Abstract
Particle size distributions are of profound interest in the study of ambient aerosols. Electrostatic classification using the Scanning Mobility Particle Sizer (SMPS) and more recently the Fast-Mobility Particle Sizer (FMPS) is the most commonly employed approach to establish particle size distributions for submicron particles in field and laboratory applications. The FMPS enables fast size distribution measurements on a timescale of seconds but has been speculated to underestimate particle size. Aerosol mass spectrometry has emerged as another well-accepted method for size-resolved compositional aerosol analysis with particle sizing being accomplished by flight time separation over a specified flight path under vacuum conditions. In this work, we characterized the particle sizing performance of an FMPS against simultaneous measurements with an Aerodyne Aerosol Mass Spectrometer (AMS) and an SMPS by sampling ambient particles, as well as polydisperse and monodisperse particles from aqueous inorganic salt solutions in the size range from 50 nm to 450 nm. The particle size measurements by AMS and SMPS produced similar results, while the FMPS significantly underestimated particle size by 40–50%. The discrepancy was observed in all studied ambient and laboratory-generated aerosols and appeared to be largely independent of the sampled species. The observations suggest that it is crucial to evaluate the sizing performance of the FMPS against other instruments to ensure an adequate accuracy of the particle size measurements. In this study, a simple postcorrection method for the FMPS measurements was applied, which was able to successfully reduce the initial underestimation.
Copyright 2013 American Association for Aerosol Research
INTRODUCTION
Airborne fine particles (PM2.5) and ultrafine particles (<0.1 μm) are of concern to the scientific community and the general public due to their association with severe health risks, which include premature mortality, and aggravation of respiratory and cardiovascular diseases (Wichmann and Peters Citation2000). Thus, it is necessary to establish ambient particle size distributions on a routine basis for monitoring purposes. Advances in instrumentation for the measurement of particle size distributions in the fine particle range have contributed to a better understanding of the sources and formation mechanisms of these particles. Among the most commonly used particle sizing instruments are the Scanning Mobility Particle Sizer (SMPS), a combination of Differential Mobility Analyzer (DMA) and Condensation Particle Counter (CPC), and more recently the Fast-Mobility Particle Sizer (FMPS), which both employ the principle of electrostatic classification of charged particles. Over the past decade, aerosol mass spectrometry has been increasingly employed for compositional analysis of major organic and inorganic submicron particulate matter components (Drewnick et al. Citation2005; Canagaratna et al. Citation2007), with the ability to acquire species-resolved particle mass size distributions through analysis of particle flight times and particle ensemble mass spectral analysis.
Instrumental comparison studies involving FMPS and SMPS instruments have so far focused on the comparability of the measured particle number concentrations. A previous study (Jeong and Evans Citation2009) reported that although number concentrations obtained from cosampling FMPS and SMPS instruments were significantly correlated, the SMPS measured lower concentrations that were attributed to diffusion losses in the lower size range resulting from a flow rate that is much smaller than that in the FMPS. The study further reported a possible sampling artifact from the FMPS system in the low size range (≈10 nm) and suggested an empirical correction procedure to correct for the counting discrepancy between the two instruments. A comparison of four mobility particle sizers (three SMPS and one FMPS) sampling polydisperse NaCl and diesel soot particles (Asbach et al. Citation2009) showed good sizing agreement among the SMPS systems, but consistently smaller particle sizes (mean deviation of 9–22%) measured by the FMPS. Size-resolved number concentrations varied significantly between different SMPS and FMPS instruments and had an apparent dependency on the flow rate settings of the SMPS instruments. Previous ambient studies (Zhang et al. Citation2005; Sun et al. Citation2010) have inferred particle bulk densities from comparison of the AMS mass-weighted size distribution and the SMPS volume-weighted size distribution. In terms of the overall distributions, SMPS and the AMS showed good interinstrumental agreement in the accumulation size modes (Zhang et al. Citation2005). However, in periods with greater dominance of smaller particles from fresh emissions, the AMS had a tendency to overestimate particle mass. This was attributed to the different responses of the AMS and the SMPS to more irregularly formed particles (such as fresh soot) where AMS-measured vacuum-aerodynamic diameter (D va) would increase while SMPS-measured mobility diameter (D m) would decrease (Jimenez et al. Citation2003; DeCarlo et al. Citation2004).
