Abstract
The geometrical length of carbon nanotubes (CNTs) is of significant importance and this study aims at its fast determination. We evaluated a method to determine the geometrical length of CNTs by electrical mobility classification and developed a filtration based method. The measurement was carried out in air and the suitable generation method for airborne CNTs from liquid suspensions was investigated, followed by a proper control of the CNT dispersion degree by changing the CNT concentration in the liquid suspension. Airborne CNTs were generated by an atomizer, classified by a differential mobility analyzer and characterized by electron microscopy. The CNT geometrical lengths measured by electron microscopy served as the basis for comparison with other methods. Two theoretical models (Lall and Friedlander 2006; Li et al. 2012) for elongated particles were investigated and the comparison showed good agreement. This demonstrated the validity of the approach to determine the CNT length by the combination of mobility measurement and model calculation. The newly developed method, which employed a filtration model with uniform screens, showed comparable results with the CNT lengths as obtained from the electron microscopy.
Copyright 2013 American Association for Aerosol Research
1. INTRODUCTION
Carbon nanotubes (CNTs) have been used as a structural material for a decade in many applications such as sports equipment, semiconductor devices, aircraft coating, etc. The amount of CNT production is increasing every year, at the same time, toxicity of CNTs has raised increasing concerns (Lam et al. Citation2006; Kim et al. Citation2010; Wang et al. Citation2011a). The geometrical length is an important intrinsic property of CNTs and the extremely high length-to-diameter ratio is one of the hallmarks of CNTs. The geometrical length plays an important role on the mechanical, electrical, and optoeletronic properties (Wang et al. Citation2009) and has significant effect on CNT agglomeration status and toxicity (Wick et al. Citation2007; Wang and Pui Citation2013). For airborne CNTs, geometrical length affects the mobility and transport properties (Kim et al. Citation2007), and it is necessary to calculate lung deposition of fibrous particles using numerical model or to assess filtration efficiency of CNTs (Wang et al. Citation2011b,Citationc). For these reasons, determination of the geometrical length of CNTs is of significant interest.
Scanning electron microscopy (SEM) is commonly used to measure the geometrical properties of nanoparticles. Dispersion plays an important role in preparation of SEM samples, since CNTs are affected by the strong adhesion force. Atomizers (Lee et al. Citation2009; Seto et al. Citation2010) and electrosprays (Ku and Kulkarni Citation2009; Jennerjohn et al. Citation2010) were introduced to aerosolize CNTs from the liquid suspension. We investigated not only the generation method for airborne CNTs using an atomizer, but also the control method for the degree of CNT dispersion.
Recently Li et al. (Citation2012) investigated the theoretical model to calculate geometrical length of cylinder shaped particles. Lall and Friedlander (Citation2006) provided a model which could calculate the geometrical property of chain aggregates as a function of the mobility diameter. In the present study, the geometric lengths of CNTs were empirically measured by SEM analysis and compared with models in previous studies. We demonstrated that the combination of the mobility measurement and model calculation could give satisfactory results for the CNT length determination.
A new method to measure the geometrical length of CNT is introduced in the study. We employed filtration method to calculate the length of CNTs. Seto et al. (Citation2010) and Wang et al. (Citation2011b) validated experimental data of penetration of CNTs through uniform screens, which was characterized by using the single fiber filtration theory. Elongated aerosol particles including CNTs and chain agglomerates have lower penetration than spheres of the same electrical mobility or same volume, because they possess larger geometrical length and stronger interception effect (Lange et al. Citation1999; Kim et al. Citation2009a; Wang et al. Citation2011b; Wang Citation2013). Conversely, the geometrical length can be calculated from penetration of airborne CNTs with the single fiber filtration theory. This method shows possibility of fast measurement of geometrical parameter of CNTs with a simple setup for filtration using the screen. Myojo (Citation1999) developed a method to determine the length distribution of fibrous aerosol based on the penetration results through mesh screens. His model included several simplifications, including neglecting the flow disturbance by the screen wires and inertia of the fibers. He demonstrated the method was applicable for fibers with lengths in the range of 5–80 μm. Our method enables measurement of the geometrical length of elongated particles down to hundreds of nanometers.
