Abstract
Size-resolved aerosol samples were collected both upwind and downwind of a large secondary road in the winter and spring of 2007 to assess contributions of on-road emissions to ambient aerosols. The aerosol samples were extracted and analyzed for a wide variety of organic compounds including polycyclic aromatic hydrocarbons (PAHs), alkanes, sugars, and organic acids. The results showed a strong seasonal pattern where the concentrations of most compounds were higher in winter than in spring. Some of the biogenic sugars were the exception, which might be the result of a “spring blooming season.” The surprising result was that the upwind site located in a residential neighborhood had very similar concentrations of most organic compounds compared to the near-roadway site. Possible reasons for the lack of differences in organic chemical concentrations between the near-road and control sites include: a large urban background concentration of aerosols superimposed on any local source; shifting wind directions that make the “downwind” site upwind during the night; and additional local sources in the residential neighborhood such as wood burning in winter.
Copyright 2014 American Association for Aerosol Research
INTRODUCTION
One of the common objectives of aerosol research is to protect human health by understanding both the composition and sources of particulate matter in the atmosphere. Numerous studies have reported the organic composition of aerosols with a focus on known toxic constituents such as polycyclic aromatic hydrocarbons (PAHs). The majority of these studies provide information on the total amount of organic compounds in the aerosol, but relatively few studies give chemical composition as a function of aerosol size fraction (Phuleria et al. Citation2006, Citation2007; Kleeman et al. Citation2008; Riddle et al. Citation2008; Eiguren-Fernandez and Miguel Citation2012; Yu and Yu Citation2012; Cahill Citation2013). However, the risks associated with aerosols are inherently dependent on both their size, which affects lung capture efficiencies (Heyder et al. Citation1986), and their composition, which affects the toxicity of the aerosol.
Size-resolved organic aerosol data also provide insight into the potential sources of the aerosols. Coarse aerosols tend to be associated with mechanical generation processes (e.g., dusts) while fine and ultrafine aerosols tend to have combustion sources or secondary aerosol formation processes. Organic aerosol size data are particularly useful when combined with tracer compounds from known sources since they suggest that the ambient aerosols were originally emitted from a particular source. This also shows whether the ambient aerosols have been modified (e.g., coagulated) as they undergo aging in the atmosphere, which may affect the bioavailability of the chemicals in the aerosols.
The objective of this research was to determine the organic constituents of size-resolved aerosols, with size ranges from <0.09 to 10 μm, at near-roadway and residential sites in a typical urban area. Size-resolved aerosol samples, including ultrafine aerosols, were collected during the winter and spring of 2007 in the Sacramento Metropolitan Area of California. The concentrations of PAHs, organic acids, sugars, and alkanes were determined in each size fraction, which provided both toxic exposure and tracer compounds to suggest sources of the aerosol.
MATERIALS AND METHODS
Ambient particulate matter was collected at two sites in Sacramento in 2007 to assess near-roadway concentrations of aerosols. The first site was on the roof of Arden Middle School approximately 5 m above ground level (). This site is 15 m away from Watt Avenue which had a daily traffic load of 65,000 vehicles per day (counted using Sacramento County traffic counting equipment) where 1.5% of the vehicles had five or more axles, which were predominantly heavy-duty trucks. The second site was located at ground level in a residential neighborhood 0.33 km west of Watt Avenue. This site was the control site for the near-road samplers for estimating the regional background of the aerosols in the Sacramento urban area.
FIG. 1 Picture showing the two sampling sites in relation to the major roadways. (Image from Google EarthTM.)
