Abstract
Soot particles in the atmosphere can be coated with organic or nonorganic material, which may affect particle morphology and optical properties. The effect of the mass of coating on the morphology of soot particles was studied using oleic acid and dioctyl sebacate (DOS) coatings. A wide range of coatings were used, with up to 10 times as much coating as the mass of the soot. It is shown that as the coating mass increases the degree of collapsing increases until the coating is so large that the soot particle becomes completely contained within a spherical droplet of the coating material. Higher amounts of coating will not cause further collapse of the particle. The degree of collapse is also a function of the initial size of the soot particle but was independent of the coating materials tested, which have similar surface tensions. A model is presented to predict the change in mobility diameter as a function of coating mass ratio. The effect of coating mass on effective density, shape factor, and fractal dimension is also reported.
Copyright 2013 American Association for Aerosol Research
1. INTRODUCTION
It is generally accepted that aerosols are an important atmospheric constituent that can influence precipitation, visibility, and human health. They can affect Earth's temperature by the scattering and absorption of solar radiation. Soot generated from the burning of fossil fuel and biomass has a large positive component of radiative forcing compared to other aerosols (Jacobson Citation2001). Freshly emitted soot particles are typically hydrophobic and fractal aggregates and mostly externally mixed with nonrefractory compounds (Shiraiwa et al. Citation2007; Slowik et al. Citation2007). Once emitted into the atmosphere, BC particles undergo an aging process and become internally mixed. The internally mixed BC particles are often referred to as “coated” (McMeeking et al. Citation2011) and this coating of nonrefractory compounds (such as sulfate and organic materials) can change the optical properties of the BC (Cappa et al. Citation2012) as well as restructure the particle to a more compact shape. The restructuring of the soot particles will change the optical properties of the particles, which ultimately changes how these particles affect Earth's climate.
Slowik et al. (Citation2007) studied the effect of coating on the morphology of soot generated from a premixed ethylene/oxygen flame. They coated particles with oleic acid and anthracene and measured their properties such as mass, volume, effective density, composition, dynamic shape factor, mass-mobility exponent, and surface area with an aerosol mass spectrometer and a scanning mobility particle sizer (SMPS). Slowik et al. (Citation2007) qualitatively showed that oleic acid coating caused the BC particles to collapse into more a compact shape.
Zhang et al. (Citation2008) investigated the variability in morphology, hygroscopicity, and optical properties of soot aerosols during atmospheric processing. They reported soot particles exposed to sulphuric acid vapor exhibit a noticeable change in morphology, characterized by a decrease in the mobility-equivalent diameter and an increase in the mass-mobility exponent and effective density. They found that coating with sulfuric acid increased the scattering and the absorption of the particles. Xue et al. (Citation2009a) studied the effects of dicarboxylic acid coating on absorption and scattering of visible light by soot. They observed that internal mixing of soot aerosol with dicarboxylic acids substantially enhanced light scattering and to some degree increased light absorption. Xue et al. (Citation2009b) studied the effects of coating with dicarboxylic acids on the mass-mobility relationship of soot particles. They observed that coatings of succinic acid did not restructure the soot core while glutaric acid coatings did cause restructuring. Xue et al. (Citation2009b) suggest the difference in the restructuring could be due to the difference in melting point of the acids. Pagels et al. (Citation2009) also investigated soot particle morphology using controlled condensation of sulphuric acid and water vapor onto laboratory-generated flame soot. They reported for a specified soot source and condensing liquid, the degree of restructuring depends highly on the mass fraction of condensed material. Cross et al. (Citation2010) also studied changes in the morphology and optical properties of soot particles with coatings of sulphuric acid and dioctyl sebacate (DOS). They reported that the sulphuric acid coating caused the fractal soot core to restructure to a more compact configuration but the DOS coating did not affect the morphology of the original soot core.
Soot particles in the atmosphere can have different amounts of coating (Shiraiwa et al. Citation2007; Cappa et al. Citation2012), and the properties of soot can change depending on the amount of coating and the coating properties, although this relationship is not fully understood. Although some of the above studies suggest that the amount of coating is an important factor for the degree of soot restructuring, none have systematically investigated the effect of coating mass with a wide range of mass coating ratios or as a function of the initial particle size. In this study, the effect of coating mass on the morphology of soot particles is investigated using oleic acid and DOS coatings. A wide range of coatings were used, with up to 12 times as much coating as the mass of the soot.