2. EXPERIMENTAL SETUP
A Collison type atomizer was used to generate airborne CNTs. The multi walled carbon nanotubes (MWCNTs) from the generator were passed through a diffusion dryer to ensure that liquid vapors were removed. In order to classify the generated airborne CNTs, a differential mobility analyzer (DMA, TSI 3081) was used. The mobility size distribution was obtained by using the scanning mobility particle sizer (SMPS). In order to control the degree of CNT dispersion, the concentration in the suspensions was varied and the resulting size distributions of aerosolized CNTs were measured.
The dispersed airborne CNTs were collected on silicon substrates, by using a nanometer aerosol sampler (TSI 3089), for SEM analysis sample preparation purpose. A large number of DMA-classified airborne MWCNTs were collected and their lengths were measured from the SEM images. The length distribution of MWCNTs was fitted to a lognormal distribution and respective mean lengths were obtained. The obtained geometrical lengths were further on compared with models provided by Li et al. (Citation2012) and Lall and Friedlander (Citation2006). The lengths were also compared with calculated lengths by the filtration method.
shows a schematic drawing of the CNT filtration measurement system, which consists of an airborne CNT generation system, a size classification system, and a penetration measurement system. MWCNTs with diameters in the range of 15–20 nm (Baytubes, BMS, Germany), which were functionalized by the nitric acid refluxing method, and in the range of 20–30 nm (Cheaptubes, USA), which possessed a functional group COOH, were dispersed in deionized water and used in the experiments.
The MWCNTs from the generator were passed through a diffusion dryer, and classified by the DMA. Classified airborne CNTs were carried by the air flow with controlled flow rate. A neutralizer (Kr-85) was used to avoid electrostatic effect in the filter. Concentration of CNTs was measured by condensation particle counters (CPC, TSI 3775) upstream and downstream of the filter in order to obtain the fraction of penetration through the filter.
One of the tested filters was the screen made of 635-mesh type 304 stainless steel, which was used in the diffusion battery (TSI 3040). The screen wire was 20 μm in diameter with the opening dimension of 20 μm. The solidity α, which is the solid fraction in the filter compared to the total volume, are 0.345 (Wang et al. Citation2011b). The face velocity was 5 cm/s in all filtration tests. CNTs penetrating through the testing filter were removed by a high-efficiency filter before the flow went to the flow meter.
3. THEORETICAL MODELS
Li et al. (Citation2012) developed a model which describes relation between the geometrical length and electrical mobility diameter of cylinder shaped particles. They considered two cases, random orientation and total aligned orientation for the free molecular and transition regimes.
For the random orientation in the free molecular regime, the geometrical length of a CNT can be expressed as:
(1) where λ is the mean free path of gas, dm is the electrical mobility size, dCNT is the CNT diameter, Cc is the Cunningham slip correction, and
(2) where f is the momentum accommodation coefficient (=0.9).
For the total alignment
(3) Lall and Friedlander (Citation2006) theoretically determined the number of primary particles for the aggregates. The Lall and Friedlander model was developed for aggregates with open structures. Considering the geometrical similarity between a CNT and a chain aggregate composed of primary spheres with the same diameter, we applied the Lall and Friedlander model to compute the CNT length. The assumption was that the CNT length can be approximated as the length of a chain aggregate composed of primary spheres with the same diameter as the CNT diameter.
The number of primary particles in an aggregate can be calculated as:
(4) where c* is the dimensionless drag force, which takes value as 9.17 for the random orientation and 6.62 for the parallel orientation.
