Abstract
In this study, we present our investigations on the counting efficiency of a TSI Environmental Particle Counter (EPC) Monitor Model 3783. The operation of the instrument is based on activation of sampled particles by water condensation, thus making it essentially a water-based condensation particle counter (WCPC). The counting efficiency was measured and simulated using different temperature settings on the conditioner and the growth tube, as well as using hygroscopic and water-soluble ammonium sulfate and sodium chloride particles, and insoluble and hydrophobic silver particles. The parameter that best describes the performance of the instrument is the cut-off diameter, or the diameter where 50% of sampled particles are activated and detected. In this study, the cut-off diameter for silver particles varied from 5.2 nm to 7.4 nm as a function of the temperature difference between the conditioner and the growth tube. The chemical composition and, specifically, the water solubility of particle components, had a significant effect on the counting efficiency. Using the standard operation settings of the EPC, the cut-off diameter for silver particles was 6.4 nm, and for ammonium sulfate and sodium chloride particles it was 4.8 nm and 4.3 nm, respectively. The simulations made with Comsol Multiphysics 3.5a simulation software gave the silver-water contact angle in the range of 29–45° depending on the temperature difference between the conditioner and the growth tube, and the effective contact angles of 25° and 21° for ammonium sulfate and sodium chloride particles.
Copyright 2013 American Association for Aerosol Research
1. INTRODUCTION
Condensation particle counters (CPC), or condensation nucleus counters (CNC), are used for measuring number concentrations of aerosol particles having diameters from a few nanometers up to some micrometers (McMurry Citation2000; Sipilä et al. Citation2009; Vanhanen et al. 2011; Yli-Ojanperä et al. Citation2012). The operation principle of a CPC relies on condensation of supersaturated working fluid vapor on the surface of aerosol particles until they are grown to optically detectable sizes. With this method, it is possible to detect small individual particles that have a small amount of mass without applying an electrical charge (McMurry Citation2000).
The new Environmental Particle Counter (EPC) Monitor Model 3783 is one of the latest laminar flow Water-based Condensation Particle Counter (WCPC) produced by TSI Inc. In the EPC TSI 3783, the sampled particles are grown to optically detectable diameters by water condensation (Hering and Stolzenburg Citation2005). The size enhancement happens in a wetted and temperature controlled wick tube with an inner diameter of 4.77 mm. The length of the cooled section of the tube (the conditioner) is 26.7 mm, and the length of the heated section (the growth tube) is 24.1 mm. The length of the insulator between the conditioner and the growth tube is 2.5 mm. The default temperature settings for the conditioner and the growth tube are 20°C and 60°C, respectively. A supersaturation region, where the particle size enhancement takes place, is formed in the growth tube, as the mass diffusivity of water is faster than thermal diffusivity of air. The general performance characteristics of this type of CPC have been presented by Hering et al. (Citation2005) and the physical operation principles by Hering and Stolzenburg (Citation2005). These studies showed that the solubility of aerosol material in the working fluid has an effect in the counting efficiency of the WCPC. Highly water-soluble particles, like sodium chloride and ammonium sulfate, have been observed to increase the counting efficiency of the water-CPCs significantly (Hering et al. Citation2005; Petäjä et al. Citation2006; Kulmala et al. Citation2007).
FIG. 1 Experimental set-up which was used to determine the counting efficiency of a TSI 3783 environmental particle counter (EPC).
