B' (Section 4.3.4). Concentration values were adjusted by varying the bubbling airflow (0.3 ≤ QG ≤ 5 L.min−1) and/or by diluting the initial T. vulgaris liquid culture with ultrapure sterile water.
To improve reproducibility, we used a generation indicator, IG , calculated as follows:
Here, fD represents the initial dilution factor of the liquid culture to be generated. The value of fD = 1 when the microorganisms recovered from six flasks were suspended in 50 mL. fD = 0.5 when the initial preparation was diluted twofold (recovery of the six flasks in 100 mL). Intermediate values between 0.5 and 1 were obtained depending on the volume of water in which the harvested microorganisms were resuspended (between 50 and 100 mL). The choice and definition of the indicator IG will be further explained in Section 4.4.
For each IG value studied, airborne concentrations were measured three times (from three distinct liquid cultures prepared and generated on three different days).
2.3.3. Stability of the Bioaerosol Over 120 min Continuous Generation
Tests were performed to evaluate generator's capacity to maintain stable bioaerosol properties over 120 min. Optical counter real-time monitoring of size distribution, particle number concentration, and indicator IG were investigated. For 120 min, the operating conditions were left unchanged.
2.3.4. Bioaerosol Generation with Other Actinomycetes Species
Bioaerosols were also generated with T. fusca (ATCCTM BAA-629) and S. californicus (ATCCTM 3312). These strains were chosen because of their relevance to occupational hygiene (Section S5). Using these two strains, we wished to demonstrate, based on a restricted number of tests, that the method used to generate a T. vulgaris bioaerosol could be used for other species. The liquid cultures generated during these tests were prepared using protocol B'. Reproducibility of concentrations between generations or stability of bioaerosol parameters during generation were not characterized during these tests.
2.4. Sampling and Analysis Methods
2.4.1. Culturable Actinomycetes Concentrations in Liquid Culture
The culturable actinomycetes concentrations were determined using serial dilutions in tryptone-salt solution (AES Chemunex). Two petri dishes (TSA) were inoculated per dilution for three successive dilutions. S. californicus was incubated at 28°C for 72 h; T. vulgaris and T. fusca were incubated at 50°C for 72 h. Colonies were counted on each dish containing fewer than 300 colonies. Results were expressed as colony forming units per milliliter of liquid (CFU.mL−1). An isolated spore, strand or fragment of mycelium and agglomerate all present culturable entities which can lead to colony formation.
2.4.2. Total Actinomycetes Spore Concentrations in Liquid Culture
The total number of actinomycetes spores in a sample corresponds to all spores present, including dead spores. The analytical steps were based on a previously described protocol (Simon et al. Citation2011). Spores were counted using DAPI® (Sigma). First, an aliquot of suspension was mixed with DAPI® at 0.5 μg.mL−1 in sterile water and filtered under vacuum (Millipore, six-place stainless steel filter holder manifold) onto a 0.2 μm polycarbonate membrane (Millipore, IsoporeTM black membrane filter, 25 mm diameter). The membrane was rinsed with 15 mL sterile purified water, laid on a glass slide and air-dried in the dark. Membranes were mounted between slide and cover slip with paraffin oil. Total spores were counted using a fluorescence microscope (LEICA DM 2500 microscope at 1000× magnification over 20 to 30 evenly spaced fields, Histolab 7.4.0 Microvision). The microscope was connected to a camera (colour video camera qImaging IEEE 1394). Results were expressed as total spores (culturable + nonculturable + dead spores, isolated or agglomerated) per milliliter of liquid (Spores.mL−1). Strands/fragments of mycelium were not counted.
2.4.3. Culturable Actinomycetes Concentration in the Bioaerosol
The bioaerosol was sampled by connecting a cassette (Millipore®, 37-mm polystyrene 3-piece closed-face cassette) to one of the sampling probes in the chamber. The cassette contained a support pad (Millipore®, cellulose absorbent pad) and a filter (Whatman®, Nuclepore® polycarbonate membrane, 0.8 μm pore size). Sampling was performed over 50 min at 2 L.min−1 (pump Gilian®, GilAir-3).
Samples were analyzed immediately after sampling. A 10 mL extraction solution (Tween80 0.01%, peptone 0.1%, ultra-pure water) was introduced into the cassette before shaking for 20 min at 2000 rpm (Heidolph®, Multi-Reax® shaker). Analysis of the extract was as described for the liquid culture (Section 2.4.1). Results were expressed as colony forming units per cubic metre of air (CFU.m−3).