The geometrical length of CNT can be expressed as:
(5) We employed the single fiber theory for CNT penetration through a screen filter, which was used by Wang et al. (Citation2011c), to calculate the geometrical lengths of CNTs. Penetration of the airborne CNTs is calculated as:
(6) where α is the solidity of the filter (=0.345), t is the thickness of 20 layers of the screen filter, df is the wire diameter in the screen (=20 μm), and the total single fiber efficiency ET is summation of efficiency due to the diffusion, interception, impaction, and interception of particles undergoing diffusion
(7) The single fiber efficiency due to diffusion is expressed as:
(8) where
(9) In the equations, Pe is the Peclet number, U0 is the face velocity, D is the particle diffusion coefficient, k is Boltzmann constant, t is the temperature, and dp is the particle diameter. Wang et al. (Citation2011b) showed the diffusion coefficient can be directly related to the electrical mobility, thus dp can be approximated using the mobility diameter of CNTs.
The single fiber efficiency due to interception can be written as (Wang and Pui Citation2009):
(10) where
(11) Ku is the Kuwabara hydrodynamic parameter. The particle diameter dp can be directly used in the equation for spheres; however, other assumptions are needed for dp of CNTs. Wang et al. (Citation2011b,Citationc) computes the CNT interception length by using the geometrical length and the orientation angle θ between the CNT and the screen wire surface. When random orientation of the CNTs is assumed, the angle θ takes the average value of 40°. Then the interception parameter for CNTs is
(12) The efficiency due to inertial impaction can be written as:
(13) where
and ρCNT is the CNT density (=1.74 g/cm3, Kim et al. 2009b). The aerodynamic radius Rae of a fibrous particle is depended on the aspect ratio and can be written as:
(14) where
(15) In the equation Rae1 is for a parallel fiber orientation and Rae2 is for a perpendicular orientation within the gas flow. For the random orientation angle ψ takes the value 54.74°.
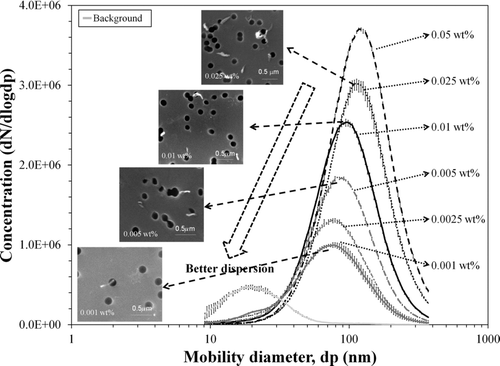
For interception of particles undergoing diffusion, single fiber efficiency is described as:
(16) The geometrical lengths of CNTs were obtained with spreadsheet software which employed the single fiber theory. We used experimental results of CNT penetration through the screen in the software and calculated the geometrical length of CNTs. As shown in the equations, the penetration is a function of the diameter, density, and length of CNTs and the diameter of screen wire. Penetration is determined experimentally and most of the parameters, such as the diameter and density of CNTs and diameter of screen wire, are known values. Only one unknown value is the geometrical length of CNT and that can be calculated from the equations presented above.
4. RESULTS AND DISCUSSION
4.1. Dispersion Degree of MWCNTs
Airborne MWCNTs were generated by an atomizer. This generation method was chosen over electrospray method, because of the sufficient amount of samples being produced. The atomizer generated relatively high concentration of airborne MWCNTs from the liquid suspension for SEM or Transmission Electron Microscope analysis. Additionally, as shown in , the size distribution of airborne MWCNTs was differentiated from the residual particle distribution under carefully controlled conditions.
Control of the dispersion degree of MWCNTs is significant for aerosol research. For instance, to define the geometrical characteristic of airborne MWCNTs, well dispersed samples with distinguishable individual MWCNTs are required. shows that modes of the size distributions of airborne MWCNTs were changed by varying the concentration in the suspension. Smaller mode sizes were obtained from the suspensions with lower concentrations. The concentration of airborne MWCNTs was also reduced when the concentration decreased in the suspension. By varying the concentration in the suspension, we were able to generate well dispersed MWCNTs or agglomerated MWCNTs with certain degree of control. Our results for Baytubes in the concentration range of 0.001 wt% to 0.05 wt% in the liquid suspension showed that lower concentrations led to better dispersed CNTs and higher concentration led to more agglomerates. The dispersion degree was determined from SEM images of the airborne MWCNTs collected on silicon substrates, as shown in the insets of . Short sampling time was used to ensure that the collected samples had significant distances among themselves and no apparent overlapping. The agglomerates showed tightly intertwined structure, which was highly unlikely to be resulted from sample overlapping on the substrate. Thus, we believe the agglomerates observed on the substrates reflected the agglomeration status of airborne MWCNTs.
