Abstract
Bioaerosol detection and identification systems need to be periodically checked for assurance that they are responsive to aerosol challenges. Herein, pressurized metered dose inhalers (pMDIs) containing ethanol suspensions of two simulants for B. anthracis spores are considered for providing suitable aerosols. Doses and shot weights from pMDIs with canisters having volumes equal to that of 200 metering-valve actuations were constant for ≤165 actuations, but drop beyond that range. There were statistically significant dose variations between replicate pMDIs and between two types of actuators used on the pMDIs. The storage half-lives of pMDIs filled with Bacillus atrophaeus (BG) and Bacillus thuringiensis subsp. israelensis (Bti) spore formulations are predicted to be 32 and 136 months, respectively, if the canisters are stored under refrigeration (4°C). The prediction is based on use of a logarithmic regression model relating CFU per actuation to storage time, with data taken at times of 1–12 months. Demonstration of the utility of the concept was provided by producing responses from a polymerase chain reaction (PCR) identifier with pMDI-generated BG and Bti aerosols that were collected with a 100 L/min wetted wall bioaerosol sampling cyclone.
Copyright 2013 American Association for Aerosol Research
1. INTRODUCTION
1.1. Background
Regular functionality checks are needed for field-deployed near-real-time bioaerosol detection (e.g., fluorescent aerosol particle detectors) and identification (e.g., polymerase chain reaction, PCR) systems to determine if they will respond to bioaerosol challenges. Acceptable functionality is an instrumentally provided indication of the presence of a challenge without regard to the accuracy of the correlation between magnitude of the aerosol input and the level of the instrument response. The approach considered herein for providing aerosols suitable for functionality checking, is the use of variants of medical pressurized metered dose inhalers (pMDIs), which are normally employed as delivery devices for inhalation-effective
pharmaceuticals.
The pMDI concept, , is inexpensive, self-contained, easy to operate, lightweight, and portable. Regarding the performance of pMDIs containing pharmaceuticals in the particulate phase, Rubin and Fink (Citation2005) note that a pMDI can provide consistent doses (amount of pharmaceutical released per actuation) for a certain number of actuations; however, smaller doses will be produced by subsequent actuations. Graham et al. (Citation1992), Everard et al. (Citation1995), and Byron (Citation1997) measured single-actuation drug doses and observed doses were reduced when the pressurized container was stored and not shaken before use, and the degree of reduction depended on orientation during storage. The drug particles in a suspension can undergo sedimentation, so patients are instructed to shake inhalers immediately before use (Byron Citation1997). With earlier pMDIs, which used chlorinated fluorinated hydrocarbons as propellants, the temperature of the pressurized container would affect the dose of medication, with lower temperatures causing smaller doses to be delivered (Rubin and Fink Citation2005). For more recent pMDIs, which use hydrofluoroalkane (HFA) propellants, the dose may not be substantially affected by temperatures as low as −20°C (June et al. Citation1996).
FIG. 1 A pressurized medical metered dose inhaler (pMDI). The device comprised of the canister, metering valve, and stem is referred to as the pressurized dispensing container (PDC).
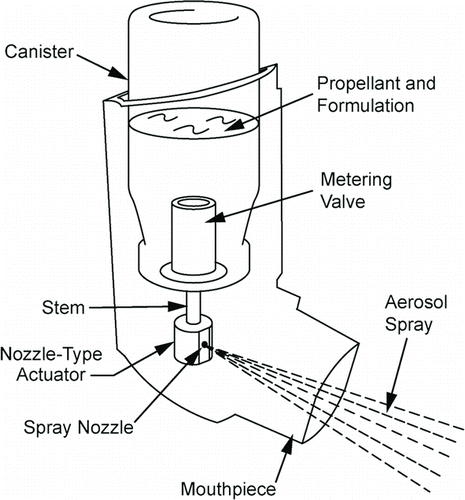
The need for priming a pMDI (discarding one or more initial doses) that contains a drug suspension was discussed by Cry et al. (Citation1991), who showed substantially lower and more variable drug doses in the first, as compared with subsequent actuations. The shot weight (weight output of propellant plus formulation per actuation) was the same for the first, second, and third actuations, so the metering valve of the pMDI performed its function properly in dispensing a constant volume of liquid; however, the reduced dose from the first actuation suggests some of the particulate matter for that actuation had been lost on the internal walls of the flow system. The need to both re-suspend drug particles and prime a pMDI device is well established and those procedures should be a part of any protocol for generating bioaerosols with pMDIs.
Variations between pharmaceutical doses of pMDI units were considered in previous studies. Byron (Citation1994) reported significant variations in albuterol doses between devices that had been stored different orientations. Cry et al. (Citation1991) reported no significant dose differences among albuterol-filled pharmaceutical production units on either a lot-to-lot or a within-lot basis. However, because of concerns about variations, U.S. Pharmacopeia set restrictions on pharmaceutical dose variations (Schumacher and Lelner Citation2012; USP Citation2012) where in tests with 10 separate devices, 9 of the 10 doses must be between 75% and 125% of the specified target dose, and none must be outside the range of 65% to 135% of the target dose.
The pMDI technology has been explored for generating non-pharmaceutical aerosols. Vervaet and Byron (Citation2000) filled canisters of pMDIs with 1, 3, 5, and 8 μm fluorescent polystyrene microspheres, and showed suspensions were stable for six months at room conditions. Carrera et al. (Citation2005) prepared devices with Bacillus atrophaeus (aka Bacillus globigii, BG) spores, which are used as a simulant for pathogenic Bacillus anthracis spores because of their similar size and physical properties, to test methodology for quantifying the number of spores in bioaerosol clusters formed from aerosolizing BG suspensions.