2. EXPERIMENTAL SETUP
provides the schematic of the experiment. The experiment consists of a burner, two differential mobility analyzers (DMA; TSI model 3080), a centrifugal particle mass analyzer (CPMA), two condensation particle counters (CPC; TSI, Model 3776), a coating chamber, and a thermodenuder.
2.1. Burner
An inverted coflow diffusion flame based on the design of Stipe et al. (Citation2005) was used to produce soot particles. This burner can produce a wide range of soot particles sizes and concentrations. Due to the inverted design, the buoyant gases from combustion flow downward which prevent the flame from flickering. Hence, the concentration and size distribution of generated soot is steady with respect to time. In addition, Kirchstetter and Novakov (Citation2007) have shown using laser fragmentation-fluorescence spectroscopy that the soot particles generated by this burner have no measurable organic material on the particles. Our direct measurement of the mass of soot particles by CPMA before and after passing through a thermodenuder at 200°C confirms that there is not a measureable amount of volatile material (less than a few percent) on the generated soot.
Natural gas and air were supplied to the burner via mass flow controllers at flow rates of 1.3 SLPM (Cole-Parmer, model 32907–69) and 18 SLPM (Omega, model FMA-774-V), respectively. Primary dilution air, controlled by another mass flow controller (Omega, FMA-774-V), was injected into the exhaust at a flow rate of 37 SLPM. An ejector dilutor was used to dilute the sample by a further factor of 15. The mass, mobility, volatility, and morphologic properties of soot generated from the inverted burner is described in detail in Ghazi et al. (Citation2012). For the flame conditions used in this study, the count median mobility diameter and geometric standard deviation where approximately 160 nm and 1.85; respectively. The mean primary particle size of the soot particles was approximately 37 nm as determined by TEM analysis (Ghazi et al. Citation2012).
2.2. Coating Chamber
Soot particles were coated with either oleic acid or DOS. The aerosol flow was passed through a coating chamber similar to the design of Moteki and Kondo (Citation2007). The coating chamber is essentially a test tube (3 cm in diameter and 20 cm in length) partially filled with the coating material and maintained at constant temperature with an oil bath. The aerosol flow enters and exits the test tube through glass tubes passing through a stopper on top of the test tube. The bath temperature was held in the range of 95 to 100°C and 112 to 117°C for oleic acid and DOS; respectively. As a result the maximum achieved mass fraction (the ratio of the mass of the coated particle to the mass of the uncoated soot particle) of oleic acid and DOS coating for 350 nm particles were 10 and 8, respectively. DOS and oleic acid were used as a surrogate for atmospheric coatings because they readily condense to form large coatings and they have been used in previous restructuring experiments (Slowik et al. Citation2007; Cross et al. Citation2010). It is likely that the material properties of the coating (such as surface tension) will affect the collapse of the soot particles. In this study, the two different materials are liquid at room temperature and have similar surface tensions (32.79 and 31.73 mN/m for oleic acid and DOS, respectively; Chumpitaz et al. Citation1999; Wohlfarth and Wohlfarth Citation1997). It might be expected that different coating materials, like sulphuric acid or condensed organic compounds that solidify upon deposition, may result in different degrees of restructuring.
2.3. Differential Mobility Analyzer
The DMA classifies particles by their electrical mobility. Two DMAs were used in this study as shown in . The first DMA contained a Kr-85 neutralizer and the particles were not reneutralized in the second DMA. The first DMA was used to select an aerosol from the polydisperse soot distribution exiting the burner. The DMA will classify a narrow range of singly-charged particles near the mobility diameter of choice (100 to 450 nm in this study) as well as larger multiply-charged particles with the equivalent electrical mobility. The second DMA was used in a scanning mode with a CPC to measure the diameter of soot particles after the thermodenuder. Multiply-charged particles were often seen in the distribution of the second DMA and multimode fitting was used (when applicable) to determine the mobility equivalent diameter of only the singly-charged particles.