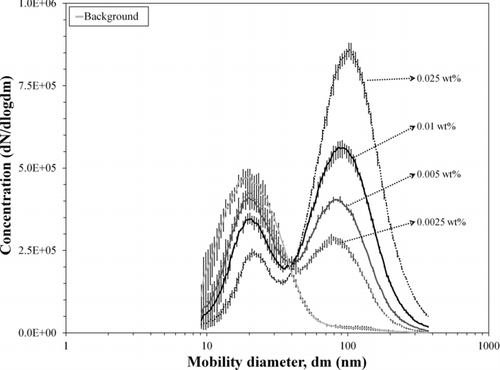
Results using MWCNTs from Cheaptubes are shown in . The smaller size peaks represent residual particles and larger size peaks the MWCNTs. With increase of the MWCNT concentration in the suspension, distribution mode of the MWCNTs increased slightly and the concentration for the mode size increased significantly. All the error bars in and indicate the maximum and minimum values of experimental data. The residual peak is comparable to the main aerosol peak in the case of Cheaptubes, whereas it is much lower than the main peak in the case of Baytubes, so that it is not prominent in . The difference between the number concentrations of airborne CNTs from the two types of CNTs even with similar mass concentration in suspensions might be because of the functionalization process. During the process, geometrical properties of CNTs were changed due to the processing conditions. For example, dependent on processing time of nitric acid oxidation, which is a common method to oxidize CNTs, CNTs can be damaged (Hu et al. Citation2003) and it can be a reason why CNTs from different providers possess different number concentrations in the specific size range. The airborne CNT size distribution and agglomeration level were dependent on the dispersion status in the suspension, which was in turn dependent on the CNT diameter, length, surface, and solvent properties. We have analyzed several kinds of MWCNTs from different manufacturers and found that the controlling method for the dispersion degree of CNTs, by varying the concentration in the suspension could not be used if the CNTs were not dispersed in the suspension properly.
4.2. Relation Between the Geometrical Length and Electrical Mobility Diameter
Airborne MWCNTs were collected on the silicon substrate in order to measure their geometrical length. shows a representative SEM image of collected MWCNTs from Cheaptubes with the 75 nm mobility diameter size. A number of images were processed for each sample, in order to obtain reliable length distributions. The minimum and maximum numbers of measured MWCNTs were 60 and 200, respectively, depending on the particle concentration of each sample. A significant fraction of straight, slightly bended or curved CNTs was obtained from the atomization method, as shown in . We used an image-processing software (Image J) to measure the geometric lengths of CNTs which represent the arc lengths for bended or curved CNTs. The small fraction of heavily curved or folded CNTs were neglected, because they cannot be compared to the electrical mobility or filtration model in the present study, which assume either cylinder shaped or straight particles. This is a limitation of the existing models; however, our study demonstrates that they give satisfactory results for significant fraction of CNTs in the size range under consideration.