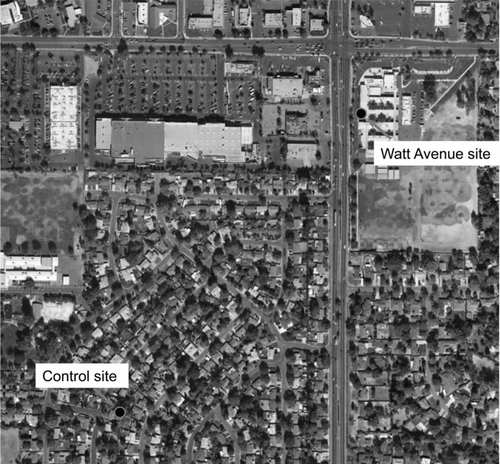
Samples were collected at four different time intervals. The first set was collected from 7 February to 7 March, which represented a winter-time weather pattern. The actual weather during sample collection consisted of a series of storms that promoted air mixing and ventilation. The other three sampling periods (19 March to 17 April, 17 April to 18 May, and 18 May to 23 June) represented more spring-like weather patterns with predictable diurnal up-slope/down-slope wind patterns and few storms.
The aerosols were collected using four 8-DRUM impactor samplers (Raabe et al. Citation1988) where two samplers were located at each site. At each site, one of the samplers used greased (Apiezon L grease, 5 ± 1 μg/cm2) mylar as a substrate to collect aerosols for elemental analysis (Cahill et al. 2007) while the other sampler used baked aluminum foil to collect particulate matter for organic analysis. For the first sampling period (7 February to 7 March), the aluminum was coated with a thin layer of Apiezon grease in the same fashion as the elemental samplers. The three subsequent sampling periods did not utilize a grease coating since it caused elevated blank concentrations of the n-alkanes. The lack of grease on the impaction substrate might cause more particle bounce, but there was little evidence for meaningful particle bounce based on the size profiles of coarse particle-associated organics, such as glucose, between the greased and nongreased substrates. The sampler divides the particulate matter into eight impactor-size fractions, namely 10 to 5.0, 5.0 to 2.5, 2.5 to 1.15, 1.15 to 0.75, 0.75 to 0.56, 0.56 to 0.34, 0.34 to 0.26, and 0.26 to 0.09 μm. A PTFE after-filter (Whatman 2 μm PTFE 46.2 mm filter) was placed after the smallest impaction stage to collect particulates with aerodynamic fractions less than 0.09 μm. A PTFE filter was chosen in preference to a quartz filter because of the lower reactivity of the PTFE filters compared to quartz filters (Watson et al. Citation2009). The filter did not contribute toward a significant background of any of the target analytes.
The samples destined for organic analysis were extracted and analyzed using the protocols utilized in other studies (Cahill Citation2010, Citation2013). The details are provided in the online supplemental information (SI). Briefly, the samples were placed in centrifuge vials and spiked with isotopically labeled internal standards to represent PAHs, alkanes, sugars, organic acids, and cholesterol. The amount of labeled compound added to the sample was 10 ng for the PAHs and 100 ng for the other chemicals. The samples were then extracted with 7 mL of toluene on a shaker to 20 h. The samples were centrifuged and the solution transferred to another centrifuge vial. Two more washes of the sample were conducted, each consisting of 7 mL toluene and 2 h on the shaker. The toluene extract was reduced by nitrogen evaporation to 2 mL. The remaining sample substrate and loose particulate matter were extracted with methanol in a similar fashion. The resulting methanol extract was then concentrated to 2 mL.
The determination of nonpolar analytes, such as PAHs and alkanes, was conducted using only the toluene extract. One milliliter of sample extract was passed through a silica gel clean-up column (0.25 g of 70–230 mesh gel) and eluted with 10 mL toluene. The extract was then reduced to 0.3 mL by nitrogen evaporation and then it was transferred to a GC vial insert and further reduced to 0.1 mL. A set of injection standards (10 ng for pyrene-d10 and 100 ng for other chemicals, see the SI for details) were then added to the sample to prepare it for instrumental analysis.
The determination of the polar analytes, namely organic acids and sugars, utilized both the toluene and methanol extracts. One milliliter of the methanol extract was reduced to 0.3 mL and transferred to a GC vial insert and evaporated to near-dryness. The remaining 1 mL of the toluene extract was reduced to 0.3 mL and added to the same GC vial insert. The mixture was then evaporated to dryness. Next, 100 μL of 50:50 mixture of BSTFA:pyridine was added to the vial and the vial was then heated at 45°C for 24 h to derivatize the hydroxyl and carboxylic acid functional groups. The injection standards were then added to the samples and the samples were immediately analyzed.