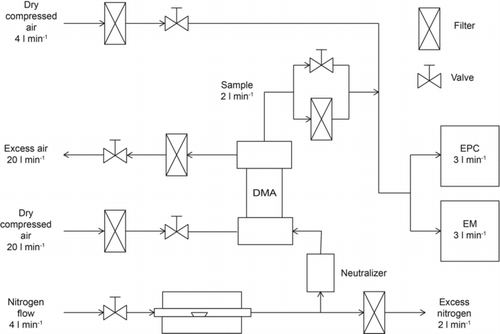
In this study, the particle counting efficiency of the TSI 3783 was experimentally investigated in the 2–12 nm diameter range and simulations were performed using Comsol Multiphysics 3.5a simulation software. Both hygroscopic and hydrophobic aerosol materials were used to study the response of the hygroscopicity of aerosol particles to the WCPC performance. Within the simulations, the heterogeneous nucleation theory and Köhler theory were used to simulate the activation of the particles inside the instrument. The investigations were done using silver, ammonium sulfate and sodium chloride particles. Silver particles below the diameter of 20 nm are spherical and insoluble in water (Sipilä et al. Citation2007). The salt particles are water soluble, but the shape and structure of the particles are not typically well known (Krämer et al. Citation2000, Hämeri et al. Citation2001, Larriba et al. Citation2011). We investigated the counting efficiency with different temperatures of the conditioner and the growth tube that allows adjustment of the cut-off diameter within certain limits. To prevent condensation, the temperature of the optics was set to the same as growth tube's. We stayed close enough to default temperature setting, so readjusting the alignment of the optics was not necessary. The counting efficiency and cut-off diameters were derived from experimental data by fitting a mathematical function. The counting efficiency curve can be used, for example, in the inversion algorithms of the size distribution measurements.
2. METHODS
2.1. Experimental Set-Up
In this study, the counting efficiency and cut-off diameter, D50 , for a TSI model 3783 were determined using a method described by Scheibel and Porstendörfer (Citation1983). A schematic figure of the calibration set-up is presented in . An aerosol sample was produced by evaporating silver (Ag), ammonium sulfate ((NH4)2SO4) or sodium chloride (NaCl) by passing a nitrogen flow (4 l min−1) through a tube furnace (Carbolite Furnaces MTF 12/388). Different temperatures were used depending on the material: 280°C for (NH4)2SO4, 750°C for NaCl, and 950°C for Ag. As the flow cooled down after exiting the furnace, the vaporized material nucleated forming polydisperse aerosol particles. The particles containing ammonium sulfate and ammonium bisulfate (NH4HSO4) produced by this method are referred to as ammonium sulfate particles in this study.
A sample flow of 2.0 l min−1 was extracted from the furnace generated aerosol and charged with an alpha-active 60 MBq241Am source (the neutralizer), and a monodisperse fraction was selected with a differential mobility analyzer (DMA VIE-08, Hauke, length 0.109 m). The sheath and excess flows of the DMA were set to 20.0 l min−1. The monodisperse sample flow was diluted with dry compressed air and directed to the EPC under investigation and to the reference instrument (TSI 3068 electrometer). The number concentration of the monodisperse charged sample was kept high enough to be reliably measured with the electrometer (EM). Aerosol particles smaller than 20 nm in diameter cannot, in practice, hold more than one elementary charge (Wiedensohler Citation1988), so multiple charging of particles can be ruled out. The EPC was set to high flow mode (inlet flow of 3.16 l min−1) and the inlet screen assembly to remove coarse particles was in place. The electrometer inlet flow was set to 3.0 l min−1. The sample flow was split to the EPC and EM using a simple tee connector. The EPC and EM had approximately the same flow rate and were connected to the experimental set-up with same kind and same length of tubing, so any transport losses before the EPC and EM can be neglected. The actual aerosol flow (0.12 l min−1), from which the aerosol number concentration is measured, is the same for low and high flow modes (TSI Citation2010). The possible difference in the counting efficiency between these two modes is due to diffusional losses before the conditioner and growth tube.
2.2. Data Analysis
The concentrations of monodisperse fractions of particles with different diameters were simultaneously measured with the EPC and EM. The counting efficiency (E(Dp)) curve of the EPC is determined as a ratio between the concentrations measured with the EPC and the EM. A least squares fit of an exponential function (1) was fitted to the experimental data, as in Petäjä et al. (Citation2006).
The supersaturation, which the particles experience inside the growth tube, can be altered by changing the temperatures of the conditioner and the growth tube. A higher temperature difference (ΔT) between the conditioner and the growth tube allows smaller particles to activate and grow large enough to be detected by the counting optics. The D50 values were plotted against the corresponding temperature differences, and a function (2) described by Petäjä et al. (Citation2006) was fitted to the measurement data
FIG. 2 The temperature and supersaturation conditions inside the conditioner and growth tube (1st and 2nd panels); the nucleation probability, or the region where the particles activate (3rd panel); and the normalized concentrations of unactivated and activated 5 nm silver (contact angle 34°) particles (4th and 5th panels). (Color figure available online.)