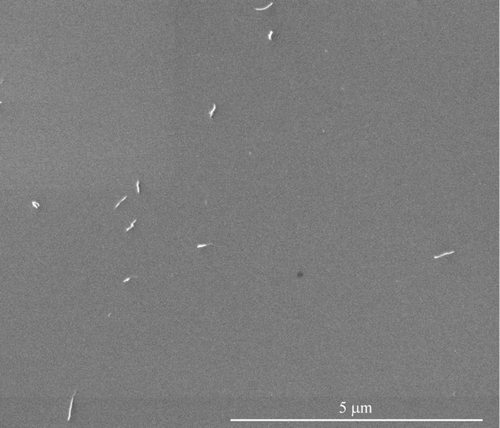
The geometrical length of airborne MWCNTs measured by SEM analysis showed a single mode distribution as presented in . In order to define the mean length of MWCNTs, the geometrical length distribution was fitted to a lognormal distribution. For instance, and show results of classified MWCNTs (Cheaptubes) with 75 nm mobility size and the 221 nm mean length. The relation between the electrical mobility diameter and geometrical length of airborne MWCNTs from Cheaptubes is shown in , where the x axis is the mobility diameter (dm) divided by the Cunningham slip correction factor (Cc). Same type of results for MWCNTs from Baytubes is shown in . According to the linear fitting of the data, Baytubes CNTs possess longer geometrical length than MWCNTs from Cheaptubes with the same mobility diameter. This was attributed to the fact that CNTs from Baytubes have smaller tube diameters than those from Cheaptubes, which is in agreement with the models of Li et al. (Citation2012) and Lall and Friedlander (Citation2006).
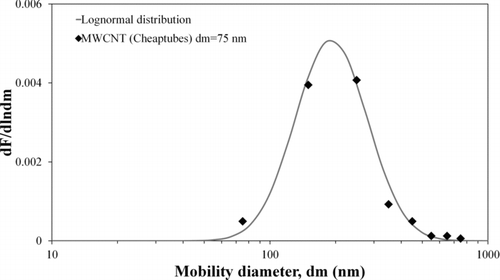
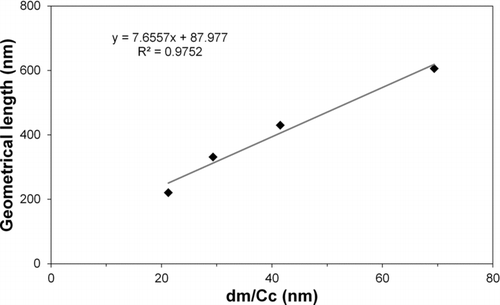
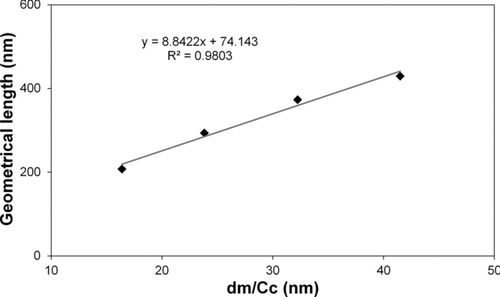
shows comparison of measured geometrical lengths of CNTs with several previous studies. Uncertainties of each study were calculated using the standard deviation of the experimental data and shown in . MWCNTs from Cheaptubes and Baytubes showed similar length distributions as the results presented by Kim and Zachariah (Citation2005). Wang et al. (Citation2011b) and Seto et al. (Citation2010) showed considerably longer lengths of CNTs than the present results and Kim and Zachariah’ results. This difference seems to be due to the sizes of diameters of used CNTs. CNTs from Cheaptubes and Baytubes and the ones used in Kim and Zachariah's study possessed relatively small diameter values at 25, 20 and 15 nm, respectively. However, CNTs in the studies by Wang et al. and Seto et al. possessed 85 and 65 nm diameters, respectively. The model of Lall and Friedlander was derived for free-molecular flows, which indicated that the Knudson number (=2λ/dCNT) should be significantly larger than 1. It appears that the flows for CNTs in Wang et al. (Citation2011b) and Seto et al. (Citation2010) were not in the free-molecular flow regime. It might be part of the reason why the particles, which possessed different geometrical lengths, were classified as same mobility size particles.