The FMPS is a relatively recent development that offers an alternative electrostatic particle sizing method to the SMPS. To evaluate its applicability and reliability, particularly with respect to the possibility of using it as a replacement for the SMPS system in ambient and laboratory-based studies, it is of interest to establish the particle sizing performance of the FMPS in direct comparison to other instruments. While previous studies have focused largely on concentration comparisons, this work presents a comparison of particle size measurements using an FMPS instrument (TSI model 3091, TSI Inc., Shoreview, MN, USA), SMPS instrument (TSI Electrostatic Classifier model 3080, TSI DMA column model 3081 and TSI WCPC model 3785, TSI Inc., Shoreview, MN, USA), and an Aerodyne HR-ToF-AMS (Aerodyne Research Inc., Billerica, MA, USA). Ambient particles at a suburban site in Hong Kong and a variety of polydisperse and monodisperse aerosols generated from inorganic salts in the laboratory were sampled by the aforementioned instruments.
Background of Instrument Particle Sizing Principles
Both the SMPS and the FMPS measure particle size according to the mobility of a charged particle in an electric field. As the instrumental and operational details have already been described extensively elsewhere, only a brief general description of the working principles of the two instruments shall be given here. The SMPS system consists of two individual components that can be operated separately: an electrical classifier with a DMA column, and a CPC (Knutson and Whitby Citation1975; Wang and Flagan Citation1990). Sampled particles are neutralized by a bipolar charger to achieve a steady-state distribution of positively and negatively charged particles. The particles and a particle-free sheath air flow enter the DMA column into a space between the outer wall and a central cylindrical electrode; a high negative voltage is applied to the central electrode. Negatively charged particles are repelled toward the outer wall. Neutral particles are transported out with the sheath flow while positively charged particles move toward the inner electrode where they exit through a narrow slit at the downstream end of the critical electrode. This ensures that only particles in a narrow range of electrical mobility exit the instrument. This monodisperse aerosol is subsequently sampled by a condensation particle counter, where particles are exposed to supersaturated water or butanol vapor and grow into large liquid droplets that can be quantitatively detected by a laser light scattering device. From the applied DMA voltage, the particle size (in terms of particle mobility diameter Dm ) is inferred by combining this information with knowledge of the charging probability. Coupled with the particle counting capability of the CPC, size distributions can be acquired. The version of the SMPS used in this study covers a size range of 10 nm to 1 μm, depending on the instrument settings and classifier column. As the measurement of a size distribution requires the instrument to scan through a range of voltages and the particle residence time within the DMA is several seconds, the overall time resolution of the instrument is typically on a timescale of several minutes.
The FMPS is a single-component instrument whose principal design is based on the Electrical Aerosol Spectrometer (EAS) from the University of Tartu (Tammet et al. Citation2002). Particles entering the instrument are first exposed to a negative unipolar corona charger to bring the aerosol to a well-defined charge state. The particles are subsequently positively charged by a second unipolar corona charger to attain a predictable charge distribution. They are then transported with a sheath air flow into the space between two concentric classification electrodes. The central electrode is maintained at a positive high voltage thus repelling particles toward the outer cylinder which is divided into several electrically isolated segments that are connected to individual electrometers. Depending on the electrical mobility, particles will strike different electrometers, i.e., particles of higher electrical mobility will strike electrometers closer to the inlet than will those of lower mobility. From the current recorded by each electrometer and assuming a predictable particle charge distribution, the number size distribution of sampled particles can be inferred. In contrast to the SMPS system, the FMPS system does not need to scan through voltages; hence, it offers a time resolution of about 1 s for each size distribution in a size range of 5–560 nm.