All samples were quantified using a Varian 3800 gas chromatograph coupled to a Saturn 2100 ion trap mass spectrometer. The chromatographic column was a 30 m Varian FactorFour high-temperature column (5% phenyl-substituted, 0.25 mm ID, 0.1 film thickness). The temperature program varied for the different classes of analytes, but the programs all shared the same structure, namely an initial temperature held for 5 min followed by a 5°C/min ramp to the final temperature where it was held for another 5 min. The initial temperatures for the polar, PAH, and native analyses were 40°C, 64°C, and 120°C, respectively, while the final temperature was 320°C, 380°C, and 320°C, respectively (SI). The ion trap mass spectrometer used electron ionization (EI) for all analytes except PAHs which utilized MS/MS methods.
Quantification was conducted by a six-point calibration curve. A relative response factor was calculated by dividing the peak area of each analyte by the peak area of the most structurally-similar internal standard. The minimum detectable limit was defined as the mean field blank value plus three standard deviations of the field blank or double the lowest detectable standard if the field blanks had no observable amounts of the analyte (Tables S1–S3).
TABLE 1 Spike-recovery values (expressed as % of original mass recovered) for all the field samples conducting a broad EI-MS scan (n = 90 samples)
RESULTS AND DISCUSSION
The recovery of the 16 internal standards added to all the field samples showed that the extraction and derivatization methodologies were generally effective with >70% recovery (). The exceptions were benzoic acid-d6 (61.9%), hexadecanoic acid-d31 (68.9%), and ribose-13C5 (40.6%) (). The substrates were largely free of contamination except for the first set that was deliberately greased to prevent particle bounce. These blanks and samples had an unacceptable background level of alkanes, so no alkane results are presented from these samples. The other chemical classes were unaffected by the grease.
FIG. 2 Chemical concentration as a function of particulate mass size for benzo[e]pyrene, levoglucosan, and glucose for both the winter and spring sampling times.
![FIG. 2 Chemical concentration as a function of particulate mass size for benzo[e]pyrene, levoglucosan, and glucose for both the winter and spring sampling times.](/cms/asset/625d80b5-85f8-41c9-8814-d813b281af62/uast_a_857757_o_f0002g.gif)
PAH Results
The PAH concentrations in the particulate matter showed several trends that suggest the most probable sources of these compounds. First, the concentrations of PAHs were much higher in winter at both sites compared to the spring samples. This trend implies that there was either a seasonal source or meteorological pattern, such as an inversion, that elevated the PAH concentrations. Second, the PAH concentrations at the “near-road” and the control site were indistinguishable in the three spring samples (pairwise t-test, p > 0.1). In winter, the control site had slightly higher PAH concentrations (pairwise t-test p = 0.017) than the near-road site (, ). This suggests that the roadway was not a significant source of PAHs compared to the regional concentrations of PAHs. This does not mean that the roadway was not a source of PAHs, but rather the local contribution of the roadway was low compared to the regional background. Third, the size distribution of the PAHs in all of the spring sample sets showed the highest concentrations of PAHs on the after filter, which shows that the PAHs were associated with ultrafine aerosols below 0.09 μm in diameter. In contrast, the winter samples had a large amount of the PAH mass in the 0.56 to 0.26 μm size range (). The different size distribution of the PAHs in winter implies either a different source of the PAHs or aerosol coagulation. It is worth noting that the different sizes of PAHs generally showed similar trends despite having potentially different sources.
TABLE 2 Concentration of PAHs (in pg/m3) in particulate matter at a control site in a residential neighborhood and at a site along Watt Ave., which is a busy secondary roadway
Organic Acids
The most abundant organic acids detected were hexadecanoic, octadecanoic, and 9-octadecenoic acids (AKA oleic acid) (). There was a seasonal difference with most of the organic acids having higher concentrations in the winter compared to the spring. This may have been the result of organic acids being emitted in wood smoke or low atmospheric ventilation rates that increased the concentrations of all aerosols. These fatty acids were found in all the aerosol size fractions (), which supports previous research that has shown that the long-chain alkanoic acids are emitted from a variety of different sources (Rogge et al. Citation1998, Citation1991; Schauer et al. Citation1993a,b, Citation2001; Hays et al. Citation2002). Once again, there was no pronounced difference between the near-roadway site and the residential control site.