4.3. CNT Lengths Measurement by the Filtration Method and Comparison with the Theoretical Models
Penetration of MWCNTs was measured and applied to the model, which included the particle capture mechanisms, to calculate the geometrical length of CNTs. shows penetration of both Cheaptubes and Baytubes MWCNTs through the screen with flow velocity of 5 cm/s. CNTs from Baytubes showed lower penetrations than those from Chepatubes, because they possessed longer geometrical lengths. We input the penetration as a parameter to the model. Uncertainties due to the fluctuation of flow rate through the filter (±3%), and due to variation of the penetration among four trials of experiments (±1%) were considered. Obtained results are shown in and for MWCNTs from Cheaptubes and Baytubes, respectively. The standard deviation was used for error bars for the lengths of CNTs from SEM measurement. The filtration approach showed reasonable results, which were mostly within or close to the uncertainty ranges of measured lengths of CNTs by SEM analysis. The discrepancy for Baytubes was larger than that for Cheaptubes or the mobility models. The possible reasons for the discrepancies are discussed below.
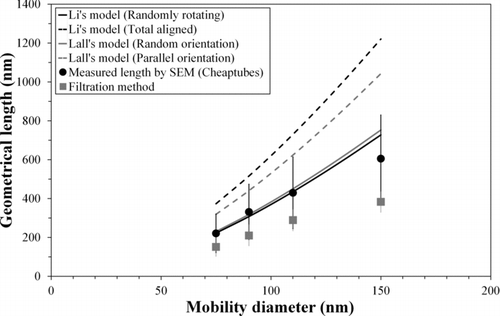
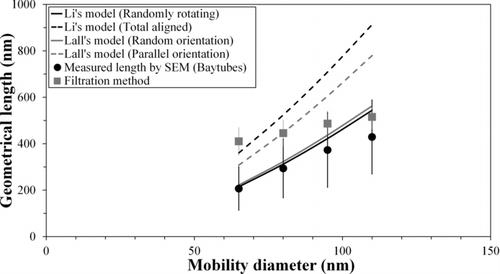
Table 1 TABLE 1 Measured and calculated geometrical lengths of CNTs
The measured length distribution of MWCNTs was compared with theoretical models including Li's model (Li et al. Citation2012) and Lall's model (Lall and Friedlander Citation2006). Comparison with our SEM results showed that the models give satisfactory results. Lall's and Li's model for the random orientation fit especially well with the data of Cheaptubes as shown in . The results of Baytubes () lay slightly below Lall's and Li's models with random orientation. The overview of measured and calculated values of all models is shown in . Diameters used in the models were 25 and 20 nm for Cheaptubes and Baytubes, respectively. Such a difference in CNT diameter led to 25–30% differences between the geometrical lengths of Cheaptubes and Baytubes in the mobility diameter models for 110 nm mobility size.
We evaluated two approaches for fast determination of CNT geometrical lengths. The first one is to measure the CNT mobility size and compute the CNT geometrical length using models related to the mobility diameter and length. Two models (Lall and Friedlander Citation2006; Li et al. Citation2012) were used for this purpose. Our results demonstrated that the models agreed well with experimental results when random orientation of CNTs was assumed. Lall's model was derived for free-molecular flows, thus its application is limited to CNTs with small diameters. Both of the models include adjustable parameters depending on particle orientation and accommodation coefficients. The values of the parameters and coefficients used in the present study worked well for the CNTs under consideration, but may not be optimal for other types of CNTs.
We developed a second approach for CNT length measurement based on filtration. This method showed reasonable agreement with the SEM analysis. The range of uncertainty depends on the filtration experiments. A number of limitations exist for this approach. Bending and curling of CNTs may occur when the aspect ratio is too high (Wang et al. Citation2011b,Citationc) and CNTs may align with the flow when the face velocity is too high (Gallily et al. Citation1986; Gradoń el al. Citation1988). These effects are not included in the filtration model and may lead to underestimation of the CNT length. On the other hand, the filter properties may not be exactly the same as assumed in the model calculations. CNT agglomeration in the filter or loading on the filter may lead to overestimation of its length.