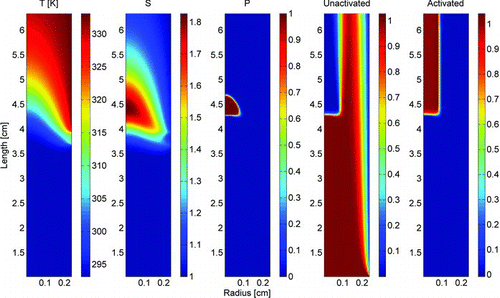
Parameters λ0 and λ were fitted to the data in least squares sense. Since the zero temperature difference does not result in supersaturated conditions within the growth tube, the largest possible cut-off diameter, λ0 , has no real meaning in practice.
2.3. Simulations
The counting efficiency of the EPC 3783 was also simulated with Comsol Multiphysics 3.5a simulation software. The supersaturation inside the growth tube was calculated by using the Incompressible Navier-Stokes model, within the Comsol Multiphysics module, to simulate the flow, and the Convection and Conduction and the Convection and Diffusion models in the Chemical Engineering module to simulate the heat and water vapor transport inside the conditioner and growth tube. The viscosity and water diffusion coefficient were assumed to be constant, and the flow entering the conditioner was assumed to be a plug flow of 0.120 l min−1. The losses from the inlet screen assembly were approximated by calculating the penetration through two lengths of 10 cm round tubing (Baron and Willeke Citation2001), the first with a flow rate of 3.0 l min−1 and another with a flow rate of 0.6 l min−1. The penetration through 200 μm screen is practically 100% in the particle size range used in this study (Cheng and Yeh Citation1980). The supersaturation field inside the growth tube was calculated assuming the inner walls have the pre-set temperatures and the RH at the walls is 100%. The results are shown in . With the supersaturation field, we calculated the activation of the particles of different diameter and contact angle using heterogeneous nucleation theory (Fletcher Citation1958) for nonsoluble particles and Köhler theory (Seinfeld and Pandis Citation2006) for soluble particles. The contact angle used with hetrogeneous nucleation theory is a measure of hydrophobicity. For totally wettable surfaces the contact angle is 0° degrees and for totally hydrophobic surfaces it is 180°. The activation rates were calculated from the heterogeneous nucleation and Köhler theories and were used with the Convection and Diffusion model in the Chemical Engineering module to calculate the activated fraction of the particles from the initial particle concentration. This fraction is the counting efficiency of the instrument, assuming that all the activated particles are detected. The activation rate in Köhler theory was assumed to be a step function; all of the particles that experience a supersaturation exceeding the critical supersaturation get activated. An example of the particle activation according the simulations is presented in .
FIG. 3 The effect of the temperature difference between the conditioner and the growth tube on the counting efficiency of the EPC TSI 3783 for silver particles. Continuous lines present fits through the experimental points. (Color figure available online.)
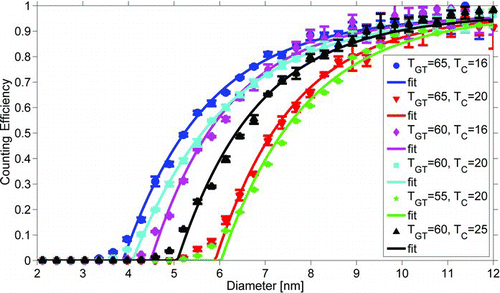
TABLE 1 The cut-off diameters (D50 ) and the smallest detectable particle diameters (D0 ) for silver particles with different conditioner and growth tube temperatures (ΔT); and the effective contact angles (θ), which were used to reproduce the corresponding cut-off diameters in the simulations. Estimated errors for the diameters are 0.3 nm, which correspond to the half-width of the transfer function through the DMA
3. RESULTS AND DISCUSSION
3.1. Counting Efficiency
The counting efficiencies of the EPC TSI 3783 with different conditioner and growth tube temperatures are presented in . The results from fitting an exponential curve (EquationEquation (1)) to each measured counting efficiency data set and the effective contact angles needed to reproduce the corresponding cut-off diameters according to simulations are presented in . The counting efficiency was only slightly altered, whether the same temperature difference was achieved by raising the growth tube temperature or lowering the temperature of the conditioner. Because of the Kelvin effect, the cut-off diameter has an exponential dependency on the saturation ratio. On the other hand, based on the Clausius-Clapeyron equation the saturation ratio is also an exponential function of the temperature difference between the conditioner and the growth tube. Therefore, an exponential curve (EquationEquation (2)) was fitted to the data. In , the cut-off diameters measured for different conditioner and growth tube temperature settings are plotted against the temperature difference. Also shown in is the temperature difference dependent behavior of the
simulated cut-off diameters for different effective contact angles. The temperature difference range is too narrow to clearly observe the exponential behavior in the measured points. For each temperature difference, a different contact angle is needed for the simulations to match the observations. Moreover, the counting efficiency curve is also steeper for the measured results, as can be seen in . This suggests that the contact angle alone is not sufficient to explain the activation of the particles inside the growth tube.