In general, the ratio of sheath to aerosol flow rate was maintained at approximately 10, except when large particles were classified which required that a lower sheath flow rate be used. The aerosol flow rate through the first DMA and CPMA was maintained at 0.72 LPM. The sheath flow of the first DMA was 6 LPM for selecting particles with diameter of 450 nm and 7 LPM for other diameters. The sheath flow of the second DMA was maintained at 3 LPM. Both CPC were operating at 0.3 LPM. To minimize the effect of coagulation on the results, all bypass flow paths had the same residence time as the coating chamber or thermodenuder.
2.4. Centrifugal Particle Mass Analyzer
The CPMA consists of two coaxial rotating cylinders and classifies particles by mass-to-charge ratio. The principle of operation is similar to the aerosol particle mass analyzer (APM; Ehara et al. Citation1996), except the inner cylinder in the CPMA rotates slightly faster than the outer one, which increases the penetration efficiency of the classifier (Olfert et al. Citation2006). The CPMA will classify a narrow range of singly-charged particles at the particle mass (m) of choice as well as heavier multiply-charged particles with the equivalent mass-to-charge ratio. The mass-to-charge ratio where the transfer function is a maximum is,
2.5. Thermodenuder
The thermodenuder consists of a long piece of copper tubing with two parts: a heating section and a cooling section. The heating section was maintained at 200°C and the cooling section was maintained at room temperature. Activated carbon was not used in the cooling section as it has been shown by Saleh et al. (Citation2011) that it should be avoided. To check the efficiency of the thermodenuder, the mass of size-selected particles before coating and after the coating/denuding process were compared. The results show no change in mass (within the uncertainty of the CPMA), which means that the thermodenuder could efficiently remove the thick coatings from the soot particles.
3. RESULTS AND DISCUSSION
shows the particle counts, measured with the CPC, of particles classified by the second DMA at various voltages. In this case, the second DMA is classifying 350 nm soot particles (and a small fraction of multiply-charged particles) exiting the first DMA in three cases: before coating, after being coated with oleic acid, and after being coated and then denuded.Footnote 1 In this figure, the CPMA was not being operated. The figure shows that coating with oleic acid increases the width of the distribution since the particles follow different trajectories in the coating chamber (which is consistent with Moteki and Kondo [Citation2007]). This wide distribution after coating shows not all particles grow the same amount, some obtain substantial amounts of coating while other particles receive relatively little coating. Due to this difference in the amount of coating, the degree of particle collapse is not the same for all of the particles, which causes the denuded distribution to become broader than the original particle distribution as shown in the figure. The novel aspect of this study is to study the effect of various amounts of coating. The figure also shows that the mode voltage (and by extension, the mode particle diameter) does not change considerably before and after coating at this specific coating chamber temperature, initial diameter, and number concentration. This behavior is due to the fact that the particles have been coated but the soot core has collapsed at the same time. However, with denuding, the mode voltage decreases (i.e., the mode particle diameter decreases) because of a collapse of the particle core. In this case, multiply-charged particles are not clearly seen in the figure, since there are few present at this setting (∼10%), and because the wide range of coatings produces a wide range of particle collapse as shown below. In all of the following results, the CPMA selected particles with a narrow range of coating thicknesses, and, in general, the multiply-charged particles appear in the voltage scan as a peak at a smaller voltage than the singly-charged particles.
FIG. 2 Particle counts of particles classified by the second DMA at various voltages. The second DMA is classifying 350 nm soot particles exiting the first DMA in three cases: before coating, after coating with oleic acid, and after coating/denuding.
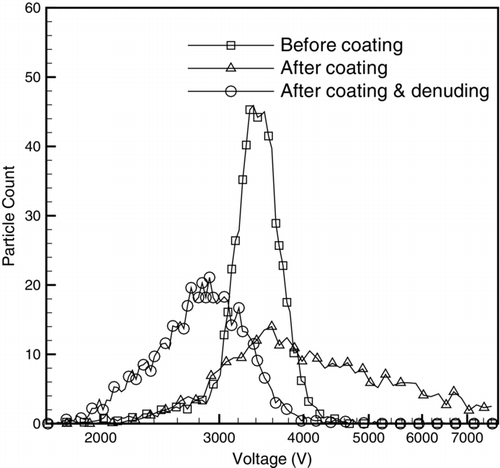
Figure 3 depicts the change in mobility diameter as a function of coating mass for undenuded soot. The mass coating ratio (m coated /m 0) and size coating ratio (d coated /d 0) are defined as:
There is no volatile material on the soot particles generated from the inverted burner, so the terms “mass of the initial soot particles,” “mass of the fresh soot particles,” and “mass of the soot particle core” all imply the same parameter.