The aerodyne HR-ToF-AMS has been extensively described previously (Drewnick et al. Citation2005; Canagaratna et al. Citation2007). Particle size information is retrieved by analyzing particle flight times in the instrument particle flight chamber. The AMS measures particle size in terms of vacuum aerodynamic diameter (D va). The relationship between the mobility diameter (Dm ) as measured by SMPS and FMPS instruments and the vacuum aerodynamic diameter is (DeCarlo et al. Citation2004):
METHODS
Experimental Section
The data used for the ambient sampling comparison originated from a series of field measurements conducted with a variety of gas- and particle-phase instruments, including a HR-ToF-AMS (Aerodyne Research Inc., Billerica, MA, USA) and an FMPS (TSI model 3091, TSI Inc., Shoreview, MN, USA), at the recently established on-campus air quality supersite at the Hong Kong University of Science and Technology (HKUST) in April and May 2011. The AMS was size-calibrated using NanosphereTM PSL particles (Duke Scientific, Palo Alto, CA, USA) of sizes in the range of 80–800 nm before and after the sampling campaign. The FMPS cannot be calibrated for sizing and does not permit changes to any settings that would affect the recorded particle size. Thus, the only adjustments to the instrument were to zero the electrometers and to clean the impactor and charger needles as recommended by the manufacturer at 3–5 day intervals.
For the laboratory-based particle sampling experiments, aqueous solutions were prepared using double deionized (DDI) water and standard ammonium nitrate NH4NO3, ammonium sulfate (NH4)2SO4, ammonium bisulfate NH4HSO4, ammonium chloride NH4Cl, and sodium chloride NaCl stock chemicals (Sigma-Aldrich, St. Louis, MO, USA; Riedel de Haën, Seelze, Germany) as well as a variety of polystyrene latex (PSL) NanosphereTM (Duke Scientific, Palo Alto, CA, USA) particle solutions in the size range of 50–450 nm. PSL particles are commonly used as size calibration standards, including in the size calibration of the AMS. For the inorganic salts, 100 ml of aqueous solutions at a concentration of 0.3% w/w were prepared; 25 ml of PSL solutions containing 5–6 drops of PSL stock and DDI water were sonicated to disperse the PSL particles. The instrumental set-ups are illustrated in . The solutions were atomized with a BMI (Brechtel Manufacturing Inc., Hayward, CA, USA) atomizer operated at 100 kPa, equipped with a syringe pump for solution injection. The generated aerosol was passed through a 1 m long diffusion drier filled with silica gel to remove bulk gas- and particle-phase water and charges acquired during atomization were dissipated using a Kr neutralizer.
FIG. 1 Instrumental setup for sampling various monodisperse and polydisperse aerosols and PSL particles by (top to bottom): FMPS, SMPS (DMA+CPC), AMS and SMPS (DMA+CPC) simultaneously, and FMPS (with DMA size-selected particles).
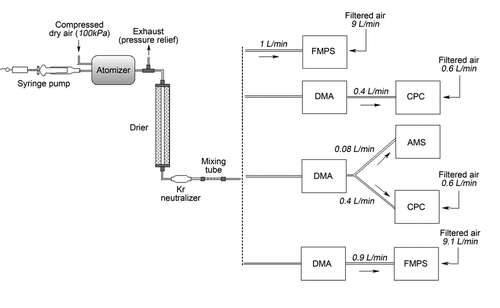
The sample flow was either directed into an FMPS (TSI model 3091, TSI Inc., Shoreview, MN, USA) at a flow rate of 1 L/min with a remaining 9 L/min make-up air drawn through a HEPA filter, into the AMS with a nominal flow rate of 0.08 L/min, or the SMPS (TSI Electrical Classifier model 3080, TSI Differential Mobility Analyzer model 3081, and TSI Water-based Condensation Particle Counter WCPC model 3785, TSI Inc., Shoreview, MN, USA) at a sample flow rate of 0.4 L/min with 0.6 L/min make-up air drawn through a HEPA filter at the CPC. For the monodisperse inorganic salt (sodium chloride, ammonium nitrate, ammonium bisulfate, and ammonium sulfate) aerosol experiments, atomized particles were first size-selected by the DMA (TSI Electrostatic Classifier model 3080 and TSI DMA column model 3081, TSI Inc., Shoreview, MN, USA) and subsequently sampled by the FMPS, with the FMPS size distributions manually averaged over a sampling period of 2 min. The AMS measurements were conducted using the Unit Mass Resolution mode (MS V-mode) and the Particle Time-of-Flight mode (PToF-mode) in conjunction with dwell times of 10 s (MS V-mode) and 20 s (PToF-mode), respectively, for a total sampling time of 5 min. SMPS size distribution scans at a sample flow of 0.4 L/min and a sheath flow of 4 L/min permitted for a size range of 12.6 to 552.3 nm with a scan time of 120 s and a 30 s delay period for voltage recovery. This corresponds to a total time of 150 s between subsequent scanning cycles. FMPS size distributions were acquired every second, with the data acquisition software averaging 20 s for each reported size distribution. The total sampling time for the FMPS varied between 2 min and 5 min. The FMPS and SMPS instruments yielded both number and volume size distributions, while the AMS provided mass size distributions only.