1.2. Overview of This Study
The goal of this study was to investigate the pMDI concept with respect to its ability to produce bioaerosols that could be used for functionality testing of bioaerosol detection and identification systems. The testing involved pMDIs filled with formulations comprised of propellant and ethanol suspensions of Bacillus spores, although some shot weight tests were conducted with pMDIs filled only with propellant. Measurements were made of the size distributions of the pMDI output aerosols, and tests were conducted to characterize the uniformity of the shot weights and aerosol doses with usage (number of actuations). Here, in the biological context, dose refers to the number of colony forming units (CFU) resulting from a single actuation. The effects on dose of the type of actuator and the variation between replicate devices were examined as were the effects on dose of storage time and temperature. We used pMDIs in demonstrating functionality testing of a laboratory bioaerosol identification system comprised of a wetted wall cyclone (WWC) and a PCR analyzer.
2. METHODS AND MATERIALS
The pMDIs used in this study, , were comprised of components manufactured by Bespak (Bespak Pharmaceutical, Cary, NC, USA). The canisters have nominal capacity of 10 mL and were filled by means of a Pamasol P2005 small-scale production unit (Pamasol Willi Mäder AG, Pfäffikon,
Switzerland). Each aluminum canister (5.06 g) was filled with 11.72 g (9.55 mL) of HFA 134a propellant to which was usually added a 0.5 mL suspension of either BG or Bacillus thuringiensis subsp. israelensis (Bti) spores in ethanol.
The BG spores were obtained in the form of dry powder from Dugway Proving Ground (Lot 040, Dugway Proving Ground, UT) and the Bti spores were grown in the laboratory using a procedure similar to that of Kreuzer-Martin et al. (Citation2003). Single colonies of Bti were used to inoculate flasks containing 650 mL of Schaeffer's Sporulation Medium (Harwood and
Cutting Citation1990). These were cultured at 37°C for 6 days, at which time there was approximately 97% sporulation. The spores were harvested by centrifuging the cultures at 8,000 g's for 15 min at a temperature of 4°C. They were rinsed, re-centrifuged four times, and heat-shocked at 70°C for 30 min. The Bti spores were recovered by centrifugation and re-suspended in ethanol. At the time of use, the BG powder was rinsed in ethanol and then centrifuged for 5 min at 2,300 g's. The supernatant was removed and the bacterial sediment was re-diluted in ethanol. The concentration of BG and Bti spores in 0.5 mL ethanol suspensions could be varied to provide different aerosol dose levels.
Using ethanol for both processing and storage of Bacillus
spores is considered safe regarding the survivability of spore-forming bacteria. Bacillus spores are resistant to ethanol (Sneath et al. Citation1986; Russell Citation1990) and it is common practice to store Bacillus spores in 40% ethanol at 5°C (Szabo et al. Citation2007; Hosni et al. Citation2011). The spores would start to germinate in the presence of water/aqueous solutions; however, in the absence of nutrients, the fresh vegetative cells would die (Driks Citation2003).
The metering valves have a nominal output of 50 μL/actuation (Model BK357, Bespak Pharmaceutical), which would ideally provide about 200 dose actuations for the approximately 10 mL volume of the canisters. The preparation process used in this study followed that of Byron (Citation1994). The spore-ethanol suspension was added to a canister, a gasketed lid containing the metering valve was crimped to the canister to form the pressurized dispensing container (PDC), and, the propellant was added. The stem of the metering valve protrudes from the PDC () and actuation is accomplished by movement of the stem into the valve, which releases the contents of the metering valve.
2.1. pMDI Delivery Methods
Differently configured pMDIs were employed in this experimental study, which with reference to and , are:
FIG. 2 Alternate configurations of pMDI devices used in study: (a) a pressurized dispensing container (PDC) with no actuator; (b) a PDC with a nozzle-type actuator; (c) a PDC with a hub-type actuator that was slip-fit over the stem and locked in place with a setscrew.
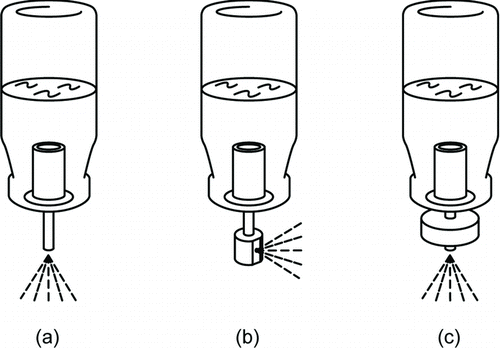
• | The classical pMDI with the mouthpiece and nozzle-type actuator (), where the latter included a Bespak spray nozzle (port size of 0.50 mm diameter), which discharged aerosol through the mouthpiece at a right angle to the axis of the PDC. The nozzle-type actuator is an integral part of the mouthpiece, so in this configuration actuation was accomplished by moving the mouthpiece relative to the PDC. | ||||
• | The basic PDC with no actuator (), where aerosol was released directly from the metering valve stem, which has an inside diameter of 2 mm. | ||||
• | The PDC of fitted with a nozzle-type actuator (). Nozzle-actuators were obtained by separating them from the mouthpiece component shown in . | ||||
• | The PDC of fitted with a disk-shaped actuator that was slip-fit over the end of the valve stem (3.2 mm outside diameter) and fixed in place on the stem (). The arrangement will be referred to as a hub-type actuator. |
2.2. Size Distributions of Aerosol Particles
An Aerodynamic Particle Sizer (APS, Model 3321, TSI Inc., Shoreview, MN, USA) was used to measure the size distributions of BG and Bti aerosols generated with the classical pMDI shown in . Before a test was conducted, the pMDI was either hand shaken for 5 s, or the PDC was removed, ultrasonicated, re-inserted into the pMDI, and then later hand shaken for 5 s. The pMDI was primed three times and then actuated with the aerosol output delivered into a 118 L chamber, from which samples were drawn into the APS. The particle size results are presented in terms of the APS-determined geometric means and standard deviations of the number distributions.