The mass coating ratio ranges from 1 (no coating) to near 11 for the measurements shown in this work. As shown in , a wide range of mass coating ratio is possible at a given condition, however, the number concentration decreases as the mass coating ratio becomes large. The maximum achievable mass coating ratio is limited by having an adequate number of particles counted by the CPC. As the initial diameter increases, the maximum achievable m coated /m 0 for DOS coating decreases as shown in . Higher coating ratios with DOS were not achieved because there were not a sufficient number of particles for reliable measurements. However, mass coating ratios of approximately 10 for oleic acid were obtained for all three initial particle sizes.
It can be seen from that at low mass coating ratios the mobility diameter can actually decrease even though coating material has been added to the particle. This trend can be attributed to the collapse of the soot particle when it is coated. This decrease in mobility size tends to have the strongest effect on particles with a larger initial particle size and for coating mass ratios of approximately 2. At this mass coating ratio, there is enough coating material to cause particle collapse, but not enough coating material to significantly increase the mobility of the particles. Pagels et al. (Citation2009) produced a similar plot for sulphuric acid and sulphuric acid-water coatings for 152 nm soot particles. They found that small amounts of coatings (m coated/m 0 ≈ 2.5) caused a substantial collapse of the particle even with the coating material condensed on the soot (d coated/d 0 ≈ 0.7). In our study, the 150 nm DOS-coated particles do not show any decrease in particle size when coated because the particle collapse is offset by the particle growth due to the condensation of the DOS on the particle. The difference between these results is most likely due to the higher surface tension of sulphuric acid and water or perhaps due to a difference in primary particle size in the soot agglomerate (15 nm in Pagels et al. [Citation2009] and 37 nm here).
shows the change in mobility diameter after coating and denuding the soot particles for different mass coating ratios of DOS and oleic acid. The percentage change in mobility diameter has been defined as:
FIG. 4 Change in mobility diameter after the coating/denuding process as a function of coating mass ratio for (a) DOS and (b) oleic acid.
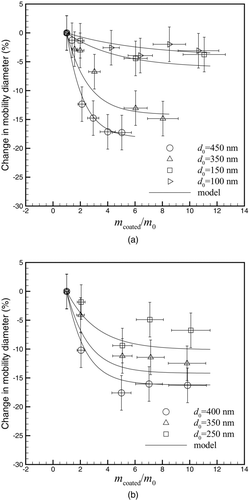
As seen in the , when the mass coating ratio becomes large, the degree of particle collapse becomes constant even if more coating is applied. It will be shown below that the particle reaches its final collapsed state after it becomes completely contained within a spherical droplet of the coating material (i.e., the dynamic shape factor of the coated particle is 1). Therefore, it is expected that higher amounts of coating will not further collapse the particle. In much of the literature (Kutz and Schmidt-Ott Citation1992; Pagels et al. Citation2009; Xue et al. Citation2009b) the restructuring of soot particles is attributed to the surface tension of the coating liquid. The surface tension of oleic acid (32.79 mN/m; Chumpitaz et al. Citation1999) and DOS (31.73 mN/m; Wohlfarth and Wohlfarth Citation1997) are quite similar. Thus, it is expected that the same degree of restructuring occurs with the two materials when the same amount of coating mass is applied.