Data Treatment
The vacuum aerodynamic diameter from the AMS mass size distributions was converted back to the mobility particle diameter using EquationEquation (1). For ammonium nitrate, a density ρ NH4NO3 = 1.72 g/cm3 and a shape factor S = 0.8 (Jayne et al. Citation2000) were used. The same data treatment was performed on ammonium sulfate and ammonium chloride using their respective bulk densities (ρ (NH4)2SO4 = 1.79 g/cm3, ρ NH4Cl = 1.53 g/cm3), and setting S = 1 due to the lack of particle shape information. The mass-based distribution (in terms of dM/dlogDp ) was converted to a volume-based distribution (dV/dlogDp ) using the same bulk densities.
RESULTS AND DISCUSSION
Ambient AMS and FMPS Measurements
The monthly averaged FMPS volume size distribution yielded a mode diameter of 190 nm, while the corresponding monthly AMS mass size distribution mode averaged at 570 nm (). Applying EquationEquation (1) to approximate the bulk particle density from these measurements gives an average particle density of 3.0 g/cm3. Considering the monthly observed average species composition with a ratio of nitrate:organics:sulfate of 1:6:10, assuming that nitrate and sulfate are in their respective ammonium salt forms NH4NO3 and (NH4)2SO4 and that organic components had an average density of 1.20 g/cm3, an approximate particle density can be calculated by using the simple reciprocal mixing rule:
This would yield an approximate bulk particle density of ρp = 1.52 g/cm3, which is only about half of that calculated from the AMS and FMPS data. While inferred densities of 1.8 g/cm3 have been reported in ambient studies (He et al. Citation2011) using AMS mass and SMPS volume size distributions, the observed value in this work is outside of reasonable expectations. Thus, the comparison of AMS- and FMPS-derived ambient size distributions as presented here, indicates a significant discrepancy between the particle sizes reported by the AMS and FMPS under ambient sampling conditions.
FIG. 3 (a) Volume size distributions from SMPS, FMPS, and AMS for the polydisperse ammonium nitrate NH4NO3 aerosol. AMS D va has been converted to Dm . Corrected FMPS volume size distribution refers to the FMPS volume size distribution after application of the sizing correction algorithm. (b) Volume size distributions from SMPS, FMPS, and AMS for the polydisperse ammonium sulfate (NH4)2SO4 aerosol. AMS D va has been converted to Dm . Corrected FMPS volume size distribution refers to the FMPS volume size distribution after application of the sizing correction algorithm. (c) Volume size distributions from SMPS, FMPS, and AMS for the polydisperse ammonium chloride NH4Cl aerosol. AMS D va has been converted to Dm . The corrected FMPS volume size distribution refers to the FMPS volume size distribution after application of the sizing correction method. Lognormal peak fitting was performed on the AMS Chl distribution due to the noisy character of the distribution. (d) FMPS- and SMPS-measured volume size distribution peak mode diameters of the polydisperse aerosols (before and after application of the sizing correction method). (Color figure available online.)
