Abstract
A two-stage particle concentration enrichment system was developed to provide highly concentrated particles at low flow rates, for applications in areas such as toxicity studies of particulate matter (PM) as well as for increasing the signal-to-noise ratio in online particle sampling instruments. The current system is an extension of the Versatile Aerosol Concentration Enrichment System (VACES) developed at University of Southern California and operates by placing a second-stage miniature virtual impactor (VI) downstream of the VACES. Particles are sequentially enriched through each stage. Laboratory evaluations were conducted using various types of polydisperse particles to simulate typical ambient PM components as well as monodisperse polystyrene latex (PSL) particles. The system's configuration was tested by adjusting the intermediate flow rate, which is the intake flow of the second-stage VI (or minor flow of the first-stage VIs), for which 15 L/min was determined to be optimal in terms of maximizing the overall concentration enrichment. Particle size distributions before and after concentration enrichment were compared using a scanning mobility particle sizer. Overall, our results indicate that the sampled particles were relative consistently enriched by factors of 100–120 (i.e., a concentration enrichment efficiency 75–85% of the ideal value) based on both PM mass and number concentrations, and along with similar physical properties of the size distribution (i.e., mode, median). Continuous and time-integrated field tests using urban ambient PM also showed consistent enrichment factors (by roughly 100–120 times) for number and mass concentrations, black carbon, and PM-bound polycyclic aromatic hydrocarbons.
Copyright 2013 American Association for Aerosol Research
1. INTRODUCTION
A number of health studies have demonstrated that exposure to high concentrations of PM has been linked to respiratory diseases (Li et al. Citation2003a), cardiovascular diseases (Delfino et al. Citation2005), neurodegenerative disorders (Campbell et al. Citation2005; Morgan et al. Citation2011), and adverse birth outcomes (Ritz et al. Citation2002; Wilhelm and Ritz Citation2005). Studies have also shown that the ultrafine fraction (defined as particles having a physical and/or aerodynamic diameter dp < 100–200 nm) may be more toxic than larger PM size fractions (Li et al. Citation2003b; Cho et al. Citation2005; Kleinman et al. Citation2008). In addition, the composition of PM is highly complex and variable as it depends on a number of factors including regional meteorology, topography, and natural (i.e., sea-salt) and anthropogenic (i.e., vehicular emissions) source strengths of PM. Urban ambient PM components include metals, trace elements, black carbon (BC), inorganic salts, and a large number of organic species (Cheung et al. Citation2011). Due to the complex composition of ambient PM, assessing which PM constituents are linked to adverse health outcomes as well as the exact mechanisms leading to these outcomes remains an active topic of research. To that end, recent advances in particle concentration techniques have become effective tools in facilitating health studies (Sioutas et al. Citation1997; Demokritou et al. Citation2002, 2003; Li et al. Citation2009; Rastogi et al. Citation2012) and in assisting ambient particle sampling (Geller et al. Citation2002; Ntziachristos et al. Citation2007).
The concentration enrichment of PM is achieved by means of virtual impactors (VIs), the operation of which is based on inertial separation of particles above and below a designated cutpoint. Particles larger than the VI cutpoint will cross the air streamlines and entrain the minor flow, while particles smaller than the cutpoint will follow the deflected streamlines and exit through the major flow. Hence, particles larger than the cutpoint are enriched by a theoretical factor equivalent to the intake-to-minor flow ratio. The design characteristics of VIs, such as separation efficiency versus particle aerodynamic diameter and internal wall losses have been extensively examined in both theoretical (Marple and Chien Citation1980) and experimental studies (Chen et al. Citation1986; Chen and Yeh Citation1987). Because of the adverse health effects associated with fine PM (dp < 2.5 μm), low cutpoint (0.1–0.25 μm), and round nozzle or slit nozzle VIs were developed in the mid-90s (Sioutas et al. Citation1994, Citation1997, Citation1999). However, ultrafine particles (dp < 0.1–0.2 μm) were not effectively concentrated by these technologies.
To expand the abilities of VIs to concentrate ultrafine PM, the Versatile Aerosol Concentrator Enrichment System (VACES) was developed, capable of concentrating both fine and ultrafine particles (Kim et al. Citation2001a,b). In the VACES, the particles are grown to super-micrometer size droplets via condensational growth, and subsequently concentrated by virtual impaction. Following concentration enrichment, particles pass through diffusion dryers where excess moisture is removed and they are returned to their original size. Previous studies have demonstrated that particle enrichment through the VACES does not have significant effects on the physical properties (i.e., mass and number concentration) and chemical composition of the particles (Kim et al. Citation2001b; Misra et al. Citation2004; Khlystov et al. Citation2005; Zhao et al. Citation2005). In addition to time-integrated filter sampling, applications of this technology have included uses in molecular and cellular in-vitro toxicity assays (Li et al. Citation2003b; Xia et al. Citation2004; Morgan et al. Citation2011), real-time in-vivo exposures (Li et al. Citation2004, Citation2009; Campbell et al. Citation2005; Kleinman et al. Citation2005; Lippmann et al. Citation2006), and direct PM collection in aqueous solutions for chemical and toxicological analysis (Daher et al. Citation2011). An advantage of using VACES for in-vitro exposure is the reduction in sampling time, which favors cell viability and exposure characterization (Sillanpaa et al. Citation2008). The VACES can also be coupled with a heater, in which semi-volatile particle-bound PM species are partitioned to gas phase, to be used for exposure studies separately to nonvolatile and semi-volatile components of PM (Pakbin et al. Citation2011). In addition to its applications for health studies and PM sampling efforts, high concentrations of PM can also enhance signal-to-noise ratios of online monitors including the Aerodyne Aerosol Mass Spectrometer (AMS) (Khlystov et al. Citation2005) and the rapid single-particle mass spectrometer (RSMS-3) (Zhao et al. Citation2005).