Previous studies have investigated the restructuring of soot particles with oleic acid and DOS coatings but they have not shown the link between the amount of coating and the degree of restructuring. Slowik et al. (Citation2007) qualitatively showed that oleic acid could cause particle collapse. Cross et al. (Citation2010) coated particles with DOS and concluded that the DOS did not cause significant collapse because the change in mass-mobility exponent was not significant (an increase in mass-mobility exponent implies that the particle became more spherical due to collapse). Cross et al. (Citation2010) did notice a small increase in the mass-mobility exponent for DOS-coated/denuded soot, but the change was within the uncertainty of the measurement (the mass-mobility exponent of fresh soot was 2.12 ± 0.04 and after the coating/denuding process the exponent was 2.17 ± 0.06). However, it can be seen from that a change in mobility diameter of the soot particles due to DOS coating can be more than 15%. This difference in conclusion is likely due to the size range of soot particles tested and the amount of particle coating. The maximum diameter of the initial soot particle used by Cross et al. (Citation2010) was approximately 250 nm. As shown in this work, small particle sizes (100 and 150 nm) have a very small change in particle size after collapsing and this small change would be difficult to detect based on changes in the mass-mobility exponent.
A parameterization was developed to describe the change in mobility diameter as a function of initial core size and amount of coating mass. The model is shown with solid lines in . This model maybe useful for climate modelers to calculate the change in the optical properties of atmospherically processed soot based of the amount of coating material and initial particle size. The model was based on a simple exponential decay:
compares the model calculations with the experimental data. Good agreement is seen for both DOS and oleic acid coatings. Note that small particles (those containing just a few primary particles) cannot collapse by coating. Therefore, a more advanced model might include a piecewise function to account for particles with a small number of primary particles. However, more experimental points with small initial diameters are needed.
The effective density of a particle has an important role in particle transport properties. It is also required for calculating mass distributions from count distributions and finding the relationship between mobility diameter and aerodynamic diameter (DeCarlo et al. Citation2004). The effective density is defined as:
FIG. 6 The effective density of coated particles as a function mass coating ratio for (a) DOS and (b) oleic acid.
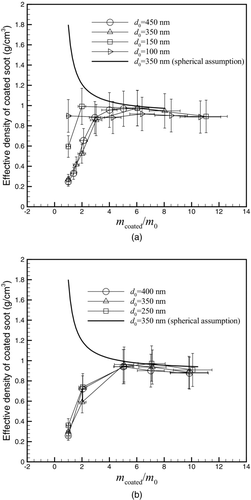
With increasing coating mass the effective density initially increases and then starts to decrease as more coating material is added. This can be explained by the fact that although the material density of soot particles is approximately 1.8 g/cm3 (Park et al. Citation2004), the effective density of the soot particles are between 0.3 to 0.8 g/cm3 depending on their initial size. Hence when the coating condenses on the soot, the density increases because the mass increases, but the mobility diameter does not increase significantly for a small amount of coating as shown in . In fact, the small amount of coating may cause the particles to collapse, which further increases the effective density. As the coating increases, particles get closer to a spherical shape and the effective density increases. With thicker coatings, the mass fraction of coating increases and due to the fact that the coating material density (0.9 g/cm3) is lower than the material density of soot (1.8 g/cm3), the effective density decreases. For comparison, the theoretical particle density of 350 nm particles for different coating mass fractions is plotted with the assumption of spherical particles. As coating mass increases and the particles reach a spherical shape and the experimental effective density approaches the theoretical density.
Another important particle property is the dynamic shape factor, which is shown in for the DOS and oleic acid coated soot. The dynamic shape factor is defined as the ratio of the actual drag force on a particle to the drag force on a spherical particle with the same volume (including any internal voids in the particle). For spherical particles the shape factor is 1. For nonspherical particles this value is usually greater than 1. The dynamic shape factor has been calculated using the expression (Hinds Citation1999),
FIG. 7 The dynamic shape factor as a function mass coating ratio for coatings of (a) DOS and (b) oleic acid. The open symbols represent coated and denuded soot particles. The closed symbols represent coated soot particles.