Comparisons were made of the aerosols produced by pMDIs that had been ultrasonicated and shaken before use with those generated from pMDIs that had only been shaken. Also, because the port size of the nozzle actuator is 0.5 mm and the inner diameter of the stem in the hub actuator is 2 mm, a test was conducted to compare the size distributions of the residual aerosols produced by the two actuation methods.
Electron photomicrographs were taken of BG and Bti spores that had been collected on carbon tape substrates in an impactor (BioStage Single-stage Impactor, SKC Inc., Eighty Four, PA, USA). These photographs were used to qualitatively illustrate the spore shapes.
2.3. Shot Weights of pMDIs
The shot weights produced by one classical pMDI () filled with only propellant and a second pMDI filled with propellant plus BG formulation and fitted with various actuators (, , and ), were measured as a function of usage (number of actuations). Measurements of the PDC weight of the unit filled with propellant-only were made after every actuation, whereas measurements of the unit filled with BG formulation were made after every 10 actuations.
2.4. Balloon Collection Method
Various methods have been used for collecting the aerosol doses produced by pMDIs. For example, Phillips et al. (Citation1990) used impaction and impingement to collect aerosols generated from albuterol formulations; and, Kamiya et al. (Citation2004) used two types of impactors in a study that examined the aerosol size distributions. Herein the doses from the test devices were characterized by use of a balloon collection method. This method was selected to help preserve the culturability of the organisms, to capture the complete aerosol plumes emitted by the devices, and to accommodate the collection of large numbers of samples that needed to be analyzed.
A washed 305 mm (12-inch) transparent latex balloon (Celebrity Balloons, San Antonio, TX, USA) was partially filled with 10 mL of phosphate buffered saline plus 0.01% Triton X-100 (PBST, Sigma Chemical Co., St. Louis, MO, USA). The output of a pMDI was released directly into the balloon; and, the balloon was then disconnected from the pMDI and shaken for 5 s. It was allowed to rest for 20 min to permit the aerosol particles to migrate to the surfaces. After the 20-min period, the contents of the balloon were vigorously mixed to re-suspend the deposited particles, and the hydrosol transferred to a 50-mL conical tube for subsequent analysis. Only the pMDIs shown in were used in tests with the balloon method because the pMDIs with mouthpiece and nozzle-type actuator () are open to the atmosphere at the back of the mouthpiece, which would allow aerosol flow to be vented to the ambient air during the actuation process. Validation tests were conducted to determine the recovery of Bti spores from balloons and the results showed the recovery to be 84 ± 11%, where the value following the ± symbol is the standard deviation.
2.5. Culture Analysis
Classical microbiological methods (Madigan and Martinko Citation2006) were used to quantify the CFU associated with Bti and BG tests. The hydrosol from a balloon, which had been transferred to a 50-mL conical tube, was mixed using a vortexer (VWR, Thorofare, NJ, USA) for 5 s. Either 100 or 200 μL of the hydrosol was pipetted onto 100 mm diameter Petri dishes containing Tryptic soy agar (Becton, Dickinson and Co., Sparks, MD, USA) and incubated at 37°C for 12–16 h. After incubation, the CFU were enumerated.
2.6. Dose Constancy and the Variability Among
Similar PDCs
Four pMDIs without actuators () were tested to determine the constancy of dose. These were filled with Bti suspensions that would ideally (based on CFU in the fill formulation) provide doses of 104 CFU/actuation. They were actuated 200 times, with balloon samples collected from two of each set of 10 actuations. Classical microbiological analyses were performed on the samples.
The results are presented as a Biodose Ratio, Rd, bio, which is the ratio of the CFU output per actuation divided by the CFU per actuation that would have been output based on the CFU in the stock fill suspension and the actuation volume, that is,
2.7. Effect of Use of Nozzle-Type and Hub Actuators and the Among-Device Variability
Tests were conducted with BG to determine if dose was affected by the use of a nozzle-type actuator () versus a hub-type actuator (). In these experiments, the variability among replicate canisters was also characterized through use of four pMDIs configured with nozzle-type actuators and four with axial-flow hub-type actuators. Fourteen samples were collected from each pMDI over the span of 4–161 actuations where the first sample collected was from the 4th and 5th actuations and the last sample was collected from the 160th and 161st actuations. The last sample is intended to represent the midpoint of the actuation range from 154.5 to 166.5 actuations. The balloon method was used to collect the aerosol and the samples were analyzed by classical microbiological culturing. The results are presented in terms of CFUact.
2.8. Effect of Storage Time and Temperature
on CFU Output
Ten Bti-filled and ten BG-filled pMDIs were used in these tests. The PDCs of five of the Bti-filled and five of the BG-filled pMDIs were stored in a refrigerator (4°C) and the remaining PDCs were stored at laboratory temperature (24°C). Tests were conducted to determine dose output per actuation, CFUact, at times of 1, 2, 3, 5, 6, 9, and 12 months after filling. Samples were acquired with the balloon collection method, and analyses were performed using classical microbiological culturing. The pMDIs were operated without actuators in the configuration shown in .