The VACES can concentrate particles at most by 20–30 times their ambient PM concentrations. The current study represents an extension of the VACES capabilities by placing two virtual impaction stages in series, yielding theoretical PM enrichment factors of up to 130. The resulting highly concentrated particles produced by the modified VACES broaden the scope of PM exposure studies, including recent and exciting advances in the areas of direct cellular exposures (Savi et al. Citation2008; Volckens et al. Citation2009), which are currently limited by the amount of PM material used in the exposure, and facilitate ambient PM sampling efforts, by improving the signal-to-noise ratios of many online monitors. This manuscript will discuss the laboratory and field validation tests of the two-stage VI system.
2. METHODS
The two-stage VI system is an extension of the previously developed VACES system (Kim et al. Citation2001a,b; Zhao et al. Citation2005). A second-stage miniature VI was placed in series after the first-stage VI to provide particles sequentially enriched through each stage. The system was first deployed and tested using stable laboratory particles, which were generated by a HOPE nebulizer (B&B Medical Technologies, Carlsbad, CA, USA). Particle mass and number concentrations as well as particle size distributions were measured before and after enrichment, using continuous monitors. Upon completion of the lab evaluation, the system was moved in the field and its concentration enrichment performance was validated using ambient PM.
The system schematic is presented in . The air stream was first drawn through the saturator tank filled with high performance liquid chromatography (HPLC) water, maintained at 35°C, to saturate the air with ultrapure water vapor. The particles leaving the saturator at about 27–28°C entered two condensational tubes that were connected to a circulating chiller to achieve super-saturation and promote condensational particle growth. The flow rate through each condensational tube was set at 100 L/min; particles were generally grown to 3–4 μm aqueous droplets, as measured by an Aerosol Particle Sizer (APS 3320, TSI Inc., Shoreview, MN, USA) and as reported in previous studies (Kim et al. Citation2001a,b). The particle droplets then passed through the first-stage VI in each line, which had a major flow rate of 100 L/min and a minor flow rate of 7.5 L/min (the determination of the optimum minor flow rate will be discussed in the next section). The two minor flows of the first-stage VIs were combined to 15 L/min to form the total intake flow of the second-stage VI, which operated with a minor flow of 1.5 L/min. Following the second-stage VI, concentrated particles were dried with a silica gel diffusion dryer (Model 3062, TSI Inc., Shoreview, MN, USA) and returned to their original size for characterization and evaluation.
The first part of the laboratory tests was to characterize the second-stage VI. Different sizes of monodisperse fluorescent polystyrene latex (PSL) particles (Polyscience Inc., Sacramento, CA, USA) were used to determine the 50% cutpoint of the VI. PSL particle sizes of 1, 1.5, 3, and 6 μm were generated by a nebulizer and the PM mass concentrations before and after the two-stage VI system were continuously measured with a DustTrak DRX Aerosol Monitor (Model 8534, TSI Inc., Shoreview, MN, USA). Different intake flows of 10, 15, and 30 L/min were tested with a minor flow fixed at 1.5 L/min.
The second part of the laboratory tests was to determine the optimal enrichment performance of the system. Different configuration tests were performed by adjusting the intermediate flow (see next section), defined as the combined minor flows of the first-stage VIs or the intake flow of the second-stage VI. In this test, polydisperse glutaric acid particles were generated and the number concentration was measured both upstream and downstream of the system with a Condensation Particle Counter (CPC 3022A, TSI Inc., Shoreview, MN, USA). Number concentration enrichment factors (i.e., the ratio of downstream to upstream values) measured by CPC were then compared to the theoretical enrichment factors to determine the enrichment efficiency. Intermediate flow rates of 10, 15, and 30 L/min were tested.