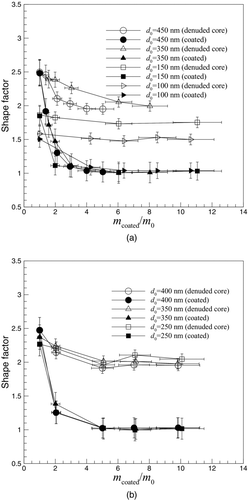
where d ve is the volume equivalent diameter, d me is the mobility equivalent diameter, and C ve and C me are the respective slip correction factors. The volume equivalent diameter of a coated particle is,

shows that initial soot particles have shape factors ranging from 1.5 to 2.5 as the diameter increases from 100 to 450 nm. Condensing coating material on the soot causes the shape factor to eventually decrease to one, which shows that the particles have reached a spherical shape. As expected, larger soot particles require higher mass coating ratios to create a spherical particle. The largest particles measured in this study become spherical at mass coating ratios of approximately 5 which approximately corresponds to the mass coating ratio at which no further coating causes particle collapse (). Another observation is that the coating and denuding process causes the shape factor to decrease as the particle restructures.
The particle mass-mobility relationship before coating with oleic acid, after coating, and after coating/denuding is shown in for particles that were coated with the highest mass coating ratio of oleic acid. It has been shown that the relation between mass and mobility diameter can be described by (Park et al. Citation2003),
FIG. 8 The particle mass-mobility relationship before coating, after coating with oleic acid (with the highest mass ratio), and after coating/denuding.
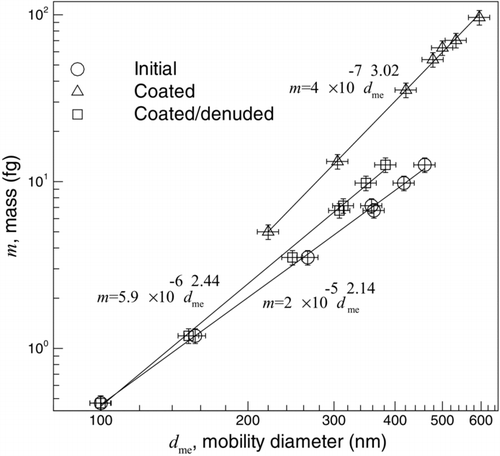
where C is a constant and D m is the mass-mobility exponent.Footnote 2 The solid lines in are a fit of the data using EquationEquation (9). The results indicate that upon coating with oleic acid, the mass-mobility exponent increases from 2.14 ± 0.03 to 3.02 ± 0.05 (for the highest mass fraction of coating) because with coating the particles become spherical in shape. After denuding, the mass-mobility exponent decreases to 2.44 ± 0.06, which is still higher than the mass-mobility exponent of fresh soot. This increase in the mass-mobility exponent is due to the restructuring of soot particles. The fact that after denuding the D m is less than 3, shows that particles are not spherical and further collapse might be possible. For example, Cross et al. (Citation2010) reported that by coating with sulfuric acid and denuding soot particles the mass-mobility exponent increased from 2.12 ± 0.04 to 2.49 ± 0.07. The higher mass-mobility exponent reported by Cross et al. could be due to the fact that the surface tension of sulfuric acid (52.55 mN/m; Wohlfarth and Wohlfarth Citation1997) is greater than oleic acid (32.79 mN/m; Chumpitaz et al. Citation1999), or perhaps the structure of the soot itself (i.e., primary particle size) may have allowed for a higher degree of collapse.
4. CONCLUSIONS
Soot particles in the atmosphere may have different amounts of coating, and the morphology and optical properties of soot can change depending on the coating mass ratio. In this study, the effect of coating mass ratio on the morphology of soot particles was investigated using oleic acid and DOS coatings. A wide range of coatings were used, with up to 12 times as much coating as the mass of the soot. Condensing coating material on the soot causes the shape factor to decrease to one for sufficiently large amounts of coating, indicating that the particles are spherical. Comparisons of mobility diameter, shape factor, and mass-mobility exponent before and after the coating/denuding process all indicate that coatings of DOS and oleic acid all cause the particles to restructure to a more compact shape (i.e., after the coating/denuding process the shape factor decreases compared to fresh particles and the mass-mobility exponent increases from 2.14 ± 0.03 to 2.44 ± 0.06). It was shown that as the coating mass increases the magnitude of collapsing increases until the coating is so large that the soot particle becomes completely contained within a spherical droplet of the coating material and higher amounts of coating will not cause further collapse of the particle. The degree of collapse is also a function of the initial size of the soot particle but was independent of the coating materials tested (DOS and oleic acid), which have similar surface tensions. A model, based on a simple exponential decay, was presented to predict the change in mobility diameter as a function of coating mass ratio. The agreement between the model and experimental results is very good.