2.9. Laboratory Demonstration of Functionality Testing
Functionality testing is intended to be conducted on fielded bioaerosol detection and identification systems that could provide critically needed information regarding malevolent bioaerosol releases. Because of data sensitivity concerns, rather than using a fielded system, we illustrate the functionality of the pMDI approach with a laboratory bioaerosol identification system that was comprised of a 100 L/min wetted wall bioaerosol cyclone sampler (McFarland et al. Citation2010) and a real-time PCR (AB StepOne RT-PCR System, Foster City, CA, USA). The experimental protocol for sample collection and analysis followed that of King and McFarland (Citation2012). For each test, five actuations from a pMDI containing BG or Bti, which had been stored at 4°C, were dispersed into a 203 mm diameter × 2.1 m long that was connected to the WWC. The liquid outflow (0.1 mL/min) from the WWC was collected and measured.
Although the intent of the experiments was to demonstrate the utility of pMDI devices in checking functionality of bioaerosol detection and identification systems, for the combination of sampling with a WWC and analyzing with a real-time PCR (RT-PCR), it was also possible to acquire numerical values for the outcomes of the tests. This was accomplished by first analyzing the output of the pMDIs using the balloon collection method followed by enumeration of cells through classical cell culturing. The results from the RT-PCR analyses were converted to CFU though use of the method employed by King and
McFarland (Citation2012).
2.10. Statistical Analyses
The results of this study generally show the value of a statistical random variable, for example, CFUact, as a function of one or more independent variables such as the length of time and the temperature at which a pMDI device was stored. Where possible, we used Excel Add-In Analysis Toolpack (Office 2010 Professional Academic Version, Microsoft Corp., Redmond, WA, USA) to evaluate the importance of different treatments of the independent variables. If the number of tests was not the same for all statistical treatments or if the number of independent variables exceeded the limit of two for the Excel Toolpack, we used Minitab 16 statistical software (Minitab Inc., State College, PA, USA). Statistical null hypotheses would be rejected if p < .05.
3. RESULTS AND DISCUSSION
3.1. Size Distributions
The average APS-measured size parameters from devices with BG formulation, which were either shaken-only or sonicated and shaken, were a geometric number mean of 0.75 μm AD and a geometric standard deviation of 1.27, . Similarly, for Bti, the average size parameters of the shaken-only and the sonicated and shaken pMDI aerosols, were a geometric mean of 0.70 μm AD and a geometric standard deviation of 1.20. Whether the pMDIs are only shaken, or sonicated and shaken, before use does not have a major impact on the size characteristics. Differences between the APS-measured geometric means for the shaken-only versus the sonicated and shaken were less than 0.1 μm for both BG and Bti.
TABLE 1 Size characteristics of test aerosols. The pMDI of Figure 1 was used to generate the aerosols
Neither the BG nor the Bti aerosols showed substantial quantities of large particles. The APS data were converted to volume size distributions and for neither spore type was there more than 1% of the aerosol volume-size distribution associated with sizes ≥3 μm AD.
Carrera et al. (Citation2007) measured sizes of BG and Bti spores as diameters (D) and lengths of the polar axes (L) of prolate spheroids. For BG, they reported D = 0.65 μm and L = 1.22 μm; and, for Bti, the values were D = 0.80 μm and L = 1.61 μm. Electron photomicrographs of BG and Bti spores, , qualitatively illustrate the approximately prolate spheroidal shapes of the spores. The aerodynamic diameters of particles having the size parameters reported by Carrera et al. can be estimated from a model presented by Hinds (Citation1999) together with additional information on spore density and a dynamic shape factor, where the latter takes into account the fluid resistance on a prolate spheroid as compared with a sphere. The spore density is approximately 1.4 g/mL (Carrera et al. Citation2008) and the shape factor for prolate spheroids aligned with the polar axes perpendicular to the flow is approximately 1.2 (Fuchs Citation1964). For these conditions, the calculated aerodynamic diameters of BG and Bti are 0.9 and 1.2 μm, respectively. That the APS-measured sizes are smaller than the predicted aerodynamic sizes may be due to non-Stokesian behavior of nonspherical particles in the APS (Cheng et al. Citation1993).
With respect to the size distributions of the residual aerosols produced by the nozzle-type and hub-type actuators, a comparative test using the APS procedure showed the number geometric mean diameter and geometric standard deviation from the nozzle-type actuator were 0.71 μm AD and 1.25, and the corresponding values from the hub-type actuator were 0.85 μm AD and 1.47. The pMDI used in this experiment contained Bti formulation. Because the spore sizes were the same in either case, the larger size and geometric standard deviation of the aerosol from the hub-type actuator was likely due a broader initial droplet size distribution, which produced a broader distribution of residual nonvolatile particles. This may be caused by differences in the atomization mechanics of a superheated two-phase HFA 134A jet that flows to the atmosphere directly from the 2 mm inside diameter stem as opposed to being restricted by a 0.5 mm diameter nozzle port before discharging to the atmosphere.
FIG. 4 (a) Shot weights from a pMDI containing propellant-only and having the configuration shown in Figure 1. Weights were determined after every actuation. (b) Shot weights from a pMDI containing BG formulation. The latter device was tested with three actuator configurations. Weight measurements were taken after 10 actuations.
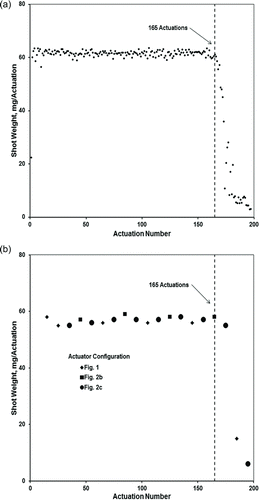
3.2. Shot Weight Constancy of pMDIs
The results of shot weight tests, in units of mg/actuation, for the complete pMDI () filled with propellant-only, are shown in . The change in PDC weight was measured after each actuation and the results show that after the first actuation, which would normally be discarded in the priming process, the shot weights were constant through the 165th actuation,
and then dropped. In the regime of constant shot weights covering the 3rd through the 165th actuations, the average shot weight ± one standard deviation was 61.6 ± 1.1 mg. Use of a statistical t-test indicates the slope of a regression line fitted to the experimental data is not significantly different than zero ( p = .57).