In addition, several other types of particles were generated by atomization and the particle size distribution before and after concentration enrichment by the system was measured. These included polydisperse particles of ammonium sulfate, ammonium nitrate, adipic acid, and glutaric acid. Ammonium sulfate and ammonium nitrate were chosen because they represent predominant inorganic species in PM2.5 in most areas of the U.S. (Malm et al. Citation2004). In addition, ammonium nitrate was chosen because it is a semi-volatile species (Mozurkewich Citation1993; Chang et al. Citation2000). Adipic acid and glutaric acid were selected because they are typical organic dicarboxylic acids formed by ozone photo-oxidation (Cruz and Pandis Citation1999; Saleh et al. Citation2012). A scanning mobility particle sizer (SMPS 3936, TSI Inc., Shoreview, MN, USA) coupled with a CPC was used to measure the particle size distribution upstream and downstream of the system. The SPMS/CPC was operated at sheath/particle flow rates of 3/0.3 L/min, covering a particle diameter range of 13–700 nm. The minor flow of the system was set to 1.5 L/min for ammonium sulfate, adipic acid, and glutaric acid and 1 L/min for ammonium nitrate.
In addition to polydisperse particles, a series of monodisperse PSL particles were generated to further evaluate the enrichment performance of the two-stage VI system. This series of tests was conducted to confirm that the concentration enrichment is not affected by a monodisperse particle size distribution as well as for ascertaining that the two-stage system is capable of concentrating coarse PM equally effectively. These tests also represent a theoretically greater challenge for this system, since PSL particles are hydrophobic and thus more difficult to be activated and grown by super saturation of water vapor. PSL particles of 100, 300, 400 nm and 1, 3, 6 μm were generated by atomization, as described earlier, and the mass concentrations before and after the two-stage VI system were measured by a DustTrak DRX. The tests for 3 and 6 μm were conducted without using the saturator and condenser parts of VACES since these particles are in the coarse PM range and already greater than the cutpoint of both Vis.
After completion of the laboratory evaluation, the system was deployed in the Particle Instrumentation Unit (PIU) of the University of Southern California from April to June 2012. The site is located in an urban area near downtown Los Angeles, California, within 150 m of a major freeway (I-110), and thus represents a typical urban mix of particle sources (Ning et al. Citation2007). The local ambient conditions during the sampling periods were 22–27°C and 20–40% relative humidity (RH), with PM2.5 concentration of 10–50 μg/m3, based on the data obtained from a local monitoring site maintained by the South Coast Air Quality Management District (SCAQMD). Particle size distribution measurements upstream and downstream of the system were conducted using SMPS/CPC system similar to the lab tests. Additionally, BC concentrations before and after the two-stage VI system were determined by means of a two-channel Aethalometer (Model AE-21, Thermo Andersen, Smyrna, GA, USA). In urban areas, BC is often dominated by vehicular emissions, which have been associated with adverse health outcomes (Invernizzi et al. Citation2011).
Finally, time-integrated ambient and concentrated PM2.5 samples were collected for PM-bound polycyclic aromatic hydrocarbon (PAH) analysis since PAHs are regarded as one of the most harmful components of ambient PM to human health (Ris Citation2007). Ambient PM2.5 was collected by a Micro-Orifice Uniform Deposit Impactor (MOUDI model 110, MSP Corp., Shoreview, MN, USA). The MOUDI was operated at 30 L/min using 37-mm Teflon filters (Teflo, Pall Corp., Life Sciences, 1-μm pore, Ann Arbor, MI, USA). A 37-mm Teflon filter was connected to the minor flow of the second-stage VI to collect concentrated PM. Ambient and concentrated PM samples were collected concurrently for about 7–8 h a day, and for about 2 days for each sample. The PAH analysis was conducted using gas chromatography-mass spectrometry (GC-MS) (Sheesley et al. Citation2004).
3. RESULTS AND DISCUSSION
3.1. Laboratory Characterization of Second-Stage VI
The characteristics of the first-stage VI were described in detail in previous studies by Kim et al. (Citation2001b). This VI was designed to have a 50% cutpoint of 1.5 μm at an intake flow rate of 110 L/min. In order to characterize the second-stage VI, different intake flow rates were tested, ranging from 10–30 L/min, with a constant minor flow of 1.5 L/min. The 50% cutpoint (d50) can be calculated by Stokes equation (Hinds Citation2002):
FIG. 3 Particle size distributions for laboratory-generated polydisperse particles for (a) ammonium sulfate, (b) adipic acid, (c) glutaric acid, and (d) ammonium nitrate (with minor flow of 1 L/min) upstream and downstream the two-stage concentrator. Physical properties of the size distribution including median, mean, mode, and total number and mass concentrations are shown. The secondary y-axis is multiplied by a factor of 100 (120 for ammonium nitrate) for visibility purposes.