FIG. 4 The cut-off diameters plotted as a function of the temperature difference between the conditioner and growth tube; and the temperature difference dependent behavior of the simulated cut-off diameters with different effective contact angles. (Color figure available online.)
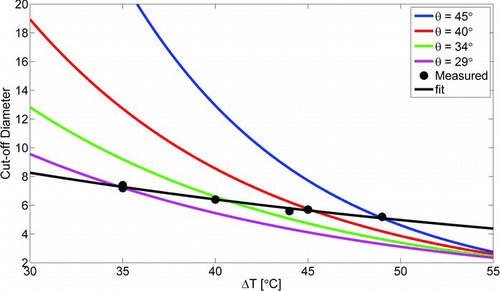
3.2. The Effect of Chemical Composition on the Counting Efficiency
The counting efficiency of EPC TSI 3783, as well as other water-based CPCs, depends on the chemical composition, and in particular, the water solubility, of sampled particles (Hering et al. Citation2005). The effect of chemical composition on the counting efficiency of EPC TSI 3783 was determined by measuring the counting efficiencies for water soluble ammonium sulfate and sodium chloride, and for insoluble silver particles. An exponential curve (EquationEquation (1)) was fitted to the measured data points. The results are presented in and . The comparison to other WCPC models are based on work by Petäjä et al. (Citation2006) for TSI 3785 and by Mordas et al. (Citation2008) for TSI 3786; and the contact angle for TSI 3785 is based on simulations performed by Stratmann et al. (Citation2010). The results from the simulations are presented in .
TABLE 2 Cut-off diameters (D50), smallest detectable particle diameters (D0) and the comparison between different WCPC models. The effective contact angles (θ) are obtained from the simulations with silver, ammonium sulfate, and sodium chloride particles with default EPC TSI 3783 conditioner and growth tube temperature settings (20°C and 60°C, respectively); estimated errors for the diameters are 0.3 nm
The counting efficiency for water soluble ammonium sulfate particles compared to insoluble silver particles was clearly higher. The cut-off diameter was 6.3 nm for silver and 4.8 nm for ammonium sulfate. The counting efficiency for sodium chloride was even better, with a cut-off diameter of 4.3 nm. The shape of ammonium sulfate particles is assumed to be almost spherical crystals and the sodium chloride particles produced by the method used in this experiment are agglomerates of unknown shape (Krämer et al. Citation2000). Therefore their diameters are not directly comparable to spherical silver particle diameters. Structurally better defined sodium chloride particles could have been produced by moistening them, but according to the results of Petäjä et al. (Citation2006), only a small difference in the counting efficiency would have been achieved.
The relative humidity inside the conditioner is close to 100%. In these conditions sodium chloride particles larger than 6 nm (Biskos et al. Citation2006) and ammonium sulfate particles larger than 8 nm (Hämeri et al. Citation2000) have already grown by condensation and gone through the phase transition from solid crystal to liquid droplet. Thus, the activation inside the growth tube occurs more easily than for hydrophobic silver particles. Also, the sodium chloride particles are more hygroscopic than the ammonium sulfate particles of the same size, which explains the difference in counting efficiency between these two (Hämeri et al. Citation2000, Citation2001).