TABLE 3 Concentrations of organic acids (ng/m3) in particulate matter at a control site in a residential neighborhood and at a site along Watt Ave., which is a busy secondary roadway
FIG. 3 Chemical concentration as a function of particulate mass size for nonanedioic acid, hexadecanoic acid, and n-C29 alkane for both the winter and spring sampling times.
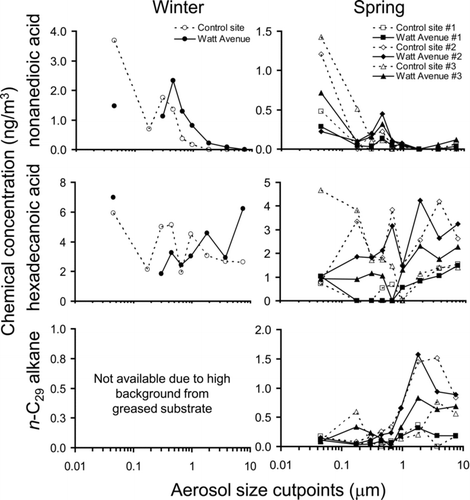
While most of the organic acids appeared to arise from a variety of sources, some organic acids were predominantly found in the finest size fractions that implies a combustion source. In particular, nonanedioic acid (), malic acid, and tartaric acid had most of the detected mass in particulates below 0.56 μm in size. These acids appeared in the same aerosol-size modes as levoglucosan in winter, but they were otherwise almost exclusively restricted to the ultrafine-size model in the spring. Their size distribution profile matches the PAH size profile, which implies a common source for both groups of chemicals.
Sugars and Related Compounds
The sugar results showed that levoglucosan, which is a well-known tracer of biomass burning (Simoneit et al. Citation1999; Nolte et al. Citation2001; Fine et al. Citation2004), showed the highest concentration of any quantified chemical during the winter sampling events, which agrees with previous studies of wintertime aerosols in the Central Valley of California () (Schauer and Cass Citation2000; Nolte et al. Citation2002; Rinehart et al. Citation2006; Chow et al. Citation2007; Kleeman et al. Citation2008). The size distribution for levoglucosan showed a maximum mass loading in the 0.26–0.56 μm size fractions (), which is in good agreement with previous research that has reported the ambient wood smoke aerosols in the 0.18–0.56 μm range (Kleeman et al. Citation2008). The size distribution of levoglucosan shows a similar pattern as the PAHs in winter (). The concentrations of levoglucosan were much lower in the spring samples, which were expected since there would be less wood burning during the warm spring months.
TABLE 4 Concentrations of sugars (and related compounds) and alkanes (ng/m3) in particulate matter at a control site in a residential neighborhood and at a site along Watt Ave., which is a busy secondary roadway
The concentrations of the sugars, such as glucose, showed the highest concentrations in the largest aerosol-size fractions (). These sugars were most likely the result of resuspended biomass such as detritus, spores, pollen, etc. Most of the sugars did not exhibit any significant seasonal differences in concentrations except for sucrose, which was not detected in winter but it was the most abundant such as during the springtime samples, which might be the result of a “spring bloom”(Fu et al. Citation2012). Sucrose was almost exclusively limited to aerosols larger than 0.75 μm with the maximum amount being found in the 5.0–10.0 μm size fraction, which was expected since particulate sugars are believed to be the result of course-suspended biomass (Jia and Fraser Citation2011). The concentration of the sugars showed no appreciable difference between the control site and the near-roadway site.