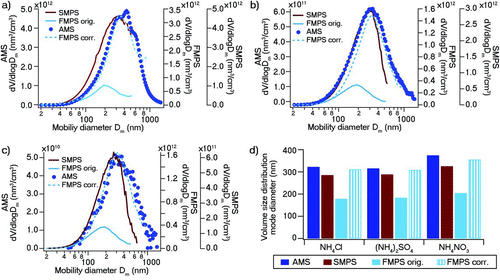
Polydisperse Inorganic Aerosol
Polydisperse ammonium nitrate NH4NO3, ammonium sulfate (NH4)2SO4, and ammonium chloride NH4Cl aerosols were sampled with their number and volume size distributions acquired by the FMPS and SMPS systems and their mass size distribution measured by the AMS. The distributions for NH4NO3, (NH4)2SO4, and NH4Cl are depicted in , respectively. For all three tested polydisperse aerosols, the SMPS volume distributions extend to slightly smaller sizes than do the mass size distributions from the AMS. This is likely due to the difference in flow rate between the AMS and the SMPS, with the flow rate of the AMS being much lower and hence more susceptible to diffusion loss in the sampling line than the SMPS. This resulted in the SMPS mode diameters appearing slightly shifted toward smaller sizes by 10–17% as compared to the AMS mode diameters (). The observed discrepancy between the FMPS measured (original) volume distribution mode diameter and that from the AMS is much more severe with an underestimation of 40–50%. Overall, the difference in mode diameter of <20% between the SMPS results and the AMS results is reasonable considering the uncertainties arising from possible diffusion loss in the AMS sampling line and the neglect of the shape factor correction particularly for the chloride and sulfate particles. However, the FMPS displayed an unexpectedly large negative bias compared to both the AMS and the SMPS.
DMA Size-Selected Particles
Previous studies (Asbach et al. Citation2009; Jeong and Evans Citation2009) used 100 nm PSL particles to verify the sizing accuracy of the FMPS, with Asbach et al. reporting an underestimation of the particle size by about 20% while Jeong et al. did not observe any significant over- or underestimation by the FMPS. A general problem with PSL particle dispersions is the relatively low concentration of actual latex spheres and the large number of differently sized surfactant particles generated during atomization. A further complication is the large sampling flow rate of the FMPS (10 L/min), which requires the usage of a particle-free dilution flow in addition to the particle-flow from the atomizer. This diminishes the PSL particle peak. Using the DMA to preselect the PSL particles does not yield appreciable amounts of PSL particles that can be adequately resolved by the FMPS due to the large dilution and the comparatively low overall PSL concentration in the atomized dispersion.
Atomized salt solutions yield much higher particle concentrations and sufficiently intense size distributions despite the large dilution and circumvent the problem of interfering surfactant particles. Particles from polydisperse inorganic aerosols (sodium chloride, ammonium nitrate, ammonium bisulfate, and ammonium sulfate) were size-selected by the DMA and sampled with the FMPS. The sizing accuracy of the DMA was verified by sampling PSL particles in the range of 50–450 nm. depicts the plot of resolved PSL particle peak diameters from the SMPS measurements against the true PSL particle size, confirming the excellent sizing capability of the SMPS with a slope of 0.98 and R 2 = 0.99. shows a typical number size distribution, obtained for DMA size-selected NH4NO3 particles of 90 nm in diameter that were sampled by the FMPS. It is evident that despite the monodisperse character of the sampled aerosols, the size distributions measured with the FMPS were still relatively broad. While the observed mode diameters varied slightly with different chemical composition of the particles, there was no clear trend suggesting that the measured particle size was independent of the chemical identity of the aerosol. The average of the mode diameters observed for differently composed particles at the same DMA-selected size was linearly fitted to the DMA-selected size (). The degree of correlation was excellent with R 2 = 0.99. The observed slope of 0.56 was significantly smaller than expected and suggests a significant mode diameter underestimation by the FMPS used in this study for particles ≥50 nm in size. Particles <50 nm in diameter were not measured in this study, which precludes a judgment on the sizing capability in the smallest size range.
FIG. 4 Plot of observed SMPS number size distribution mode diameter versus actual PSL particle size. A linear least squares fit with correlation coefficient R 2, slope b, and intercept a is also depicted. (Color figure available online.)
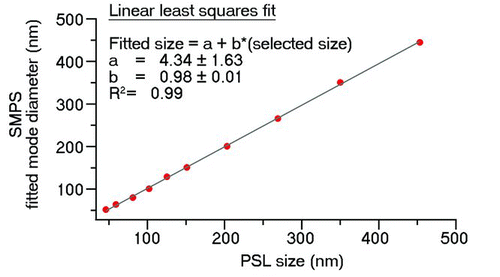
FIG. 5 (a) Example of an FMPS-acquired ammonium nitrate NH4NO3 particle number size distribution (selected DMA particle size: 90 nm) with lognormal peak fitting. (b) Plot of observed FMPS number size distribution mode diameter versus selected DMA particle size of various inorganic aerosols. A linear least squares fit using an all-species average value for each size with correlation coefficient R 2, slope b, and intercept a is also depicted. (Color figure available online.)