The simulated counting efficiency when Köhler theory was used was significantly better than the measured for sodium chloride and ammonium sulfate. Our results show clearly that the traditional Köhler theory, assuming an ideal system, fails for the small sizes relevant to this study. Possible explanations include: (a) nonideal wetting of the CPC wick resulting in a slightly smaller supersaturation than simulated, (b) Köhler theory assumes activation is instantaneous, i.e., it does not allow for a time dependence of the activation process itself. This latter issue results in an error in our modeling simulations, where the activation is handled in a stepwise manner, by initiating without delay when a certain degree of supersaturation is reached. We tackled these problems using the heterogeneous nucleation theory instead, scaling the results using the effective contact angle as a free parameter. Although the physical meaning of this pseudo-parameter is somewhat contradictory, the results were in a fair agreement with the observations as can be seen from .
It seems that there are no previously published studies of the hygroscopic growth of the salts used in this study below the diameter of 6 nm (Hämeri et al. Citation2000, Citation2001; Biskos et al. Citation2006), so there is no verification whether the Köhler theory can explain the growth and the activation of the salt particles in this size range. The studies on the hygroscopic growth of ammonium sulfate and sodium chloride by Hämeri et al. (Citation2000, Citation2001) and Biskos et al. (Citation2006) shows that the hygroscopic growth of the particles below the diameter of 10 nm is less than the theory predicts. Presumably, the difference between the theory and the actual particle growth increases with even smaller particle diameters and thus some of the difference between our own experimental counting efficiency and simulations based on Köhler theory might be expected.
4. CONCLUSIONS
We evaluated the counting efficiency of EPC TSI 3783 water-based environmental particle counter. The measurements were done using hydrophobic silver particles, and hygroscopic ammonium sulfate and sodium chloride particles. The degree of supersaturation inside the growth tube was altered by changing the temperature difference between the conditioner and the growth tube, and its effect on counting efficiency was measured. The results of these investigations agree sufficiently with earlier studies. The uncertainties within the EPC and true size and shape of the particles can account for the small differences that were found. Especially in case of sodium chloride particles, the true shapes of the particles are unknown.
The effect of the degree of supersaturation, or the temperature difference between the conditioner and the growth tube, on the counting efficiency was as expected. The higher the temperature difference was, the higher the degree of supersaturation was achieved and smaller the particles could be detected. In these investigations, the cut-off diameter range was from 5.2 nm to 7.4 nm for silver particles. This range could be broadened even more. The conditioner temperature could not reach lower temperatures than 16°C, but the upper limit for the temperatures for the growth tube and the optics were not determined.
The chemical composition and, in particular, water solubility, had an effect on the counting efficiency of the EPC. With default operation conditions, the cut-off diameter for hydrophobic silver particles was 6.4 nm, whereas the cut-off diameters for hygroscopic ammonium sulfate and sodium chloride were 4.5 nm and 4.3 nm, respectively. The uncertainties of the cut-off diameters, stemming from an unknown shape, for ammonium sulfate and sodium chloride are larger than for silver. The difference between cut-off diameters of ammonium sulfate and sodium chloride can be explained by their hygroscopic qualities: sodium chloride is more hygroscopic than ammonium sulfate, and therefore, smaller sodium chloride particles are activated and detected.
The activation inside the growth tube of the instrument and the counting efficiency were also simulated using Comsol Multiphysics 3.5a simulation software. To simulate the activation of the insoluble silver particles, the heterogeneous nucleation theory was used. We found out that using contact angle as a single free parameter in our simulations could not fully explain the activation. The water-silver contact angles retrieved from the simulations were in the range (29–45°), which is in agreement with the value of 35.6° suggested by Wagner et al. (Citation2003). Similar results were obtained in a study by Stratmann et al. (Citation2010) for a WCPC TSI 3785 using FLUENT computational fluid dynamics code with the Fine Particle Model (FPM). Their simulations suggest a contact angle of 43.5° for default temperature settings, and they discovered a similar contact angle dependence on the temperature difference between the conditioner and the growth tube.
The Köhler theory was used to simulate the growth and the activation of soluble ammonium sulfate and sodium chloride particles. However, the simulations predicted activation of significantly smaller particles, and therefore higher counting efficiency than the experimental data. As a result, we decided to use heterogeneous nucleation theory with effective contact angle as a free parameter. The effective contact angles obtained from the simulations were 25° for ammonium sulfate and 21° for sodium chloride with the default conditioner and growth tube temperatures.