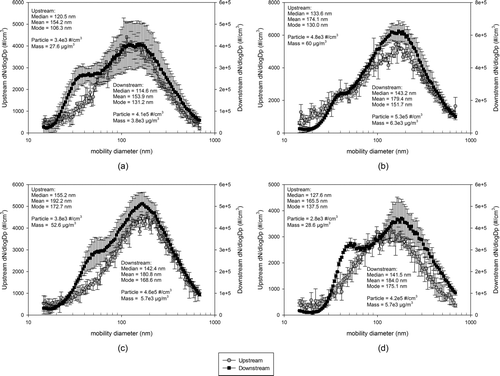
3.2. System Configuration Test
To test whether the overall concentration enrichment factor of the two-stage VI system is significantly influenced by the intermediate flow (i.e., the minor flow of the first-stage VIs and intake flow of the second-stage VI), different intermediate flow rates of 10, 15, and 30 L/min were tested. The theoretical enrichment factor, which is defined as the ratio of the intake flow of the first-stage VIs (200 L/min) to the minor flow of the second-stage VI (1.5 L/min), is 133. Results from the test shows that the two-stage system has relatively higher enrichment efficiency and less variability when operating at an intermediate flow rate of 15 L/min, with an enrichment efficiency of 80% (±6%), in comparison to 10 or 30 L/min, with an enrichment efficiency of 59% (±9%) and 61% (±10%). Using 15 L/min as the intermediate flow also yields a more even enrichment at each stage, with theoretical concentration enrichment factors of 13.3 in the first stage (200 to 15 L/min) and 10 in the second stage (15 to 1.5 L/min). Therefore, a higher overall enrichment efficiency may be achieved in addition to the lower variability in the overall concentration enrichment. As a result, 15 L/min was chosen as the optimal intermediate flow rate during all laboratory and field evaluation tests, with concentration enrichment factors of 100 to 120, corresponding to approximately 80 to 90% of the ideal theoretical value of 133.
TABLE 1 Upstream and downstream the two-stage concentrator mass concentrations, mass enrichment factors, and enrichment efficiency based on PSL tests
3.3. Laboratory Evaluation of the Two-Stage VI System
illustrates the concentration enrichment and size distribution of lab-generated particles. In each figure, the upstream concentration is presented on the primary y-axis on the left side and downstream concentration on the secondary y-axis on the right side. The secondary y-axis is 100 times (120 times in ) greater than the primary y-axis to make both datasets visible in one plot. show the size distribution results of ammonium sulfate, adipic acid, and glutaric acid, performed with a minor flow of 1.5 L/min. Although we observe some degree of distortion in the size distribution between the upstream and downstream, the overall mass and number enrichment factors are relatively stable and consistently over 100 times, corresponding to about 80% of the theoretical enrichment factor of 133. This is in close agreement with the expected value of 81% assuming that each VI stage performs at about 90% concentration efficiency. In addition to the consistent enrichment factors, physical properties of the particles, including the median, mode, and mean diameters for the upstream and downstream particles are also presented in the figures. For each species, the differences of median, mode, and mean diameter between upstream and downstream are within 10–15%, indicating that major physical properties of particle size distribution are not altered before and after particle concentration. The total number and mass concentration for both upstream and downstream are also included in these figures. The total number concentration enrichment factors for ammonium sulfate, adipic acid, and glutaric acid are 120 ± 31, 110 ± 10, and 121 ± 14; the total mass concentration enrichment factors are 130 ± 27, 105 ± 16, and 109 ± 10, respectively. shows the results of ammonium nitrate with a minor flow of 1 L/min. The total number and mass enrichment factors are 150 ± 16 and 195 ± 22, respectively, compared to the theoretical value of 200.
The slight discrepancy between mass- and number-based enrichment factors can be to a certain degree attributed to the lower concentration enrichment of sub-20 nm particles, as noted in our earlier publications (Geller et al. Citation2005; Ning et al. Citation2006) in which we discuss in greater detail the relationship between the minimum particle diameter that can be activated and grown by super-saturation in our VACES for externally-mixed ambient particles (e.g., urban background). The experimentally determined super-saturation ratio (S) in the VACES is in the range of 1.05–1.15, sufficient to activate externally-mixed insoluble particles of at least 20 nm and above (Ning et al. Citation2006). From the lab tests results, the average enrichment factors for particles in 13–20 and 20–30 nm are 53 ± 9 and 89 ± 21, respectively.
In , a local peak is observed at 50–60 nm for both the upstream and downstream particle size distribution among all four tested particles. presents the average enrichment efficiency (the average ratio of measured enrichment factor divided by theoretical value) for different size ranges among the lab and field evaluation tests. It is clear that in size range of 30 ∼ 60 nm, the average enrichment efficiency is over 100%, which corresponds to the local peak in . This peak may have been introduced by the sampling methodology, considering that the SMPS scans were not taken concurrently, and may also be the result of potential coagulation of smaller particles into larger particles for these size ranges. With the exception of sub-20 and 30 ∼ 60 nm particles, the results shown in and , prove the ability of the two-stage VI system to achieve an overall enrichment factor of 100–120 for submicrometer PM without drastically altering the particle size distribution and its physical properties (i.e., mode, median).