2.4.4. Total Actinomycetes Concentration in the Bioaerosol
The bioaerosol was sampled and biological particles were extracted from the cassette as described above (Section 2.4.3). For the lowest concentrations, the extract from several cassettes (two to five, sampled simultaneously on several probes) were pooled to increase the number of observable spores per microscope field. Analysis was as for the liquid culture (Section 2.4.2). Results were expressed as total spores per cubic metre of air sampled (Spores.m−3).
2.4.5. Particle Number Concentration and Particle Size Distribution in the Bioaerosol
OPC Grimm® G1109 was used to monitor in realtime the particle number concentration and the size distribution. This OPC works at a sampling airflow of 1.2 L.min−1 and classes particles according to their size (“optical” diameter, d opt) between 0.25 and 32 μm across 31 channels.
Size distribution of the culturable particles generated was assessed with a Sioutas® Cascade Impactor (SKC). It consists of four impaction stages (Whatman®, 25-mm Nuclepore® polycarbonate membrane, 0.8 μm pore size) and an outlet filter (Pall-Corporation, Port Washington, NY, USA; 37-mm GLA-5000 PVC membrane, 5.0 μm pore size). Sampling was performed at 9 L.min−1 (SKC, Leland Legacy®) over 40 min. The vertically positioned impactor was connected to one of the probes in the sampling chamber. Particles were separated in the following aerodynamic diameter ranges: <0.25, 0.25–0.5, 0.5–1.0, 1.0–2.5, and 2.5–10 μm (Misra et al. Citation2002). After sampling, the five filters were carefully recovered and individually placed in sterile 50 mL Greiner tubes. The biological particles collected were then recovered by washing each filter with 10 mL of extraction solution for 20 min with agitation at 2000 rpm. Each extract was then analyzed using a similar protocol to that described in Section 2.4.1. The collection supports in the impactor were not greased to avoid effects on recovery of the biological particles and analysis of the culturable flora.
3. RESULTS
3.1. Comparison of Protocols to Prepare Liquid Cultures
For each of the six protocols tested, the culturable and total T. vulgaris concentrations were measured (). The same characteristics were measured for the bioaerosols generated ().
FIG. 2 Culturable actinomycetes (▪) and total spore (▵) concentrations in (a) liquid cultures and (b) bioaerosols for the six culture preparation protocols (T. vulgaris). Error bars represent the 95% confidence interval of three repetitions.
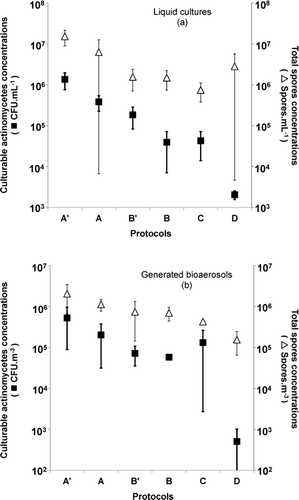
In the liquid cultures (), protocols A', A, and B' provided the highest mean culturable concentrations: 1.4 × 106, 3.8 × 105, and 1.8 × 105 CFU.mL−1, respectively. Protocols B, C, and D were all below 105 CFU.mL−1. Protocol D provided the lowest concentration. Mean total spore concentrations were ≈107 Spores.mL−1 for protocols A' and A, while protocols B', B, and C provided concentrations close to 106 Spores.mL−1. The 95% confidence interval values for protocols A and D were broader than for the other protocols tested.
Protocol A' provides the highest airborne culturable concentrations (), while protocol D provides the lowest. Protocols A', A, B', and B provide quite similar total spore concentrations, ≈106 Spores.m−3. The culturable T. vulgaris concentrations are generally over 105 UFC.m−3 for protocols A' and A, while they are almost all below 105 UFC.m−3 for protocols B' and B. The airborne culturable concentrations measured with protocols B' and B are quite reproducible, with narrower 95% confidence intervals than for the other protocols.
The results presented in show that the culturable actinomycetes and total spore concentrations measured in the liquid cultures or in the bioaerosol are affected by the protocol used.
3.2. Particle Size Distribution of the T. vulgaris Bioaerosol Generated
3.2.1. Optical Particle Counter Measurements
Examples of the size distribution of airborne T. vulgaris particles as a function of their optical diameter are presented in .
FIG. 3 Size distributions of the bioaerosol generated from T. vulgaris cultures prepared according to protocols (a) A' and (b) B' or (c) generated from S. californicus cultures prepared with protocol B'. Error bars represent the 95% confidence interval of three repetitions.