Atmospheric particles are always a mix of different chemical compositions. Therefore, the total concentrations measured with the EPC have a dependency on aerosol chemical characteristics, which vary both temporally and spatially (Biswas et al. Citation2005). This is a drawback for high time resolution ambient aerosol number concentration monitoring, since the composition of ultrafine particles is not sufficiently known.
We conclude that with the default temperature settings, the instrument can be confidently used down to 10 nm in practical applications for both hygroscopic and hydrophobic substances. In the ambient atmosphere, small nucleation mode particles are generally rather hygroscopic (Riipinen et al. Citation2009) excluding freshly emitted soot and organic particles (Saathoff et al. Citation2003a, Citation2003b). Thus, with caution, the instrument could be applied even down to 5–6 nm. Of course, in that case a careful assessment of error arising from the unknown chemical composition should be done corresponding to each deployment and application of the EPC. By increasing the temperature difference between the conditioner and the growth tube, the operation range could be extended to at least 1 nm smaller sizes.
Acknowledgments
We would like to acknowledge Gregory S. Lewis for his encouragement to elaborate this study, Jari Seppä and Mika Lumio from Labtronic for providing the instrument, and the Academy of Finland for funding (projects 139656 and 251417).
REFERENCES
- Baron , P. A. and Willeke , K. 2001 . Aerosol Measurement—Principles, Techniques and Applications (2nd ed.) , 1131 New York : John Wiley and Sons .
- Biskos , G. , Russell , L. M. , Buseck , P. R. and Martin , S. T. 2006 . Nanosize Effect on the Hygroscopic Growth Factor of Aerosol Particles . Geophys. Res. Lett. , 33 : L07801
- Biswas , S. , Fine , P. M. , Geller , M. D. , Hering , S. V. and Sioutas , C. 2005 . Performance Evaluation of a Recently Developed Water-Based Condensation Particle Counter . Aerosol Sci. Technol. , 39 : 419 – 427 .
- Cheng , Y. S. and Yeh , H. C. 1980 . Theory of Screen-Type Diffusion Battery . J. Aerosol Sci. , 11 : 313 – 320 .
- Fletcher , N. H. 1958 . The Size Effect in Heterogeneous Nucleation . J. Chem. Phys. , 29 : 572 – 577 .
- Hämeri , K. , Laaksonen , A. , Väkevä , M. and Suni , T. 2001 . Hygroscopic Growth of Ultrafine Sodium Chloride Particles . J. Geophys. Res. , 106 : 20749 – 20757 .
- Hämeri , K. , Väkevä , M. , Hansson , H.-C. and Laaksonen , K. 2000 . Hygroscopic Growth of Ultra-Fine Ammonium Sulfate Aerosol Measured Using an Ultrafine Tandem Differential Mobility Analyser . J. Geophys. Res. , 105 : 22231 – 22242 .
- Hering , S. V. and Stolzenburg , M. R. 2005 . A Method for Particle Size Amplification by Water Condensation in a Laminar, Thermally Diffusive Flow . Aerosol Sci Technol , 39 : 428 – 436 .
- Hering , S. V. , Stolzenburg , M. R. , Quant , F. R. , Oberreit , D. R. and Keady , P. B. 2005 . A Laminar-Flow, Water-Based Condensation Particle Counter (WCPC) . Aerosol Sci. Technol. , 39 : 659 – 672 .
- Kulmala , M. , Mordas , G. , Petäjä , T. , Grönholm , T. , Aalto , P. P. and Vehkamäki , H. 2007 . The Condensation Particle Counter Battery (CPCB): A New Tool to Investigate the Activation Properties of Nanoparticles . J. Aerosol Sci. , 38 : 289 – 304 .
- Krämer , L. , Pöschl , U. and Niessner , R. 2000 . Microstructural Rearrangement of Sodium Chloride Condensation Aerosol Particles on Interaction with Water Vapor . J. Aerosol Sci. , 31 : 673 – 685 .
- Larriba , C. , Hogan , C. J. Jr. , Attoui , M. , Borrajo , R. , Garcia , J. F. and de la Mora , J. F. 2011 . The Mobility- Volume Relationship Below 3.0 nm Examined by Tandem Mobility-Mass Measurements . Aerosol Sci. Technol. , 45 : 453 – 467 .
- McMurry , P. H. 2000 . The History of CPCs . Aerosol Sci. Technol. , 33 : 297 – 322 .