In addition to polydisperse particles, similar concentration enrichment tests were conducted with monodisperse PSL particles of 100, 300, 400 nm and 1, 3, 6 μm using the DustTrak DRX. shows the results of mass concentration enrichment for different PSL particle sizes. Generally, the concentration enrichment factors were in the range of 100–120 with uncertainties of 10–20%. These results corroborate the concentration enrichment performance of the two-stage VI system for ultrafine PM, PM2.5 and coarse PM.
3.4. Field Evaluation of Two-Stage VI System
Field validation measurements were carried out after completion of all laboratory tests. Particle size distribution tests were also conducted using the SMPS/CPC system and over 20 scans were taken for both ambient air (upstream) and concentrated air (downstream). These results are shown in . The median diameter for both upstream and downstream is approximately 30 nm for the duration of the field tests. Similar to the observations in the laboratory, the overlap of the two plots validates the consistent enrichment by a factor of around 100 for ambient PM. In addition, the mode, median, and mean diameters for both upstream and downstream differ by approximately 5%. However, we do observe some degree of distortion in size distribution for sub-20 nm and 30 ∼ 60 nm particles in the upstream and downstream, which may be attributed to the dynamic nature of ambient aerosols and the alternate (as opposed to concurrent) sampling between up-and downstream of our system.
FIG. 5 Particle size distributions of ambient PM upstream and downstream of the two-stage virtual impactor system.
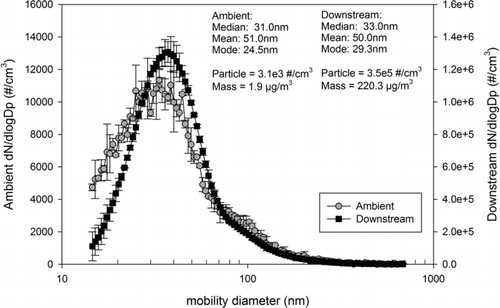
Similar results were observed for BC tests conducted by the two-channel Aethalometer. Ambient BC mass concentrations had an average of 500 ± 63 ng/m3 while downstream concentrations after passing through the two-stage VI system had an average of 54,000 ± 5100 ng/m3, corresponding to an enrichment factor of 100–110, consistent with laboratory results discussed earlier.
The final field evaluation test was based on time-integrated measurements of PAH concentrations upstream and downstream of the two-stage VI system. Results are presented in . Sixteen PAH species were analyzed by GC/MS: acenaphthene acenaphthylene, anthracene, benzo(a)anthracene, benzo(a)pyrene, benzo(b)fluornathene, benzo(ghi)perylene, benzo(k)fluoranthene, chrysene, dibenz(a,h)anthracene, fluoranthene, fluorene, indeno(1,2,3-cd)pyrene, phenanthrene, and pyrene. In , the average enrichment factor among different PAH species are presented. Aside from four species that are under detection limit (acenaphthene, acenaphthylene and indeno(1,2,3-cd)pyrene, and naphthalene are not shown), 12 species are presented in the plots. Overall, with the exception of dibenz(a,h)anthracene, the concentration enrichment factors for each species are very consistent, with an average value of 109 ± 26, which is in good agreement with those observed for other species in lab and field tests. Based on these results, the concentration enrichment performance of the two-stage VI system also seems to be unaffected by the molecular weight as well as the vapor pressure and volatility of the measured PAH
4. SUMMARY AND CONCLUSIONS
A two-stage VI system was developed and evaluated in this study. The particle size distribution measurements in the lab and in the field illustrate that an enrichment factor of 100–120 for both number and mass concentration is achieved along with similar physical properties of the size distribution (i.e., mode, median). Even though some degree of distortion in PM size distribution was observed, especially for sub-20 nm and 50 ∼ 60 nm particles, the results from this study have shown that the two-stage VI system is very effective at consistently enriching particles to very high number and mass concentrations. Field test results also demonstrated the ability of the system to concentrate BC and particle-bound PAH by enrichment factors close to their ideal value. Overall, the results in this study demonstrate that the two-stage VI system is appropriate for providing a robust and consistent concentration enrichment for different types of particles, a feature that makes it a potentially valuable tool in applications requiring highly concentrated particles especially at low flow rates (on the order of 1–2 L/min).
Acknowledgments
This study was funded by the National Institutes of Health through Grant #1566G HB474, and the Environmental Protection Agency through Grants/Awards #1566G HB474 and #RD-83374301-0 to the University of Southern California (USC). The research described herein has not been subjected to the agency's required peer and policy review and therefore does not necessarily reflect the views of the agency, and no official endorsement should be inferred. The authors would like to thank Dr. Junfeng Zhang (USC Keck School of Medicine) for his assistance in the chemical analysis.
Notes
aConcentration in μg/m3.
bConcentration in mg/m3.
REFERENCES
- Campbell , A. , Oldham , M. , Becaria , A. , Bondy , S. C. , Meacher , D. and Sioutas , C. 2005 . Particulate Matter in Polluted Air May Increase Biomarkers of Inflammation in Mouse Brain . Neurotoxicology , 26 ( 1 ) : 133 – 140 .