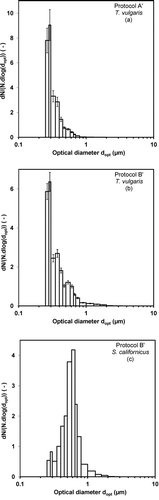
The size distribution of the bioaerosol generated from T. vulgaris cultures prepared with protocol B' () revealed the presence of a population of particles with a mode centred on a 0.55 μm optical diameter. This value is close to the expected aerodynamic diameter for T. vulgaris spores, 0.57 μm (Reponen et al. Citation1998). It is, however, difficult to precisely describe this population because it partially overlaps with populations of fine particles with modes close to 0.37 μm () and 0.1 μm (measurements with TSITM LAS Model-3340-data not shown). The population centred on 0.55 μm presented a pronounced right asymmetry, including particles with diameters up to several microns. These descriptive parameters vary little with test day or generation flow rate used.
The size distribution of the bioaerosol coming from protocol A' () was exclusively submicronic and did not show any discernible population representative of T. vulgaris spores or mycelium.
The general shape of the size distribution for protocols A and D (data not shown) is comparable to that for protocol A'. Similarly, the size distribution for protocols B and C (data not shown) are identical to that for protocol B'.
The protocol used to prepare the liquid T. vulgaris cultures affects the size distribution of the particles generated. In some cases, it reveals specific particle populations which are observable with an OPC.
3.2.2. Size Distribution of Culturable Particles Using the Sioutas® Cascade Impactor
Examples of size distributions for culturable T. vulgaris spores and mycelium as a function of the aerodynamic diameter are shown in .
FIG. 4 Size distributions of the bioaerosol (T. vulgaris) using preparation protocols A', B, B', and C. Sioutas® cascade impactor's measurements. Error bars represent the 95% confidence interval of nine repetitions.
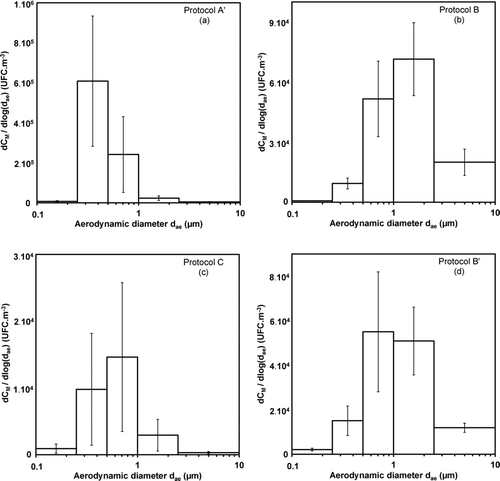
The size distribution measured differs depending on the method used to prepare the liquid culture.
The mode of the size distribution for the bioaerosol generated using protocol C is in the 0.5—1.0 μm range (). This impactor stage certainly contains a large number of spores (expected aerodynamic diameter 0.57 μm-Reponen et al. Citation1998). The concentrations measured in neighboring stages (0.25–0.5 and 1.0–2.5 μm) also indicate non-negligible levels of culturable particles with aerodynamic diameters below 0.5 μm and greater than 1 μm.
The size distribution of the bioaerosol generated using protocol A' has a mode in the 0.25–0.5 μm range, and very few culturable particles with diameters greater than 1 μm (). This protocol involves a sonication step during actinomycetes recovery which could cause this shift in size distribution toward fine diameters due to the formation of numerous smaller culturable entities.
For protocols B and B', the majority of culturable particles are collected on stages 0.5–1.0 and 1.0–2.5 μm ( and ). Discernible concentrations were only present on the 2.5– 10 μm stage of the impactor with protocols B and B' and not for the other protocols. This indicates the presence of culturable entities with much larger aerodynamic diameters than expected for T. vulgaris spores; these could be agglomerates of spores or nonfragmented mycelium strands.
Observations of epifluroescence microscope slides reveal the varied nature of the airborne culturable entities (Figure S2): spores, mycelium fragments, agglomerates of spores, and strands of mycelium. The different ranges of aerodynamic diameters measured on the impactor stages thus correspond to the heterogeneous culturable particles observed in the bioaerosol.
The size distribution and nature of particles making up the bioaerosol are significantly affected by the preparation steps involved in the protocol used to prepare the liquid culture.
3.3. Concentration Ranges and Reproducibility of the T. vulgaris Bioaerosols Generated
Total spores and culturable actinomycetes concentrations were measured as a function of the indicator IG . For each generation, the IG value can be adjusted by varying the bubbling airflow and/or by diluting the initial T. vulgaris liquid culture. Results for T. vulgaris are given in .
FIG. 5 Evolution of culturable actinomycetes and total spore concentrations in the bioaerosol as a function of IG for (a) T. vulgaris and for (b) T. fusca and S. Californicus. Error bars represent the 95% confidence interval of three repetitions. Liquid cultures were prepared with protocol B'. 0.3 < QG < 5 L.min−1; QE = 20 L.min−1; RH = 50 ± 2%; H liq = 8 mm.