5. CONCLUSIONS
Since the atomizer generation method allowed us to produce sufficient amount of CNTs for the microscopy studies, this was the method used for the generation of airborne MWCNTs from liquid suspensions. Under carefully controlled conditions, the peak in the distribution curve for airborne CNTs was differentiated from the residuals. We were able to control the degree of dispersion by changing the concentration of MWCNTs in the suspension. Better dispersed CNTs were obtained with the lower concentration of the CNT suspensions.
Furthermore, the measured geometrical length by SEM analysis with results from previous experimental studies were compared and it was found out that the diameter of CNTs affected the relation between the geometrical length and mobility diameter. In the free-molecular flow regime, CNTs with smaller diameters possessed longer geometrical lengths when the mobility size was fixed. Further studies on effect of the diameter of CNTs are necessary when the flows are not in the free-molecular range. We compared the geometrical lengths of two types of CNTs, which possessed different diameters, with the models published before: the model originally developed for a chain aggregate with random orientation (Lall and Friedlander Citation2006); and the model for cylinder shaped particles with random orientation (Li et al. Citation2012). They both agreed well with the measured geometrical length of both types of CNTs. This provided an approach to determine the CNT length by the combination of mobility measurement and model calculation. We have developed a new approach to calculate the geometrical length of CNTs using filtration method. Penetration of CNTs through a screen filter was applied to the filtration model, which provides the possibility of fast measurement of the geometrical length of elongated particles. Calculated lengths showed reasonable agreement with the SEM analysis.
This study was partially supported by the Swiss National Science Foundation (NFP 64), “Evaluation platform for safety and environment risks of carbon nanotube reinforced nanocomposites,” 406440 131286. The authors thank the support of members of the Center for Filtration Research: 3M, Boeing, Commercial Airplanes, Cummins Filtration Co., Inc., Entergris, Inc., Hollingworth & Vose Co., MANN+HUMMEL, GMBH, MSP Corp., Samsung Electronics Co., Ltd., Shigematsu Works Co., Ltd., TSI Inc., and W. L. Gore & Associates, Inc., and affiliate member NIOSH.
REFERENCES
- GallilyI.SchibyD.CohenA.H.HollanderW.SchlessD.19865267286
- GradońL.GrzybowskiP.PiłacińskiW.19884312531259
- HuH.ZhaoB.ItkisM.E.HaddonR.C.20031071383813842
- JennerjohnN.Eiguren-FernandezA.PriknodkoS.FungD.C.HirakawaK.S.Zavala-MendezJ.D.et al.20102125255603
- KimS.C.ChenD.R.QiC.GeleinR.M.FinkelsteinJ.N.ElderA.et al.2010414251
- KimS.H.MulhollandG.W.ZachariahM.R.200738823842
- KimS.H.MulhollandG.W.ZachariahM.R.2009a47512971302
- KimS.C.WangJ.EmeryM.ShinW.-G.MullholandG.PuiD.Y. H.2009b434344355
- KimS.H.ZachariahM.R.20051621492152
- KuB.KulkarniP.20091113931403
- LallA.A.FriedlanderS.K.2006373260271
- LamC.W.JamesJ.T.McCluskeyR.ArepalliS.HunterR.L.200636189217
- LangeR.FissanH.Schmidt-OttA.1999166065
- LeeS.LeeJ.H.BaeG.N.200912501512
- LiM.MulhollandG.W.ZachariahM.R.20124610351044
- MyojoT.1999303039
- SetoT.FurukawaT.OtaniY.UchidaK.EndoS.201044734740
- WangJ.201330256266
- WangJ.AsbachC.FissanH.HülserT.KuhlbuschT.A.J.ThompsonD.et al.2011a1313731387
- WangJ.KimS.C.PuiD.Y. H.2011b453443452
- WangJ.KimS.C.PuiD.Y. H.2011c1345654573
- WangJ.PuiD.Y.H.200911185196
- WangX.LiQ.XieJ.JinZ.WangJ.LiY.et al.20099931373141
- WangJ.PuiD.Y. H.2013856976
- WickP.ManserP.LimbachL.K.Dettlaff-WeglikowskaU.KrumeichF.RothS.et al.2007168121131