In the atmosphere, coating materials can comprise of hydrophilic coatings such as secondary organic aerosols or sulfuric acid, where water uptake may play a significant role in soot restructuring (Pagels et al. Citation2009). Further work should be conducted with other coating materials and relative humidity to investigate these effects as a function of mass coating ratio. Also, other sources of soot, with different agglomerate properties (primary particle size and initial ‘compactness’) should be investigated to determine the full validity of the model.
Acknowledgments
The authors would like to acknowledge the support of NSERC Discovery Grant and Brookhaven National Laboratory (Grant number: SFR02855).
Notes
An inversion of the data was not performed to find the size distribution of the particles in these cases due to the inversion errors associated with narrow transfer functions entering the second DMA (Rader and McMurry Citation1986).
Previous work has erroneous called this exponent the “fractal dimension” or “mass fractal dimension.” The mass-mobility exponent is not equivalent to the fractal dimension and the term “mass fractal dimension” is a misnomer. Sorensen (2011) has shown the relationship between the true fractal dimension and the mass-mobility exponent.
REFERENCES
- Cappa , C. D. , Onasch , T. B. , Massoli , P. , Worsnop , D. R. , Bates , T. S. Cross , E. S. 2012 . Radiative Absorption Enhancements Due to the Mixing State of Atmospheric Black Carbon . Sci. , 337 ( 6098 ) : 1078 – 1081 .
- Chumpitaz , L. D. A. , Coutinho , L. F. and Meirelles , A. J. A. 1999 . Surface Tension of Fatty Acids and Triglycerides . J. Am. Oil Chem. Soc. , 76 : 379 – 382 .
- Cross , E. , Onasch , T. , Ahern , A. , Wrobel , W. , Slowik , J. G. Olfert , J. 2010 . Soot Particle Studies—Instrument Inter-comparison—Project Overview . Aerosol Sci. Technol. , 44 : 592 – 611 .
- DeCarlo , P. , Slowik , J. G. , Worsnop , D. , Davidovits , P. and Jimenez , J. 2004 . Particle Morphology and Density Characterization by Combined Mobility and Aerodynamic Diameter Measurements. Part 1: Theory . Aerosol Sci. Technol. , 38 : 1185 – 1205 .
- Ehara , K. , Hagwood , C. and Coakley , K. J. 1996 . Novel Method to Classify Aerosol Particles According to Their Mass-to-Charge Ratio - Aerosol Particle Mass Analyser . J. Aerosol Sci. , 27 : 217 – 234 .
- Ghazi , R. , Tjong , H. , Soewono , A. , Rogak , S. and Olfert , J. 2012 . Mass, Mobility, Volatility, and Morphology of Soot Particles Generated by a McKenna and Inverted Burner . Aerosol Sci. Technol. , in review
- Hinds , W. C. 1999 . Aerosol Technology—Properties, Behavior, and Measurement of Airborne Particles. , New York : John Wiley & Sons . 2nd ed
- Jacobson , M. Z. 2001 . Strong Radiative Heating Due to the Mixing State of Black Carbon in Atmospheric Aerosols . Nat. , 409 : 695 – 697 .
- Kirchstetter , T. W. and Novakov , T. 2007 . Controlled Generation of Black Carbon Particles from a Diffusion Flame and Applications in Evaluating Black Carbon Measurement Methods . Atmos. Environ. , 41 : 1874 – 1888 .
- Kutz , S. and Schmidt-Ott , A. 1992 . Characterization of Agglomerates by Condensation-Induced Restructuring . J. Aerosol Sci. , 23 : S357 – S360 .
- McMeeking , G. R. , Good , N. , Petters , M. D. , McFiggans , G. and Coe , H. 2011 . Influences on the Fraction of Hydrophobic and Hydrophilic Black Carbon in the Atmosphere . Atmos. Chem. Phys. , 11 : 5099 – 5112 .
- McMurry , P. H. , Wang , X. , Park , K. and Ehara , K. 2002 . Relationship Between Mass and Mobility for Atmospheric Particles . Aerosol Sci. Technol. , 36 : 227 – 238 .