Alkanes
The last class of chemicals investigated was the alkanes
(). The alkanes were only quantified in the spring samples since the greased substrates used in the winter sampling events had a high background of alkanes. In the spring samples, the alkanes were mostly found in the larger aerosol-size fractions (), which suggests that they are arising from detritus, spore, and other biological material suspended in the atmosphere. The distribution of the alkanes followed a similar pattern as the sugars, although the alkanes have a maximum concentration between 1.15 and 5.0 μm. Once again, there was no appreciable influence of the roadway observed when comparing the concentrations of the alkanes from the near-road site and the residential control site.
The most abundant of the alkanes detected were the n-C29 and n-C31 alkanes. The odd-numbered carbon-length alkanes were more abundant than the even-numbered carbon-chain-length alkanes, which indicates a biogenic source of the alkanes. The carbon preference index (CPI), which is the sum of the odd-numbered carbon alkanes divided by the sum of the even-numbered carbon alkanes (Simoneit Citation1984; Mazurek et al. Citation1991), was 3.83 at the control site and 4.35 at the near-road site. Alkanes from a pure petroleum source would be expected to have a CPI of 1.0 while biological sources are expected to have values significantly greater than 1, and hence the values observed in this research would be indicative of a strong biological source for many of the alkanes.
DISCUSSION
The objective of this research was to assess the contribution of a large secondary roadway (65,000 vehicles/day) to aerosol concentrations adjacent to the roadway. The expectation was that the near-road site would have higher concentrations of chemicals associated with vehicle emissions compared to the residential control site located 0.33 km away from the road based on numerous road-side and tunnel sampling studies (Phuleria et al. Citation2006, Citation2007; Riddle et al. Citation2008; Eiguren-Fernandez and Miguel Citation2012). However, the results did not match the preconceived expectations in that there was no consistent pattern of higher concentrations of vehicle-related chemicals (e.g., PAHs) at the near-road site when compared to the control site. Additionally, the results from the elemental samples collected at the same time in the same fashion only showed a difference in ultrafine metals between the near-road and control site in winter (Cahill et al. 2007). The elemental analysis did not show differences between the sites in any of the spring sampling periods or for larger (>0.09 μm) aerosol sizes in winter. Therefore, the elemental and organic results are consistent in suggesting minimal differences between the near-road and residential sites.
There are several possible reasons why concentrations of organic compounds were generally similar between the near-road and control sites. The first reason was the relatively high regional background concentration of chemicals that obscures the influence of a local source. The sites were located in an urbanized area where the nearest undeveloped space was 6.4 km away and 12.8–14.4 km in most directions. Therefore, the air mass must pass over a large number of roads and urban development before reaching both the control and near-road sites. The article by Phuleria et al. (Citation2007) showed a similar trend in the Los Angles basin where the background aerosol concentrations are a significant portion of the near-freeway (I-710) concentrations for PAHs.
The second reason was that springtime meteorology was typically a regular up-slope/down-slope wind pattern that results in good ventilation at the site. The rapid air movement dilutes the local emissions and makes them harder to observe. Also, the daytime wind direction is typically “up-slope” into the Sierra Foothills during the day, which puts the school downwind off the roadway. At night, the air flow is “down-slope” and the school is upwind of the roadway. The organic samples collected in this study were integrated over the entire collection time, so the daily cycle in wind direction might dilute the influence of the roadway.
The wintertime meteorology, with frequent stagnation events and inversions, would be more favorable to detect the local roadway source. However, the wintertime organic samples were dominated by wood smoke that simply swamped any local influence of the roadway. The control site in the residential neighborhood had higher concentrations of wood smoke and hence it had slightly higher concentrations of many of the PAHs. Determining wood smoke contribution to the local aerosols was not the objective of this project, but this result does highlight the regulatory need to control wood smoke.
The main conclusion from this research is that the difference in aerosol concentrations between residential and near-road sites may not be as high as expected. High concentrations of regional aerosols may obscure local emission sources such as a single roadway. Furthermore, residential areas may have local aerosol sources (e.g., wood smoke, cooking, and gas-powered yard equipment) that may elevate aerosol concentrations in residential areas. These results suggest that more detailed aerosol studies need to be focused in residential areas rather than near expected sources.