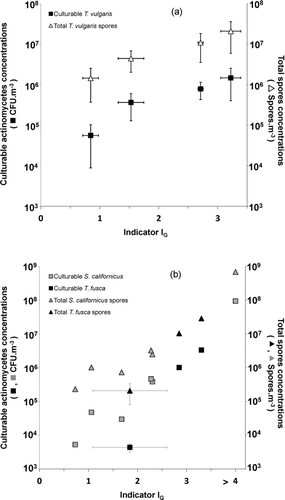
These results suggest that this generator could reproduce T. vulgaris aerosols in the following ranges (minimum and maximum values for all runs combined): total spore concentration between 4.3 × 105 and 3.6 × 107 Spores.m−3; culturable concentration between 8.6 × 103 and 2.6 × 106 CFU.m−3.
Variance in the indicator IG , as a process parameter, is also presented in .
Results indicate that the higher the indicator IG , the more concentrated the bioaerosol. With appropriate adjustment of the IG indicator, the operator can target a given concentration level.
3.4. Stability of the Bioaerosol Over 120 min Continuous Generation
The number concentration and size distribution of particles are stable over time, for at least 120 min continuous generation (data not shown). The average size distribution for 120 min generation is characterized by a profile very like that presented in . For each counting channel for d opt ≤ 1 μm, a coefficient of variation of less than 10% is measured. The stability of the indicator IG , which is exclusively dependent on the evolution of the concentrations CN (0.30 ≤ d opt < 0.45 μm) and CN (d opt ≥ 0.45 μm) (Section 2.3.2), was also evaluated. The IG indicator is also very stable over 120 min generation (coefficient of variation less than 4%), for all values tested (0.66 ≤ IG ≤ 3.31). More details on the stability are provided in Section S7 of SI.
3.5. Generation Tests with Streptomyces californicus and Thermobifida fusca
The results of generation tests with S. californicus and T. fusca are presented in . These concentration ranges are in good agreement with those obtained during tests with T. vulgaris.
The size distributions for bioaerosols of S. californicus and T. fusca (data not shown) are identical in pattern to those measured for T. vulgaris (). Nevertheless, the generation leading to the highest total spore concentration for S. californicus (7.0 × 108 spores.m−3—) gave rise to a specific size distribution, exceptionally rich in biological particles, which is shown in . This size distribution reveals unique physiological characteristics of bioaerosol release from this actinomycete culture (spores, mycelium, etc.). The distribution is characterized by a median optical diameter close to 0.58 μm, with a geometric standard deviation of 1.2 and a right asymmetry extending to almost 3 μm. This confirms the position of actinomycetes particles within the size distributions.
4. DISCUSSION
4.1. Choice of Conditions to Analyze the Culturable Actinomycetes Concentration
It is always difficult to choose a nutritive medium and incubation temperature for actinomycetes growth. No single culture medium is suitable for general use for all thermotolerant and thermophilic actinomycetes. T. vulgaris was grown on TSA medium at 50°C for 15 days prior to preparation of liquid cultures. These conditions were optimal for colony formation and spore production, as determined by preliminary comparisons (data not shown) with two other media (DifcoTM actinomycetes isolation agar mixed with 5 g.L−1 glycerol and Amner's R8 medium (Amner et al. Citation1989) and two other temperatures (45°C and 56°C). Incubation during 15 days was a compromise between the protocols described by Gazenko et al. (Citation1998, 7 days) and Reponen et al. (Citation1998, 3 weeks).
4.2. Activation of T. vulgaris Spores
T. vulgaris spores are formed endogenously, and are thus true endospores. Thermoactinomycetes endospores are constitutively dormant (Ensign Citation1978; Locci Citation2005); this means that fresh spores require activation to germinate when incubated in favorable conditions. According to Ensign's works (1978), our method of generation causes a heat shock and activates dormant T. vulgaris spores. Indeed, spores were incubated at 20°C for over three hour (time between the preparation of the liquid culture and the analysis of the samples collected during generation) and then, were plated out at 50°C. S. californicus and T. fusca form arthrospores which do not require activation before culture.
4.3. Comparison of Protocols to Prepare Liquid Cultures
4.3.1. Comparison of Culturable Actinomycetes and Total Spore Concentrations
Protocol D was not used because it provided significantly lower culturable concentrations than the other protocols, both in liquid and aerosol phases (). The ethanol concentration (30% ethanol solution in water) and the treatment time (1 min) used should not kill spores but are sufficient to kill the vegetative cells (Gazenko et al. Citation1998). We could not determine whether the low culturable concentrations result from an adverse effect of ethanol on the culturability of mycelium strands and fragments only, or if they are also related to a decrease in spore viability. Kawamoto et al. Citation1982 demonstrated that ethanol and other alcohols decreased Micromonospora and Streptomyces species survival in liquid cultures (after incubation at 30°C for 30 min).