- Moteki , N. and Kondo , Y. 2007 . Effects of Mixing State on Black Carbon Measurements by Laser-Induced Incandescence . Aerosol Sci. Technol. , 41 : 398 – 417 .
- Olfert , J. S. , Reavell , K. St J. , Rushton , M. G. and Collings , N. 2006 . The Experimental Transfer Function of the Couette Centrifugal Particle Mass Analyzer . J. Aerosol Sci. , 37 : 1840 – 1852 .
- Olfert , J. S. , Symonds , J. P. R. and Collings , N. 2007 . The Effective Density and Fractal Dimension of Particles Emitted from a Light-Duty Diesel Vehicle with a Diesel Oxidation Catalyst . J. Aerosol Sci. , 38 : 69 – 82 .
- Pagels , J. , Khalizov , A. , McMurry , P. and Zhang , R. 2009 . Processing of Soot by Controlled Sulphuric Acid and Water Condensation—Mass and Mobility Relationship . Aerosol Sci. Technol. , 43 : 629 – 640 .
- Park , K. , Cao , F. , Kittelson , D. B. and McMurry , P. H. 2003 . Relationship Between Particle Mass and Mobility for Diesel Exhaust Particles . Environ. Sci. Technol. , 37 : 577 – 583 .
- Park , K. , Kittelson , D. B. , Zachariah , M. R. and McMurry , P. H. 2004 . Measurement of Inherent Material Density of Nanoparticle Agglomerates . J. Nanopart. Res. , 6 : 267 – 272 .
- Rader , D. J. and McMurry , P. H. 1986 . Application of the Tandem Differential Mobility Analyzer to Studies of Droplet Growth or Evaporation . J. Aerosol Sci. , 17 : 771 – 787 .
- Saleh , R. , Shihadeh , A. and Khlystov , A. 2011 . On Transport Phenomena and Equilibration Time Scales in Thermodenuders . Atmos. Meas. Tech. , 4 : 571 – 581 .
- Shiraiwa , M. , Kondo , Y. , Moteki , N. , Takegawa , N. , Miyazaki , Y. and Blake , D. R. 2007 . Evolution of Mixing State of Black Carbon in Polluted Air from Tokyo . Geophys. Res. Lett. , 34 : 2 – 6 .
- Slowik , J. G. , Cross , E. S. , Han , J.-H. , Kolucki , J. , Davidovits , P. Williams , L. R. 2007 . Measurements of Morphology Changes of Fractal Soot Particles Using Coating and Denuding Experiments: Implications for Optical Absorption and Atmospheric Lifetime . Aerosol Sci. Technol. , 41 : 734 – 750 .
- Sorensen , C. M. 2011 . The Mobility of Fractal Aggregates: A Review . Aerosol Sci. Technol. , 45 : 765 – 779 .
- Stipe , C. , Higgins , B. , Lucasa , D. , Koshland , C. and Sawyer , R. 2005 . Inverted Co-Flow Diffusion Flame for Producing Soot . Rev. Sci. Instrum. , 76 : 023 – 908 .
- Wohlfarth , C. and Wohlfarth , B. 1997 . Surface Tension of Pure Liquids and Binary Liquid Mixtures, Group , New York : Springer .
- Xue , H. , Khalizov , A. F. , Wang , L. , Zheng , J. and Zhang , R. 2009a . Effects of Dicarboxylic Acid Coating on the Optical Properties of Soot . Phys. Chem. Chem. Phys. , 11 : 7869 – 7875 .
- Xue , H. , Khalizov , A. F. , Wang , L. , Zheng , J. and Zhang , R. 2009b . Effects of Coating of Dicarboxylic Acids on the Mass-Mobility Relationship of Soot Particles . Environ. Sci. Technol. , 43 : 2787 – 2792 .
- Zhang , R. , Khalizov , A. F. , Pagels , J. , Zhang , D. , Xue , H. and McMurry , P. H. 2008 . Variability in Morphology, Hygroscopicity, and Optical Properties of Soot Aerosols During Atmospheric Processing . Environ. Sci. , 105 : 10291 – 10296 .