- Chang , M. C. , Sioutas , C. , Kim , S. , Gong , H. and Linn , W. S. 2000 . Reduction of Nitrate Losses from Filter and Impactor Samplers by Means of Concentration Enrichment . Atmos. Environ. , 34 ( 1 ) : 85 – 98 .
- Chen , B. T. , Yeh , H. C. and Cheng , Y. S. 1986 . Performance of a Modified Virtual Impactor . Aerosol Sci. Technol. , 5 ( 3 ) : 369 – 376 .
- Chen , B. T. and Yeh , H. C. 1987 . An Improved Virtual Impactor – Design and Performance . J. Aerosol Sci. , 18 ( 2 ) : 203 – 214 .
- Cheung , K. , Daher , N. , Kam , W. , Shafer , M. M. , Ning , Z. Schauer , J. J. 2011 . Spatial and Temporal Variation of Chemical Composition and Mass Closure of Ambient Coarse Particulate Matter (PM10–2.5) in the Los Angeles Area . Atmos. Environ.,Atmos. Environ. , 45 ( 16 ) : 2651 – 2662 .
- Cho , A. K. , Sioutas , C. , Miguel , A. H. , Kumagai , Y. , Schmitz , D. A. and Singh , M. 2005 . Redox Activity of Airborne Particulate Matter at Different Sites in the Los Angeles Basin . Environ. Res. , 99 ( 1 ) : 40 – 47 .
- Cruz , C. N. and Pandis , S. N. 1999 . Condensation of Organic Vapors on an Externally Mixed Aerosol Population . Aerosol Sci. Technol. , 31 ( 5 ) : 392 – 407 .
- Daher , N. , Ning , Z. , Cho , A. K. , Shafer , M. , Schauer , J. J. and Sioutas , C. 2011 . Comparison of the Chemical and Oxidative Characteristics of Particulate Matter (PM) Collected by Different Methods: Filters, Impactors, and BioSamplers . Aerosol Sci. Technol. , 45 ( 11 ) : 1294 – 1304 .
- Delfino , R. J. , Sioutas , C. and Malik , S. 2005 . Potential Role of Ultrafine Particles in Associations Between Airborne Particle Mass and Cardiovascular Health . Environ. Health Perspect. , 113 ( 8 ) : 934 – 946 .
- Demokritou , P. , Gupta , T. , Ferguson , S. and Koutrakis , P. 2003 . Development of a High-Volume Concentrated Ambient Particles System (CAPS) for Human and Animal Inhalation Toxicological Studies . Inhalation Toxicology , 15 ( 2 ) : 111 – 129 .
- Demokritou , P. , Gupta , T. and Koutrakis , P. 2002 . A High Volume Apparatus for the Condensational Growth of Ultrafine Particles for Inhalation Toxicological Studies . Aerosol Sci. Technol. , 36 ( 11 ) : 1061 – 1072 .
- Geller , M. D. , Biswas , S. , Fine , P. A. and Sioutas , C. 2005 . A New Compact Aerosol Concentrator for Use in Conjunction with Low flow-Rate Continuous Aerosol Instrumentation . J. Aerosol Sci. , 36 ( 8 ) : 1006 – 1022 .
- Geller , M. D. , Kim , S. , Misra , C. , Sioutas , C. , Olson , B. A. and Marple , V. A. 2002 . A Methodology for Measuring Size-Dependent Chemical Composition of Ultrafine Particles . Aerosol Sci. Technol. , 36 ( 6 ) : 748 – 762 .
- Hinds , W. C. 2002 . Aerosol Technology: Properties, Behavior, and Measurement of Airborne Particles , New York, NY : Wiley-Interscience .
- Invernizzi , G. , Ruprecht , A. , Mazza , R. , De Marco , C. , Mocnik , G. Sioutas , C. 2011 . Measurement of Black Carbon Concentration as an Indicator of Air Quality Benefits of Traffic Restriction Policies Within the Ecopass Zone in Milan, Italy . Atmos. Environ. , 45 ( 21 ) : 3522 – 3527 .
- Khlystov , A. , Zhang , Q. , Jimenez , J. L. , Stanier , C. , Pandis , S. N. and Canagaratna , M. R. 2005 . In Citu Concentration of Semi-Volatile Aerosol Using Water-Condensation Technology . J. Aerosol Sci. , 36 ( 7 ) : 866 – 880 .
- Kim , S. , Jaques , P. A. , Chang , M. C. , Barone , T. and Xiong , C. 2001b . Versatile Aerosol Concentration Enrichment System (VACES) for Simultaneous in vivo and in vitro Evaluation of Toxic Effects of Ultrafine, Fine and Coarse Ambient Particles - Part II: Field Evaluation . J. Aerosol Sci. , 32 ( 11 ) : 1299 – 1314 . Friedlander, S. K.