Protocols A' and A provide the highest average culturable actinomycetes and total spore concentrations (). The sonication step probably plays a major role: a high power sonication may provide sufficient vibrations and energy to detach some spores from the mycelium and to break the hyphal structure into smaller fragments. Examination of sonicated liquid cultures confirmed that the mycelia of two Micromonospora species were broken into fragments after 2 min, and were completely disrupted after 20 min (Kawamoto et al. Citation1982). If disagglomeration also occurs, it may lead to increased number of isolated spores. Consequently, sonication may increase the number of spores accessible for counting during epifluorescence microscopic analysis. Some spores may be revealed after sonication, whereas they would have been masked as part of agglomerates or mycelium strands. Similarly, the number of culturable entities forming colonies is also increased by breaking down agglomerates of spores or fragmenting strands of mycelium.
Protocols B', B, and C provide intermediate concentrations. Through their narrow 95% confidence intervals, the bioaerosols coming from protocols B' and B provide the most reproducible culturable concentrations. In contrast, protocol C results in highly variable culturable concentrations in the bioaerosol, making it unsuitable for generation experiments.
4.3.2. Residual Nondesired Fine Particles in Bioaerosols
The size distribution of S. californicus biological particles shown in gives a clear view of the diameters for the population of interest. It constitutes an uncharacteristic example because, in all other tests, these particles are partially masked by residual non-desired fine particles, as described in Section 3.2.1. This constitutes a known artifact of aerosol wet generation (Simon et al. Citation2011). Undesired particles may be residues, impurities or dissolved substances which are still present in the liquid culture despite the washing steps included in the protocol (Section S8). In the case of protocol A' (), the population of biological particles is completely masked by the numerous residual particles also present.
4.3.3. Comparison of Size Distributions of the Bioaerosols
The impactor size distributions of culturable T. vulgaris particles () provide complementary data. For bioaerosols generated using protocols B' and C, the mode of the distribution is located on the 0.5–1.0 μm stage ( and ). This confirms the presence of numerous spores as the expected median aerodynamic diameter is 0.57 μm.
Culturable entities are also detected on other stages of the impactor, indicating the presence of culturable particles other than spores.
The culturable concentrations measured on the 0.25–0.5 μm stage could be explained by supposing that particles bounced off the stage above (Misra et al. Citation2002; Dunbar et al. [2005]) and were collected on lower stages (impaction stages were not greased). However, it is more likely that mycelium debris with small aerodynamic diameters was collected on this stage; some of them preserved their viability and were able to start new seats of growth. Biological entities smaller than spores exist, as revealed by numerous epifluorescence observations of microscope slides (Figure S2a). These actinomycetes fragments are almost certainly pieces of the hyphae of aerial mycelium or remnants of the fibrous sheaths surrounding developing spores (Williams et al. Citation1972; Lacey Citation1989; Gorny et al. Citation2003).
Similarly, the culturable concentrations measured on stages 1.0–2.5 and 2.5–10 μm can be explained by the presence of particles larger than isolated spores. These particles are agglomerates of spores, strands of mycelium, or ramified larger structures. The more selective frit used in protocol B' (pore diameters 10–16 μm) leads to a mode of the size distribution on stage 0.5–1.0 μm (). With protocol B (frit with pore diameter 16–40 μm) the particles overall have larger diameters, with a 1.0–2.5 μm mode ().
In contrast, bioaerosols produced using protocols A and A' have a mode on the 0.25–0.5 μm stage and the culturable actinomycetes concentrations measured on stages 1.0–2.5 and 2.5–10 μm are relatively low (). The presence of so many particles which can be assimilated to fragments/debris, and so few strands of mycelium or spore agglomerates is the result of sonication, as discussed in Section 4.3.1.
4.3.4. Choice of a Protocol to Prepare Liquid Actinomycetes Cultures for Generation
To prepare the liquid actinomycetes cultures for bioaerosol generation, we chose protocol B'. It provides high airborne concentrations (as high as ≈106 or ≈107 Spores.m−3) with satisfactorily reproducibility. It contains a complex mixture of spores, agglomerates of spores, and mycelium strands of various lengths; such a diversity constitutes richness for this experimental bioaerosol because it is closer to that of real aerosols found in workplaces. The population of biological particles is partially visible with an OPC, and allow iterative adjustments to the IG index. It is simple to implement; when six culture flasks are harvested, the culture can be prepared, ready for generation, by a single operator in approximately 60 min.
For future work, depending on the objectives, another protocol may be chosen to prepare cultures for generation, to produce a bioaerosol with different particles’ and concentrations’ characteristics.