With reference to , the shot weights are shown for tests with the PDC filled with BG formulation, where three actuation configurations (, , and ) were used with the single PDC. PDC weights are plotted in at the midpoints of each set of 10 actuations. The first set of 10 actuations was considered to be priming and was discarded. Stable outputs were obtained for the region from 10 to approximately 165 actuations, above which there was a significant decline in shot weight. The average shot weight for the constant output regime was 56.9 ± 1.1 mg, The use of different actuation approaches showed little effect as the mass output of the pMDI fitted with the actuator arrangement of was 56.2 mg/actuation, that of was 58 mg/actuation, and that of was 56.7 mg/actuation. The statistical ANOVA does not reject a hypothesis that the mean values are equal (p = .09).
The metering valve controls the volume, not the weight, dispensed by a pMDI during an actuation. There was a slight difference in the densities of propellant-only (1,226 kg/m3) and propellant plus BG formulation (1,212 kg/m3); consequently, for the region of ≤165 actuations, the pMDI filled with propellant-only released an average volume of 50.2 ± 0.9 μL/actuation, and that filled with the BG formulation released an average volume of 46.9 ± 0.9 μL/actuation. Use of a statistical t-test at the 95% confidence level indicates the means of the two data sets are not equal (p < .001). The U.S. FDA (Citation1998) considered providing guidelines for pMDIs that included metering valve performance. The guidelines suggested shot weights of acceptable individual valves should be within 15% of the desired value. Assuming the desired volumetric output was 50 μL/actuation, the values of 50.2 and 47.0 μL/actuation would have been acceptable. Although those FDA guidelines were not implemented, they do provide an indication of desired performance of metering valves.
3.3. Constancy of Dose
pMDIs filled with Bti formulation and configured as shown in , were subjected to 200 actuations. Aerosol from two actuations from each group of 10 (the 4th and 5th actuations) were collected by the balloon method and analyzed for CFU. Results from the tests are shown in where Biodose Ratio, Rd ,bio, is presented as a function of actuation number. For actuation numbers ≤165, the overall mean ± 1 standard deviation are Biodose Ratios of 22% ± 5%.
FIG. 5 Biodose Ratio of Bti as a function of usage. Results show average ±1 standard deviation from tests with four replicate pMDIs at each actuation number.
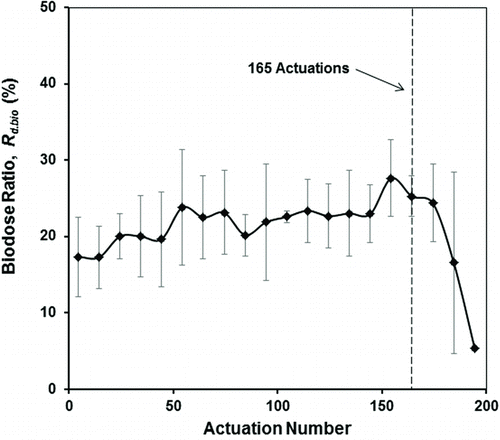
An ANOVA two-factor without statistical replication analysis was performed on the test results from the four pMDIs and 17 actuation sets per pMDI. Statistically, the hypothesis of equality of Biodose from the four different pMDI units was rejected (p < .001). However, differences in Biodose with actuation number, for ≤165 actuations, were not significant (p = .84). The Biodose Ratio and the CFUact are linearly related, as shown in EquationEquation (1), so the statistical conclusions regarding Biodose Ratio are directly translatable to conclusions for CFUact. Knowledge of the Biodose Ratio should be of value to users of the technology, who would seek to procure devices with dose levels that are compatible with functionality testing of their specific bioaerosol detection and identification systems.
3.4. Effect on Dose of Actuator Type and the Dose Variability Among Replicate Units
Results of tests with BG formulation to compare the dose output of the nozzle-type actuator () and the hub-type actuator (), are shown in . The flow through the stem of the pMDI was unobstructed when the hub actuator was used, whereas the flow through the nozzle-type actuator made a 90° turn and then discharged through the 0.50 mm diameter exit port. Four replicate PDCs were used for each actuator type, so a total of eight data sets were collected. In turn, each sample consisted of two actuations, with the first analyzed sample being the 4th actuation and the last analyzed sample being the 161st actuation. The experimental results in are the average doses over that range of actuations, which were produced by a given pMDI fitted with a particular nozzle. Statistical analysis, using three factor ANOVA without replication, did not reject the null hypothesis of equality dose from a given device with actuation numbers (p = .98); however, the null hypothesis of equal doses from the two types of actuators was rejected (p < .001) as was equality of doses from replicate pMDIs (p < .001).
Regarding the effect of actuator type, the four pMDIs fitted with nozzle-type actuators provided an overall average dose of 55.1 ± 23.3 CFU/actuation as compared with an overall average dose of 79.9 ± 14.3 CFU/actuation for the four pMDIs fitted with hub actuators. The ± values are one standard deviation about a mean. However, because the doses from both types of actuators are constant with usage, either actuation approach should be acceptable for functionality testing of bioaerosol detectors and identifiers.
FIG. 6 Dose of BG spores for two actuation approaches (nozzle-type of Figure 2b and hub type of Figure 2c) with four replicate PDCs for each actuator type. Data in each set represents the average covering the 4th through the 165th actuation. Lines at top of columns are +1 standard deviation error bars.