- Mordas , G. , Manninen , H. E. , Petäjä , T. , Aalto , P. P. , Hämeri , K. and Kulmala , M. 2008 . On Operation of the Ultra-Fine Water Based CPC TSI 3786 and Comparison with Other TSI models (TSI 3776, TSI 3772, TSI 3025, TSI 3010, TSI 3007) . Aerosol Sci. Technol. , 42 : 152 – 158 .
- Petäjä , T. , Mordas , G. , Manninen , H. , Aalto , P. P. , Hämeri , K. and Kulmala , M. 2006 . Detection Efficiency of a Water-Based TSI Condensation Particle Counter 3785 . Aerosol Sci. Technol. , 40 : 1090 – 109 .
- Riipinen , I. , Manninen , H. E. , Yli-Juuti , T. , Boy , M. , Sipilä , M. and Ehn , M. 2009 . Applying the Condensation Particle Counter Battery (CPCB) to Study the Water-Affinity of Freshly-Formed 2–9 nm Particles in Boreal Forest . Atmos. Chem. Phys. , 9 : 3317 – 3330 .
- Saathoff , H. , Moehler , O. , Schurath , U. , Kamm , S. , Dibbel , B. and Michelcic , C. 2003a . The AIDA Soot Aerosol Characterisation Campaign 1999 . J. Aerosol Sci. , 34 : 1277 – 1296 .
- Saathoff , H. , Naumann , K.-H. , Schnaiter , M. , Schöck , W. , Möhler , O. and Schurath , U. 2003b . Coating of Soot and (NH4)2SO4 Particles by Ozonolysis Products of α-pinene . J. Aerosol Sci. , 34 : 1297 – 1321 .
- Scheibel , H. G. and Porstendörfer , J. 1983 . Generation of Monodisperse Ag- and NaCl-aerosols with Particle Diameters Between 2 and 300 nm . J. Aerosol Sci. , 14 : 113 – 126 .
- Seinfeld , J. H. and Pandis , S. N. 2006 . Atmospheric Chemistry and Physics: From Air Pollution to Climate Change , New York : John Wiley and Sons .
- Sipilä , M. , Lehtipalo , K. , Attoui , M. , Neitola , K. , Petäjä , T. and Aalto , P. P. 2009 . Laboratory Verification of PHCPC's Ability to Monitor Atmospheric sub-3 nm Clusters . Aerosol Sci. Technol. , 43 : 126 – 135 .
- Sipilä , M. , Lushnikov , A. A. , Khriatchev , L. , Kulmala , M. , Tervahattu , H. and Räsänen , M. 2007 . Experimental Observation on Two-Photon Photoelectric Effect From Silver Aerosol Nanoparticles . New J. Phys. , 9 : 368
- Stratmann , F. , Herrmann , E. , Petäjä , T. and Kulmala , M. 2010 . Modelling Particle Activation and Growth in a TSI WCPC Model 3785 . Atmos. Meas. Tech. , 3 : 273 – 281 .
- TSI EPC 3783 Spec Sheet . 2010 . TSI inc .
- Vanhanen , J. , Mikkilä , J. , Lehtipalo , J. , Sipilä , M. , Manninen , H. E. Siivola , E. 2011 . Particle Size Magnifier for Nano-CN Detection . Aerosol Sci. Technol. , 45 : 533 – 542 .
- Wagner , P. E. , Kaller , D. , Vrtala , A. , Lauri , A. , Kulmala , M. and Laaksonen , A. 2003 . Nucleation Probability in Binary Heterogeneous Nucleation of Water-n-propanol Vapor Mixtures on Insoluble and Soluble Nanoparticles . Phys. Rev. E. , 67 : 021605.1 – 021605.12 .
- Wiedensohler , A. 1988 . An Approximation of the Bipolar Charge Distribution for Particles in the Submicron Range . J. Aerosol Sci. , 19 : 387 – 389 .
- Yli-Ojanperä , J. , Sakurai , H. , Iida , K. , Mäkelä , J. M. , Ehara , K. and Keskinen , J. 2012 . Comparison of Three Particle Number Concentration Calibration Standards Through Calibration of a Single CPC in a Wide Particle Size Range . Aerosol Sci. Technol. , 46 : 1163 – 1173 .