4.4. The Generation Indicator IG
The indicator IG allows the operator to predict a given concentration level (culturable actinomycetes or total spores) by adjusting its value at the start of generation. This indicator is necessary because the airborne biological parameters are not accessible in real-time.
Measurements with the OPC Grimm® allow the operator to adapt the operating conditions as a function of the composition of the cultures to be generated. The use of a realtime indicator was previously tested when generating E. coli cells with the same aerosolizer (Simon et al. Citation2011). In this work, following the cumulated particle number concentration for particles with a diameter greater than 0.3 μm allowed a satisfactory estimation of the total airborne bacteria concentration, and thus was an appropriate indicator for the operator. Use of such a realtime indicator was only possible because the optical diameters of the E. coli cells and undesired smaller particles did not overlap.
The bioaerosols generated from our liquid actinomycetes cultures, however, had a typical size distribution for which the particles of interest partially overlap with finer undesired particles. A series of generation tests indicated that the level of residual particles can fluctuate from one culture to the next. Because they partially overlap, an increase in the residual particle population has a knock-on effect, producing a concomitant increase in the particles of interest. In these conditions, direct monitoring of the population of interest with an OPC, as suggested in Simon et al. (Citation2011), is not possible.
The IG indicator overcomes these difficulties. Adjusting the indicator takes approximately 15 min and is carried out before each assay. It requires generation of the initial liquid culture for a few minutes and realtime measurement of the ratio of the two particle number concentrations CN (d opt ≥ 0.45 μm) and CN (0.30 ≤ d opt < 0.45 μm) with an OPC.
This initial assessment, always performed on a culture harvested from six flasks in 50 mL of water (fD = 1), assesses the proportion of biological particles relative to the proportion of residual particles. If the IG value initially calculated is not in line with the operator's expectations (e.g., different from the value of a previous assay to be reproduced), it is possible to serially dilute the liquid culture (six flasks in V mL, where 50 < V ≤ 100 mL, i.e., 0.5 ≤ fD < 1) and to adjust the bubbling airflow. In addition to modifying the dilution factor, fD , diluting the culture and adapting the generation conditions will affect the CN (d opt ≥ 0.45 μm) and CN (0.30 ≤ d opt < 0.45 μm) concentrations, and most of all, their ratio. These modifications is explained by how the particles generated are formed, starting with the creation and bursting of a bubble of liquid, aerosolization of jet droplets and film droplets and, finally, drying of these droplets (Blanchard and Syzdek Citation1988; Günther et al. Citation2003). Dilution of the liquid culture modifies the “biological particle quantity/liquid quantity” ratio for each droplet produced. After drying, it influences the “biological particle quantity/residual particle quantity” ratio in the bioaerosol. The IG is recalculated until a value close to the desired value for the assay is reached. Once these brief preliminary experiments have been performed, the generation per se can start. The final value of the IG indicator is calculated based on the dilution factor, fD , and the mean particle number concentrations over the whole generation time. Use of the IG indicator is crucial for assays, first to attain, and then to reproduce target concentrations.
4.5. Concentration Ranges for the Bioaerosols Generated and Reproducibility
Whatever the species considered, the majority of culturable particle concentrations range between ≈103 and ≈106 CFU.m−3, while the total spore concentrations are in the range ≈105 to ≈107 Spores.m−3 (). These concentrations span a range commonly encountered in workplace environments. The culturable actinomycetes concentration levels published for a number of field studies are summarized in Table S3. They generally range between 104 and 106 CFU.m−3. This makes our experimental actinomycetes bioaerosols suitable for a variety of experiments (e.g., measure biocollector performance, develop nonculturable analysis methods, test protective equipment or investigate studies on inhalation toxicology). Moreover, the high culturable concentrations produced with the LSA show that the viability of many actinomycetes entities is well preserved during the generation. The highest concentrations can then be further increased by increasing the number of flasks initially harvested during preparation of the liquid culture or by increasing the bubbling airflow above 5 L.min−1.
4.6. Stability of the Bioaerosol Parameters Over Time
The results presented in Section 3.4 indicate good stability of the particle number concentrations and of the IG indicator over 120 min of continuous generation. The stability of the culturable and total spore concentrations has not been determined but is assumed based on the stability of IG . This bubbling generator was previously shown to produce stable culturable concentrations over time for bacteria (Simon et al. Citation2011).
The use of dry generators does not always allow the operator to work with such long-term stability over time. When a dry generation method is used, up to 90% of available actinomycetes propagules become airborne during the first 10 min of the release process (Gorny et al. Citation2003). The release rate with dry dispersion becomes much lower after 10 min because of the depletion of the reservoir of biological particles. A satisfactorily stability was obtained with Streptomyces albus for one hour of continuous dispersion with a swirling-flow disperser (Reponen et al. Citation1997).