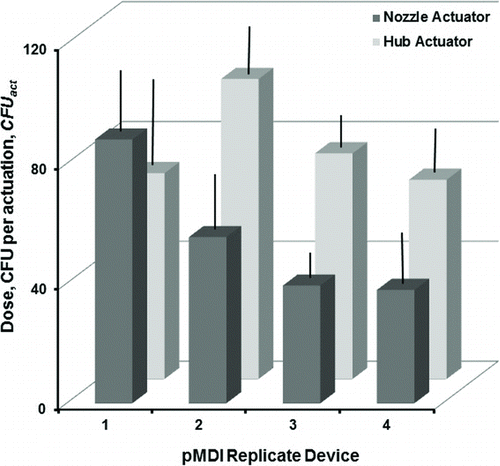
The differences in doses produced by replicate pMDIs filled with Bacillus spore formulations appear to be large compared with the US Pharmacopeia (USP Citation2012) requirement, which states that 10 units are to be tested and no dose may be outside the range of 65% to 135% of the target dose. If it were assumed the mean doses produced by the nozzle actuator and hub actuators were the target doses, then at least one of the data sets (Nozzle Actuator Replicate 1) would be outside the limit, which would cause the approach to fail the USP requirement. Because the canisters, valves, and stems used in this study are also used in the pharmaceutical industry, the differences between replicate units in this study are likely due, in part, to our use of biological particles. There may be enhanced adherence of spores on the internal walls as compared with adherence of pharmaceutical particle in acceptable units, and those spore losses could vary from unit-to-unit. However, even with nonbiological materials, there can be adherence to the inner walls of a PDC. A qualitative check on such losses was made by cutting open a PDC that had been filled with a formulation of 1 μm fluorescent polystyrene beads and stored for 9 months. Under ultraviolet light, fluorescence was observed on the inner wall of the canister, on the metering valve walls, and in the stem. Prior to opening the device, the dose was estimated by fluorescent analyses of samples collected with the balloon method. Only about 20% of the original fill material was output in the doses, which suggests that about 80% was lost to the walls of the device.
3.5. Effects of Storage Time and Temperature
on Bti Dose
The shelf life of spore-filled pMDIs should be known because ultimately with time, there will be a loss of usefulness. The results, in terms of CFUact associated with storage times of 1 to 12 months for BG and Bti formulations at 4°C and room temperature (24°C), are shown in . Trend lines (Excel 2010, Microsoft Corporation, Redmond, WA, USA) are also plotted.
FIG. 7 Effects of storage time and temperature on pMDI doses for BG and Bti. Regression lines are shown for each data set. Room temperature, RT, was 24°C.
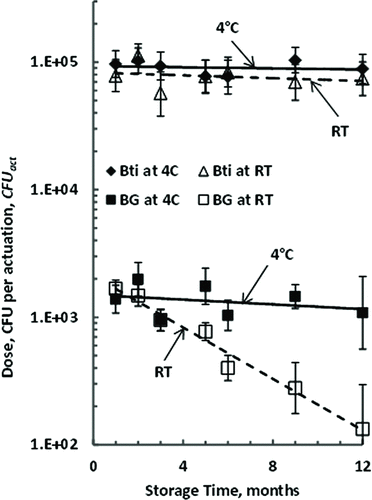
The trend lines are logarithmic regression lines fit to the experimental data, viz:
The values of CFUact,0 and the time constant, τ, which were calculated from the trend line parameters, are shown in .
TABLE 2 Calculated storage half-life of pMDIs using regression analysis for coefficients in EquationEquation (3)
The calculated half-life of the BG stored at room temperature is only 3.0 months, whereas the half-lives of the BG and Bti stored at 4°C are 33 and 136 months respectively. The half-life of Bti stored at room temperature is 20 months. The lower limits of the 95% confidence intervals of the half-lives can be calculated from analysis of the complete storage data set. Five replicates were used at each condition (storage time, temperature, and Bacillus spore species) except for a single replicate with BG stored at room temperature for 6 months, where the CFU measurement was 6 geometric standard deviations from the geometric mean. That data point was discarded because of apparent contamination. The lower limit of the 95% confidence interval for the half-lives of BG and Bti stored at room temperature and 4°C are shown in braces in the last column of . For BG and Bti stored at 4°C, the lower limits of the 95% confidence interval are ≥11 months.
There are significant differences between the Bti and BG spores that can explain their dissimilar survivability during long-term archiving. For example, the difference in hydrophobicity of their exosporium could result in increased hardiness for the strongly hydrophobic Bti, compared to the more hydrophilic BG (Greenberg et al. Citation2010). Also, the BG spores are smaller and lack the filaments that extend off exosporium in Bti, which may render the BG spores more sensitive. Phylogenetically, they are more distant relatives, demonstrated most importantly by the BclA gene, encoding a protein that is found in the Bti exosporium and helps determine the adhesive properties of the spore (Rety et al. Citation2005). As B. atrophaeus (BG) is lacking this gene, important changes can be expected in behavior compared to Bti. Although numerous articles are available regarding the characteristics of Bacillus anthracis, less is known about the simulant Bacillus strains and more work is needed to study and compare the spore survival of these species as also concluded by Greenberg et al. (Citation2010).
3.6. Demonstration of Functionality Testing of Bioaerosol Detectors
The demonstration of functionality testing involved use of a WWC to sample pMDI-generated aerosols and a RT-PCR to analyze the WWC collection liquid. Fifteen tests, with each test comprised of five actuations, were conducted with both BG and Bti. Positive responses were obtained for both BG and Bti, with the RT-PCR indicating count readings that corresponded to geometric mean doses of 522 CFUact for BG and 28,700 CFUact. for Bti. Simply for comparison, pMDI actuations were also analyzed with the balloon collection method and classical microbiological enumeration methodology. Five replicate tests, with five actuations for each test, were conducted in these experiments. The results showed geometric mean doses of 1,300 CFUact for BG and 95,100 CFUact for Bti.