- Kim , S. , Jaques , P. A. , Chang , M. C. , Froines , J. R. and Sioutas , C. 2001a . Versatile Aerosol Concentration Enrichment System (VACES) for Simultaneous in vivo and in vitro Evaluation of Toxic Effects of Ultrafine, Fine and Coarse Ambient Particles - Part I: Development and Laboratory Characterization . J. Aerosol Sci. , 32 ( 11 ) : 1281 – 1297 .
- Kleinman , M. T. , Araujo , J. A. , Nel , A. , Sioutas , C. , Campbell , A. and Cong , P. Q. 2008 . Inhaled Ultrafine Particulate Matter Affects CNS Inflammatory Processes and May Act via MAP Kinase Signaling Pathways . Toxicology Lett. , 178 ( 2 ) : 127 – 130 .
- Kleinman , M. T. , Hamade , A. , Meacher , D. , Oldham , M. , Sioutas , C. and Chakrabarti , L. 2005 . Inhalation of Concentrated Ambient Particulate Matter Near a Heavily Trafficked Road Stimulates Antigen-Induced Airway Responses in Mice . J. Air Waste Manage. Assoc. , 55 ( 9 ) : 1277 – 1288 .
- Li , N. , Alam , J. , Venkatesan , M. I. , Eiguren-Fernandez , A. , Schmitz , D. and Di Stefano , E. 2004 . Nrf2 is a Key Transcription Factor That Regulates Antioxidant Defense in Macrophages and Epithelial Cells: Protecting Against the Proinflammatory and Oxidizing Effects of Diesel Exhaust Chemicals . J. Immunol. , 173 ( 5 ) : 3467 – 3481 .
- Li , N. , Hao , M. Q. , Phalen , R. F. , Hinds , W. C. and Nel , A. E. 2003a . Particulate Air Pollutants and Asthma - A Paradigm for the Role of Oxidative Stress in PM-Induced Adverse Health Effects . Clin. Immunol. , 109 ( 3 ) : 250 – 265 .
- Li , N. , Sioutas , C. , Cho , A. , Schmitz , D. , Misra , C. and Sempf , J. 2003b . Ultrafine Particulate Pollutants Induce Oxidative Stress and Mitochondrial Damage . Environ. Health Perspect. , 111 ( 4 ) : 455 – 460 .
- Li , N. , Wang , M. Y. , Bramble , L. A. , Schmitz , D. A. , Schauer , J. J. and Sioutas , C. 2009 . The Adjuvant Effect of Ambient Particulate Matter Is Closely Reflected by the Particulate Oxidant Potential . Environ. Health Perspect. , 117 ( 7 ) : 1116 – 1123 .
- Lippmann , M. , Ito , K. , Hwang , J. S. , Maciejczyk , P. and Chen , L. C. 2006 . Cardiovascular Effects of Nickel in Ambient Air . Environ. Health Perspect. , 114 ( 11 ) : 1662 – 1669 .
- Malm , W. C. , Schichtel , B. A. , Pitchford , M. L. , Ashbaugh , L. L. and Eldred , R. A. 2004 . Spatial and Monthly Trends in Speciated Fine Particle Concentration in the United States . J. Geophys. Res.-Atmos. , 109 ( D3 )
- Marple , V. A. and Chien , C. M. 1980 . Virtual Impactors - a Theoretical-Study . Environ. Sci. Techno. , 14 ( 8 ) : 976 – 985 .
- Misra , C. , Fine , P. M. , Singh , M. and Sioutas , C. 2004 . Development and Evaluation of a Compact Facility for Exposing Humans to Concentrated Ambient Ultrafine Particles . Aerosol Sci. Technol. , 38 ( 1 ) : 27 – 35 .
- Morgan , T. E. , Davis , D. A. , Iwata , N. , Tanner , J. A. , Snyder , D. and Ning , Z. 2011 . Glutamatergic Neurons in Rodent Models Respond to Nanoscale Particulate Urban Air Pollutants in Vivo and in Vitro . Environ. Health Perspect. , 119 ( 7 ) : 1003 – 1009 .
- Mozurkewich , M. 1993 . The Dissociation-Constant of Ammonium-Nitrate and Its Dependence on Temperature, Relative-Humidity and Particle-Size . Atmos. Environ., Part A-General Topics , 27 ( 2 ) : 261 – 270 .
- Ning , Z. , Geller , M. D. , Moore , K. F. , Sheesley , R. , Schauer , J. J. and Sioutas , C. 2007 . Daily Variation in Chemical Characteristics of Urban Ultrafine Aerosols and Inference of Their Sources . Environ. Sci. Techno. , 41 ( 17 ) : 6000 – 6006 .
- Ning , Z. , Moore , K. F. , Polidori , A. and Sioutas , C. 2006 . Field Validation of the New Miniature Versatile Aerosol Concentration Enrichment System (mVACES) . Aerosol Sci. Technol. , 40 ( 12 ) : 1098 – 1110 .