5. CONCLUSION
We have shown that a wet bubbling generator can produce bioaerosols of actinomycetes spores and mycelium. Six protocols to prepare the liquid T. vulgaris cultures prior to generation were tested. The culture preparation method was shown to affect the culturable actinomycetes and total spore concentrations in the bioaerosol generated. Similarly, the nature, proportion, and physiologic of the different biological particles making up the bioaerosol (fragments, spores, mycelium, agglomerates of spores, and/or mycelium) depend on the preparation protocol used. Using the best protocol, the generator's performances were then validated using three species: T. vulgaris, T. fusca, and S. californicus. Experimental bioaerosols were shown to be stable for at least 120 min of continuous generation, and to present concentrations (culturable actinomycetes and total actinomycetes spores) representative of those measured in workplaces. Choice of the LSA was judicious because its gentle single-pass bubbling process was efficient to preserve the viability of many actinomycetes entities and to generate high culturable concentrations. A generation indicator, mainly based on realtime measurements of particle number concentrations, allows the operator to satisfactorily reproduce a target concentration between trials. The generator's flexibility and the method used (bubbling and ascending airflows, liquid film height, characteristics of the porous disc, preparation and composition of the liquid culture, etc.) are suitable for the production of bioaerosols with other actinomycetes species. These bioaerosols could be used in a large number of laboratory trials with various objectives and constraints.
In the future, these experimental bioaerosols can be used in tests to develop and validate molecular biology sampling and analysis methods for airborne actinomycetes. The properties that can be conferred to the bioaerosol using the generation method developed (stability over time, wide range of concentrations, satisfactory reproducibility) will also allow use in other tests related to the prevention of occupational diseases associated with exposure to actinomycetes.
Supplementary Files.zip
Download Zip (1.3 MB)Acknowledgments
[Supplementary materials are available for this article. Go to the publisher's online edition of Aerosol Science and Technology to view the free supplementary files.]
REFERENCES
- AFNOR . 2006 . Preservation of Test Organisms Used for the Determination of Bactericidal, Mycobactericidal, Sporicidal and Fungicidal Activity Standard NF-EN-12353, p. 23, AFNOR, Paris
- Amner , W. , Edwards , C. and McCarthy , A. J. 1989 . Improved Medium for Recovery and Enumeration of the Farmer's Lung Organism, Saccharomonospora viridis . Appl. Environ. Microbiol. , 55 : 2669 – 2674 .
- Blanchard , D. C. and Syzdek , L. D. 1988 . Film Drops Production as a Function of Bubble Size . J. Geophysic. Res. , 93 : 3649 – 3654 .
- Dunbar , C. , Kataya , A. and Tiangbe , T. 2005 . Reducing Bounce Effects in the Andersen Cascade Impactor . Int. J. Pharm. , 301 ( 1–2 ) : 25 – 32 .
- Ensign , J. C. 1978 . Formation, Properties, and Germination of Actinomycete Spores . Annu. Rev. Microbiol. , 32 : 185 – 219 .
- Fink , J. N. 1978 . “ Hypersensitivity Pneumonitis ” . In Allergy Principles and Practice , Edited by: Middleton , E. , Reed , C. E. and Ellis , E. F. 855 St-Louis : The C.V. Mosby Comp. .
- Gazenko , S. V. , Reponen , T. A. , Grinshpun , S. A. and Willeke , K. 1998 . Analysis of Airborne Actinomycete Spores with Fluorogenic Substrates . App. Environ. Microbiol. , 64 : 4410 – 4415 .
- Gorny , R. L. , Mainelis , G. , Grinshpun , S. A. , Willeke , K. , Dutkiewicz , J. and Reponen , T. 2003 . Release of Streptomyces Albus Propagules from Contaminated Surfaces . Environ. Res. , 91 : 45 – 53 .
- Griffiths , W. D. and DeCosemo , G. A. L. 1994 . The Assessment of Bioaerosols: A Critical Review . J. Aerosol Sci. , 25 : 1425 – 1458 .
- Griffiths , W. D. , Stewart , I. W. , Reading , A. R. and Futter , S. J. 1996 . Effect of Aerosolisation, Growth Phase and Residence Time in Spray and Collection Fluids on the Culturability of Cells and Spores . J. Aerosol Sci. , 27 ( 5 ) : 803 – 820 .
- Günther , A. , Wälchli , S. and Rudolph von Rohr , P. 2003 . Droplet Production from Disintegrating Bubbles at Water Surfaces. Single vs. Multiple Bubbles . Int. J. Multiphase Flow , 29 : 795 – 811 .