The results of these tests show the pMDI-generated Bti aerosols can trigger a response in a bioaerosol detection system. However, the results additionally suggest the response of a bioidentifier to aerosol from a pMDI is not quantitative at this point in the development of pMDIs. This is illustrated by the ratio of the equivalent number of cells detected by the RT-PCR to the number of cells generated with the pMDI, that is, CFUact,RT-PCR/CFUact,Balloon, which was 0.4 for BG and 0.3 for Bti.
Researchers dealing with pharmaceutical aerosols have observed significant losses of dose in the mouth-throat area of the respiratory system, for example, Zhang et al. (Citation2007) reported 29% losses. In the present study, the pMDI aerosols were released into a 203 mm diameter × 2.1 m long tube placed upstream of the WWC, which was intended to provide assurance that the liquid phase of the spray droplets had evaporated and the aerosol jet from pMDI had dissipated so wall losses would be small in the WWC inlet.
4. RECOMMENDATION AND CONCLUSIONS
Tests were conducted with pMDIs containing Bti and BG spore formulations to examine their suitability for providing aerosols to check the functionality of bioaerosol detection and identification systems. Important considerations for use of pMDIs in this role are:
• | pMDIs should be at least shaken and then primed three actuations before use. | ||||
• | APS-measured particle size distributions created from aerosolization of BG and Bti formulations have geometric mean sizes of about 0.7–0.8 μm AD and geometric standard geometric standard deviation of about 1.3. | ||||
• | Less than 1% of the particle volume distribution is associated with particles ≥3 μm AD. | ||||
• | A PDC, which contains a fill volume that should provide 200 actuations, will have constant shot weights to 165 actuations. | ||||
• | Spore doses are constant with usage to 165 actuations. | ||||
• | Replicate pMDIs produce significantly different spore doses, which may be a result of differences in wall losses between units. | ||||
• | A Bespak spray nozzle actuator produces significantly lower doses than a hub-type actuator. However, as both actuators show constant doses for ≤165 actuations, either approach should be satisfactory. | ||||
• | PDCs should be stored under refrigeration (4°C), which should provide a half-life of a year or more. | ||||
• | In laboratory-type tests with BG and Bti aerosols, which were conducted to illustrate the utility of pMDIs in functionality testing, the aerosols were introduced into the collector (a 100 L/min WWC) via a transport tube. The latter was included to reduce the risk that large liquid droplets or high speed particles might impact on the walls of the WWC inlet. When a pMDI is used for functionality testing of fielded bioaerosol detection and identification systems, the potential for such losses should be considered. |
Acknowledgments
We wish to express our appreciation to Mr. Daniel Sanford of Texas A&M University, Dr. Erica Valdes of the U.S. Army Edgewood Chemical and Biological Center, and Mr. Joe Masci of Bespak Europe, Ltd., for assistance.
REFERENCES
- Byron , P. 1994 . Dosing Reproducibility from Experimental Albuterol Suspension metered-Dose Inhalers . Pharm. Res. , 11 ( 4 ) : 580 – 584 .
- Byron , P. 1997 . Performance Characteristics of Pressurized Metered Dose Inhalers in vitro . J. Aerosol Med. , 10 ( S ) : S3 – S6 . 1
- Carrera , M. , Kesavan , J. , Zandomeni , R. and Sagripanti , J.-L. 2005 . Method to Determine the Number of Bacterial Spores Within Aerosol Particles . Aerosol Sci. Technol. , 39 : 1 – 6. .
- Carrera , M. , Zandomeni , R. O. , Fitzgibbon , J. and Sagripanti , J.-L. 2007 . Difference Between the Spore Sizes of Bacillus Anthracis and Other Bacillus species . J. Appl. Microbiol. , 102 ( 2 ) : 313 – 312 .
- Carrera , M. , Zandomeni , R. O. and Sagripanti , J. L. 2008 . Wet and Dry Density of Bacillus Anthracis and Other Bacillus Species . J. Appl. Microbiol. , 105 : 68 – 77 .
- Cheng , Y. S. , Chen , B. T. , Yeh , H. C. , Marshall , I. A. , Mitchell , J. P. and Griffiths , W. D. 1993 . Behavior of Compact Nonspherical Particles in the TSI Aerodynamic Particle Sizer Model APS33B: Ultra-Stokesian Drag Forces . Aerosol Sci. and Technol. , 19 ( 3 ) : 255 – 267 .
- Cry , T. D. , Graham , S. J. , Li , R. and Lovering , E. G. 1991 . Low First-Spray Drug Content in Albuterol Metered-Dose Inhalers . Pharm. Res. , 8 ( 5 ) : 658 – 660 .
- Driks , A. 2003 . The Dynamic Spore . Proc. Natl. Acad. Sci. , 100 ( 6 ) : 3007 – 3009 .
- Everard , M. L. , Devadason , S. G. , Summers , Q. A. and Le Souef , P. N. 1995 . Factors Affecting Total and “Respirable” Dose Delivered by a Salbutamol Metered Dose Inhaler . Thorax , 50 : 746 – 749 .
- Fuchs , N. A. 1964 . The Mechanics of Aerosols , New York : The Macmillan Company .
- Graham , S. J. , Ormsby , E. D. and Lovering , E. G. 1992 . Single Spray Drug Content in a Metered-Dose Aerosol Formulation and a Collection Scheme for Content Uniformity . Pharmacopeial Forum , 16 ( 6 ) : 4400 – 4403 .