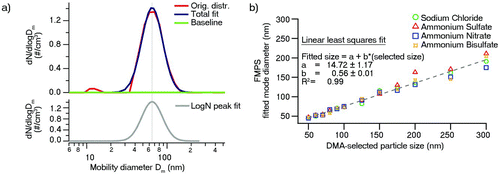
FIG. 6 Comparison of ambient FMPS and SMPS measurements acquired at a suburban site in Hong Kong in February 2012. (a) Time series of measured total particle concentration. (b) Plot of number size distributions averaged over the two sampling periods, with the FMPS distribution after sizing correction as a dotted line. (c) Plot of volume size distributions averaged over the two sampling periods, with the FMPS distribution after sizing correction as a dotted line. (Color figure available online.)
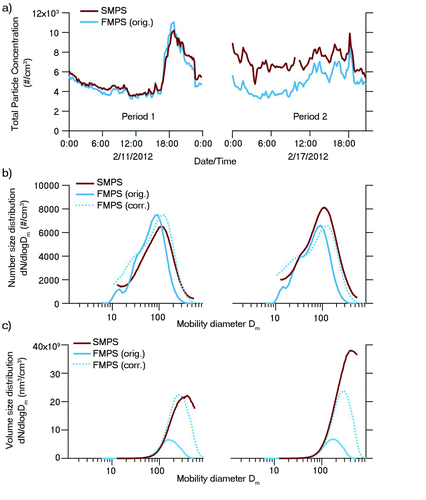
Correction of Polydisperse Particle Size Distribution
Using the slope and intercept from the linear least squares fit of the salt experiments (from ), a simple correction algorithm for the FMPS volume size distribution was applied. The correction only shifts the midpoint diameter of the FMPS size acquisition bins while keeping the bin width unmodified. Due to the positive intercept, the size bins below 15.8 nm in particle size were discarded to avoid producing negative data points. The corrected size bins were then used to convert the original number concentration function F(N) for each bin into a new volume size distribution function F(V) according to the following equation:
Concentration Comparison
SMPS and FMPS were directly compared in terms of the total measured particle concentration, with ambient data acquired at the HKUST supersite in February 2012. Within the measurement period, the agreement between SMPS and FMPS differed as a function of time and thus two representative one-day periods were chosen for further study: period 1 spanning the 11th of February and period 2 spanning the 17th of February. The total particle concentrations measured by FMPS and SMPS agreed very well in period 1, but differed significantly in period 2, in which the number concentration measured by the FMPS was on average 40% lower than that of the SMPS (). This confirms the reported discrepancies in particle counting in previous studies (Asbach et al. Citation2009; Jeong and Evans Citation2009). As apparent from the number () and volume size distributions (), the agreement in the particle number and volume concentrations improved significantly after applying the FMPS sizing correction (dotted line). However, the size correction cannot completely eliminate the original difference between the SMPS and FMPS measurements, as the overall discrepancies between SMPS and FMPS size distributions are very likely a combined result of accuracy issues in both sizing described in this work as well as particle counting described in previous studies.
CONCLUSION
A comparison of the volume size distributions of polydisperse and monodisperse inorganic aerosols measured by a TSI SMPS and a TSI FMPS as well as the mass size distribution acquired by an Aerodyne HR-ToF-AMS has shown that the SMPS and the AMS produced similar particle sizing results, while the FMPS underestimated particle size significantly with respect to the other two instruments. On average, the FMPS underestimated the particle size by 40–50%. The observations highlight the importance of evaluating the sizing performance of the FMPS and cross-validating the acquired data from the FMPS with those obtained with other instruments, particularly since the FMPS by default is not delivered with any tool for size calibration. Postcorrection of the negatively biased size mode diameters from the FMPS by employing a previously size-calibrated DMA to produce a variety of monodisperse particles for sampling by the FMPS is however possible and was shown to be capable of considerably reducing the initial sizing discrepancy.
Acknowledgments
Funding support from the University Grants Committee of Hong Kong (Special Equipment Grant SEG-HKUST07), the Environment and Conservation Fund (ECF ECWW09EG04), and the Research Grants Council of Hong Kong (Hong Kong PhD Fellowship Scheme, for Berto P. Lee) is gratefully acknowledged.