- Hoskisson , P. A. , Hobbs , G. and Sharples , G. P. 2000 . Response of Micromonospora Echinospora (NCIMB 12744) Spores to Heat Treatment with Evidence of a Heat Activation Phenomenon . Lett. Appl. Microbiol. , 30 : 114 – 117 .
- Jung , J. H. , Lee , C. H. , Lee , J. E. , Lee , J. H. , Kim , S. S. and Lee , B. U. 2009 . Design and Characterization of a Fungal Bioaerosol Generator Using Multi-Orifice Air Jets and a Rotating Substrate . J. Aerosol Sci. , 40 : 72 – 80 .
- Kawamoto , I. , Oka , T. and Nara , T. 1982 . Spore Resistance of Micromonospora Olivoasterospora, Micromonospora Sagamiensis and Related Organisms . Agric. Biol. Chem. , 46 : 221 – 231 .
- Lacey , J. 1989 . “ Thermoactinomycetes ” . In Bergey's Manual of Systematic Bacteriology , Edited by: Krieg , N. R. and Holt , J. G. Vol. 4, , 2573 – 2585 . The Williams & Wilkins Co . ., Baltimore, MD, USA, pp.
- Lacey , J. and Crook , B. 1988 . Fungal and Actinomycetes Spores as Pollutants of the Workplace and Occupational Allergens . Ann. Occup. Hyg. , 32 : 515 – 533 .
- Locci , R. 2005 . Actinomycete spores. Encyclopedia of Life Sciences , John Wiley & Sons, Inc., Chichester, England .
- Mainelis , G. , Berry , D. , Reoun An , H. , Yao , M. , DeVoe , K. Fennell , D. E. 2005 . Design and Performance of a Single-Pass Bubbling Bioaerosol Generator . Atmos. Environ. , 39 : 3521 – 3533 .
- Mainelis , G. , Willeke , K. , Baron , P. , Reponen , T. , Grinshpun , S. Gorny , R. 2001 . Electrical Charges on Airborne Microorganisms . J. Aerosol Sci. , 32 : 1087 – 1110 .
- McCullough , N. V. , Brosseau , L. M. , Vesley , D. and Vincent , J. H. 1998 . Improved Methods for Generation, Sampling, and Recovery of Biological Aerosoles in Filter Challenge Tests . Am. Ind. Hyg. Assoc. J. , 59 : 234 – 241 .
- Misra , C. , Singh , M. , Shen , S. , Sioutas , C. and Hall , P. M. 2002 . Development and Evaluation of a Personal Cascade Impactor Sampler (PCIS) . J. Aerosol Sci. , 33 : 1027 – 1047 .
- Reponen , T. , Willeke , K. , Ulevicius , V. , Grinshpun , S. A. and Donnelly , J. 1997 . Techniques for Dispersion of Microorganisms into Air . Aerosol Sci. Technol. , 27 : 405 – 421 .
- Reponen , T. A. , Gazenko , S. V. , Grinshpun , S. A. , Willeke , K. and Cole , E. C. 1998 . Characteristics of Airborne Actinomycete Spores . App. Environ. Microbiol. , 64 : 3807 – 3812 .
- Rule , A. M. , Schwab , K. J. , Kesavan , J. and Buckley , T. J. 2009 . Assessment of Bioaerosol Generation and Sampling Efficiency Based on Pantoea Agglomerans . Aerosol Sci. Technol. , 43 : 620 – 628 .
- Simon , X. , Duquenne , P. , Koehler , V. , Piernot , C. , Coulais , C. and Faure , M. 2011 . Aerosolisation of Escherichia Coli and Associated Endotoxin Using an Improved Bubbling Bioaerosol Generator . J. Aerosol Sci. , 42 : 517 – 531 .
- Stone , R. C. and Johnson , D. L. 2002 . A Note on the Effect of Nebulization Time and Pressure on the Culturability of Bacillus Subtilis and Pseudomonas Fluorescens . Aerosol Sci. Technol. , 36 : 536 – 539 .
- Terzieva , S. , Donnelly , J. , Ulevicius , V. , Grinshpun , A. S. , Willeke , K. Stelma , G. 1996 . Comparison of Methods for Detection and Enumeration of Airborne Microorganisms Collected by Liquid Impingement . App. Environ. Microbiol. , 62 : 2264 – 2272 .
- Ulevicius , V. , Willeke , K. , Grinshpun , A. S. , Donnelly , J. , Lin , J. and Mainelis , G. 1997 . Aerosolization of Particle from a Bubbling Liquid: Characteristics and Generator Development . Aerosol Sci. Technol. , 26 : 175 – 190 .
- Williams , S. T. , Bradshaw , R. M. , Costerton , J. W. and Forge , A. 1972 . Fine Structure of the Spore Sheath of Some Streptomyces Species . J. Gen. Microbiol. , 72 : 249 – 258 .