- Greenberg , D. L. , Busch , J. D. , Keim , P. and Wagner , D. M. 2010 . Identifying Experimental Surrogates for Bacillus Anthracis Spores: A Review . Invest. Genet. , 1 ( 1 ) : 1 – 12 .
- Harwood , C. and Cutting , S. , eds. 1990 . Molecular Biological Methods for Bacillus , Chichester , , UK : John Wiley & Sons .
- Hinds , W. C. 1999 . Aerosol Technology: Properties, Behavior, and Measurement of Airborne Particles, 2nd Ed. , New York : John Wiley & Sons .
- Hosni , A. , Szabo , J. and Bishop , P. 2011 . Efficacy of Chlorine Dioxide as a Disinfectant for Bacillus Spores in Drinking-Water Biofilms . J. Environ. Eng. , 137 ( 7 ) : 569 – 574 .
- June , D. , Carlson , S. and Ross , D. 1996 . “ The Effect of Temperature on Drug Delivery. Characteristics of Chlorofluorocarbon (CFC) and Hydrofluoroalkane (HFA) Metered Dose Inhalers (MDIs) ” . In Respiratory Drug Delivery V: Program and Proceedings , 354 – 356 . Buffalo Grove, IL : Interpharm Press .
- Kamiya , A. , Sakagami , M. , Hindle , M. and Byron , P. R. 2004 . Aerodynamic Sizing of Metered Dose Inhalers: An Evaluation of the Andersen and Next Generation Pharmaceutical Impactors and Their USP Methods . J. Pharm. Sci. , 93 ( 7 ) : 1828 – 1837 .
- King , M. D. and McFarland , A. R. 2012 . Bioaerosol Sampling with a Wetted Wall Cyclone: Cell Culturability and DNA Integrity of Escherichia coli Bacteria . Aerosol Sci. Technol. , 46 : 82 – 92 .
- Kreuzer-Martin , H. W. , Lott , M. J. , Dorigan , J. and Ehleringer , J. R. 2003 . Microbe Forensics: Oxygen and Hydrogen Stable Isotope Ratios in Bacillus Subtilis Cells and Spores . Proc. Natl. Acad. Sci. , 100 ( 3 ) : 815 – 819 .
- Madigan , M. T. and Martinko , J. M. , eds. 2006 . Brock Biology of Microorganisms, 11th ed. , Upper Saddle River, NJ : Prentice Hall .
- McFarland , A. R. , Haglund , J. S. , King , M. D. , Hu , S. , Phull , M. S. Moncla , B. W. 2010 . Wetted Wall Cyclones for Bioaerosol Sampling . Aerosol Sci. Technol. , 44 : 241 – 252 .
- Phillips , E. M. , Byron , P. R. , Fults , K. and Hickey , A. J. 1990 . Optimized Inhalation Aerosols. II. Inertial Testing Methods for Particle Size Analysis of Pressurized Inhalers . Pharm. Res. , 7 ( 12 ) : 1228 – 1233 .
- Rety , S. , Salamitou , S. , Garcia-Verdugo , I. , Hulmes , D. J. , Le Hegarat , F. Chaby , R. 2005 . The Crystal Structure of the Bacillus Anthracis Spore Surface Protein BclA Shows Remarkable Similarity to Mammalian Proteins . J. Biol. Chem. , 280 : 43073 – 43078 .
- Rubin , B. and Fink , J. 2005 . Optimizing Aerosol Delivery by Pressurized Metered-Dose Inhalers . Resp. Care , 50 ( 9 ) : 1191 – 1200 .
- Russell , A. D. 1990 . The Bacterial Spore and Chemical Sporicidal Agents . Cli. Microbiol. Rev. , 3 : 99 – 111 .
- Schumacher , J. and Lelner , S. 2012 . A Critical Evaluation of the Revised and New USP Chapters for Aerosols: <601> and <5> . Inhalation , : 6 – 11 . Available from: http://www.inhalationmag.com February, 2012
- Sneath , P. H. A. , Mair , N. S. , Sharpe , M. E. and Holt , J. G. , eds. 1986 . Bergey's Manual of Systematic Bacteriology , Vol. 2 , 1105 Baltimore, Maryland , , USA : Williams & Wilkins .
- Szabo , J. G. , Rice , E. W. and Bishop , P. L. 2007 . Persistence and Decontamination of Bacillus atrophaeus Subsp. globigii Spores on Corroded Iron in a Model Drinking Water System . Appl. Environ. Microbiol. , 73 : 2451 – 2457 .
- U.S. FDA. 1998 . Guidance for Industry. Metered Dose Inhaler (MDI) and Dry Powder Inhaler (DPI) Document distributed for comment purposes. Drug Information Branch (HFD-210), Center for Drug Evaluation and Research (CDER), Food and Drug Administration, U.S. Department of Health and Human Services. Rockville, MD. October 1998
- USP . 2012 . Aerosols, nasal sprays, metered-dose inhalers, and dry powder inhalers <601>. Informational Session at the 2012 Respirator Drug Delivery Meeting , Rockville, MD : U.S. Pharmacopeia .
- Vervaet , C. and Byron , P. 2000 . Polystyrene Microsphere Spray Standards Based on CFC-Free Inhaler Technology . J. Aerosol Med. , 13 ( 2 ) : 105 – 115 .
- Zhang , Y. , Gilbertson , K. and Finlay , W. H. 2007 . In vivo—in vitro Comparison of Deposition in Three Mouth-Throat Models with Qvar and Turbuhaler Inhalers . J. Aerosol Med. , 20 ( 3 ) : 227 – 235 .