REFERENCES
- Asbach , C. , Kaminski , H. , Fissan , H. , Monz , C. , Dahmann , D. and Mülhopt , S. 2009 . Comparison of Four Mobility Particle Sizers with Different Time Resolution for Stationary Exposure Measurements . J. Nanopart. Res. , 11 : 1593 – 1609 .
- Canagaratna , M. R. , Jayne , J. T. , Jimenez , J. L. , Allan , J. D. , Alfarra , M. R. and Zhang , Q. 2007 . Chemical and Microphysical Characterization of Ambient Aerosols with the Aerodyne Aerosol Mass Spectrometer . Mass Spectrom. Rev. , 26 : 185 – 222 .
- DeCarlo , P. F. , Slowik , J. G. , Worsnop , D. R. , Davidovits , P. and Jimenez , J. L. 2004 . Particle Morphology and Density Characterization by Combined Mobility and Aerodynamic Diameter Measurements. Part 1: Theory . Aerosol Sci. Technol. , 38 : 1185 – 1205 .
- Drewnick , F. , Hings , S. S. , DeCarlo , P. , Jayne , J. T. , Gonin , M. and Fuhrer , K. 2005 . A New Time-of-Flight Aerosol Mass Spectrometer (TOF-AMS)—Instrument Description and First Field Deployment . Aerosol Sci. Technol. , 39 : 637 – 658 .
- He , L.-Y. , Huang , X.-F. , Xue , L. , Hu , M. , Lin , Y. and Zheng , J. 2011 . Submicron Aerosol Analysis and Organic Source Apportionment in an Urban Atmosphere in Pearl River Delta of China Using High-Resolution Aerosol Mass Spectrometry . J. Geophys. Res. Atmos. , 116 : D12304
- Jayne , J. T. , Leard , D. C. , Zhang , X. , Davidovits , P. , Smith , K. A. Kolb , C. E. 2000 . Development of an Aerosol Mass Spectrometer for Size and Composition Analysis of Submicron Particles . Aerosol Sci. Technol. , 33 : 49 – 70 .
- Jeong , C.-H. and Evans , G. J. 2009 . Inter-Comparison of a Fast Mobility Particle Sizer and a Scanning Mobility Particle Sizer Incorporating an Ultrafine Water-Based Condensation Particle Counter . Aerosol Sci. Technol. , 43 : 364 – 373 .
- Jimenez , J. L. , Bahreini , R. , Cocker , D. R. , Zhuang , H. , Varutbangkul , V. and Flagan , R. C. 2003 . New Particle Formation from Photooxidation of Diiodomethane (CH2I2) . J. Geophys. Res. –Atmos. , 108 : 4318 – 4343 .
- Knutson , E. O. and Whitby , K. T. 1975 . Aerosol Classification by Electric Mobility: Apparatus, Theory, and Applications . J. Aerosol Sci. , 6 : 443 – 451 .
- Sun , J. Y. , Zhang , Q. , Canagaratna , M. R. , Zhang , Y. M. , Ng , N. L. and Sun , Y. L. 2010 . Highly Time- and Size-Resolved Characterization of Submicron Aerosol Particles in Beijing Using an Aerodyne Aerosol Mass Spectrometer . Atmos. Environ. , 44 : 131 – 140 .
- Tammet , H. , Mirme , A. and Tamm , E. 2002 . Electrical Aerosol Spectrometer of Tartu University . Atmos. Res. , 62 : 315 – 324 .
- Wang , S. C. and Flagan , R. C. 1990 . Scanning Electrical Mobility Spectrometer . Aerosol Sci. Technol. , 13 : 230 – 240 .
- Wichmann , H.-E. and Peters , A. 2000 . Epidemiological Evidence of the Effects of Ultrafine Particle Exposure . Philos. Trans. R. Soc. Lond. Ser. A, Math. Phys. Eng. Sci. , 358 : 2751 – 2769 .
- Zhang , Q. , Canagaratna , M. R. , Jayne , J. T. , Worsnop , D. R. and Jimenez , J. L. 2005 . Time- and Size-Resolved Chemical Composition of Submicron Particles in Pittsburgh: Implications for Aerosol Sources and Processes . J. Geophys. Res. –Atmos. , : 110