- Ntziachristos , L. , Ning , Z. , Geller , M. D. , Sheesley , R. J. , Schauer , J. J. and Sioutas , C. 2007 . Fine, Ultrafine and Nanoparticle Trace Element Compositions Near a Major Freeway with a High Heavy-Duty Diesel Fraction . Atmos. Environ. , 41 ( 27 ) : 5684 – 5696 .
- Pakbin , P. , Ning , Z. , Eiguren-Fernandez , A. and Sioutas , C. 2011 . Modification of the Versatile Aerosol Concentration Enrichment System (VACES) for Conducting Inhalation Exposures to Semi-Volatile Vapor Phase Pollutants . J. Aerosol Sci. , 42 ( 9 ) : 555 – 566 .
- Rastogi , N. , McWhinney , R. D. , Akhtar , U. S. , Urch , B. , Fila , M. and Abbatt , J. P. D. 2012 . Physical Characterization of the University of Toronto Coarse, Fine, and Ultrafine High-Volume Particle Concentrator Systems . Aerosol Sci. Technol. , 46 ( 9 ) : 1015 – 1024 .
- Ris , C. 2007 . US EPA Health Assessment for Diesel Engine Exhaust: A Review . Inhalation Toxicology , 19 : 229 – 239 .
- Ritz , B. , Yu , F. , Fruin , S. , Chapa , G. , Shaw , G. M. and Harris , J. A. 2002 . Ambient Air Pollution and Risk of Birth Defects in Southern California . Am. J. Epidemiol. , 155 ( 1 ) : 17 – 25 .
- Saleh , R. , Khlystov , A. and Shihadeh , A. 2012 . Determination of Evaporation Coefficients of Ambient and Laboratory-Generated Semivolatile Organic Aerosols from Phase Equilibration Kinetics in a Thermodenuder . Aerosol Sci. Technol. , 46 ( 1 ) : 22 – 30 .
- Savi , M. , Kalberer , M. , Lang , D. , Ryser , M. , Fierz , M. and Gaschen , A. 2008 . A Novel Exposure System for the Efficient and Controlled Deposition of Aerosol Particles onto Cell Cultures . Environ. Sci. Techno. , 42 ( 15 ) : 5667 – 5674 .
- Sheesley , R. J. , Schauer , J. J. , Bean , E. and Kenski , D. 2004 . Trends in Secondary Organic Aerosol at a Remote Site in Michigan's Upper Peninsula . Environ. Sci. Techno. , 38 ( 24 ) : 6491 – 6500 .
- Sillanpaa , M. , Geller , M. D. , Phuleria , H. C. and Sioutas , C. 2008 . High Collection Efficiency Electrostatic Precipitator for in Vitro Cell Exposure to Concentrated Ambient Particulate Matter (PM) . J. Aerosol Sci. , 39 ( 4 ) : 335 – 347 .
- Sioutas , C. , Kim , S. and Chang , M. 1999 . Development and evaluation of a prototype ultrafine particle concentrator . J. Aerosol Sci. , 30 ( 8 ) : 1001 – 1017 .
- Sioutas , C. , Koutrakis , P. and Burton , R. M. 1994 . Development of a Low Cutpoint Slit Virtual Impactor for Sampling Ambient Fine Particles . J. Aerosol Sci. , 25 ( 7 ) : 1321 – 1330 .
- Sioutas , C. , Koutrakis , P. , Godleski , J. J. , Ferguson , S. T. , Kim , C. S. and Burton , R. M. 1997 . Fine Particle Concentrators for Inhalation Exposures - Effect of Particle Size and Composition . J. Aerosol Sci. , 28 ( 6 ) : 1057 – 1071 .
- Volckens , J. , Dailey , L. , Walters , G. and Devlin , R. B. 2009 . Direct Particle-to-Cell Deposition of Coarse Ambient Particulate Matter Increases the Production of Inflammatory Mediators from Cultured Human Airway Epithelial Cells . Environ. Sci. Techn. , 43 ( 12 ) : 4595 – 4599 .
- Wilhelm , M. and Ritz , B. 2005 . Local Variations in CO and Particulate Air Pollution and Adverse Birth Outcomes in Los Angeles County, California, USA . Environ. Health Perspect. , 113 ( 9 ) : 1212 – 1221 .
- Xia , T. , Korge , P. , Weiss , J. N. , Li , N. , Venkatesan , M. I. Sioutas , C. 2004 . Quinones and Aromatic Chemical Compounds in Particulate Matter Induce Mitochondrial Dysfunction: Implications for Ultrafine Particle Toxicity . Environ. Health Perspect. , 112 ( 14 ) : 1347 – 1358 .
- Zhao , Y. , Bein , K. J. , Wexler , A. S. , Misra , C. , Fine , P. M. and Sioutas , C. 2005 . Field Evaluation of the Versatile Aerosol Concentration Enrichment System (VACES) Particle Concentrator Coupled to the Rapid Single-Particle Mass Spectrometer (RSMS-3) . J. Geophys. Res.-Atmos. , 110 ( D7 )