Supplemental_Information.zip
Download Zip (116 KB)Acknowledgments
[Supplementary materials are available for this article. Go to the publisher's online edition of Aerosol Science and Technology to view the free supplementary files.]
We would like to thank the Sacramento Metropolitan Air Quality Management District for supporting this research; the staff of the Arden Middle School for their help setting up and maintaining the Watt Avenue site; and the Harding family for access to their backyard for the residential sampling site.
REFERENCES
- Cahill , T. A. , Cahill , T. M. , Spada , N. J. and Barnes , D. E. 2007 . Organic and Elemental Aerosols near Watt Avenue, Late Winter-Spring, 2007. Final Report to the Sacremento Metropolitan Air Quality Management District, Sacremento, CA .
- Cahill , T. M. 2010 . Size-Resolved Organic Speciation of Wintertime Aerosols in California's Central Valley . Environ. Sci. Technol. , 44 : 2315 – 2321 .
- Cahill , T. M. 2013 . Annual cycle of size-resolved organic aerosol characterization in an urbanized desert environment . Atmos. Environ. , 71 : 226 – 233 .
- Chow , J. C. , Watson , J. G. , Lowenthal , D. H. , Chen , L. W. A. , Zielinska , B. Mazzoleni , L. R. 2007 . Evaluation of Organic Markers for Chemical Mass Balance Source Apportionment at the Fresno Supersite . Atmos. Chem. Phys. , 7 : 1741 – 1754 .
- Eiguren-Fernandez , A. and Miguel , A. H. 2012 . Size-Resolved Polycyclic Aromatic Hydrocarbon Emission Factors from On-Road Gasoline and Diesel Vehicles: Temperature Effect on the Nuclei-Mode . Environ. Sci. Technol. , 46 : 2607 – 2615 .
- Fine , P. M. , Cass , G. R. and Simoneit , B. R. T. 2004 . Chemical Characterization of Fine Particle Emissions from the Fireplace Combustion of Wood Types Grown in the Midwestern and Western United States . Environ. Eng. Sci. , 21 : 387 – 409 .
- Fu , P. , Kawamura , K. , Kobayashi , M. and Simoneit , B. R. T. 2012 . Seasonal Variations of Sugars in Atmospheric Particulate Matter from Gosan, Jeju Island: Significant Contributions of Airborne Pollen and Asian Dust in Spring . Atmos. Environ. , 55 : 234 – 239 .
- Hays , M. D. , Geron , C. D. , Linna , K. J. , Smith , N. D. and Schauer , J. J. 2002 . Speciation of Gas-Phase and Fine Particle Emissions from Burning of Foliar Fuels . Environ. Sci. Technol. , 36 : 2281 – 2295 .
- Heyder , J. , Gebhart , J. , Rudolf , G. , Schiller , C. F. and Stahlhofen , W. 1986 . Deposition of Particles in the Human Respiratory Tract in the Size Range of 0.005–15 μm . J. Aerosol Sci. , 17 : 811 – 825 .
- Jia , Y. and Fraser , M. 2011 . Characterization of Saccharides in Size-Fractionated Ambient Particulate Matter and Aerosol Sources: The Contribution of Primary Biological Aerosol Particles (PBAPs) and Soil to Ambient Particulate Matter . Environ. Sci. Technol. , 45 : 930 – 936 .
- Kleeman , M. J. , Riddle , S. G. and Jakober , C. A. 2008 . Size Distribution of Particle-Phase Molecular Markers During a Severe Winter Pollution Episode . Environ. Sci. Technol. , 42 : 6469 – 6475 .
- Mazurek , M. A. , Cass , G. R. and Simoneit , B. R. T. 1991 . Biological Input to Visibility-Reducing Aerosol-Particles in the Remote Arid Southwestern United-States . Environ. Sci. Technol. , 25 : 684 – 694 .
- Nolte , C. G. , Schauer , J. J. , Cass , G. R. and Simoneit , B. R. T. 2001 . Highly Polar Organic Compounds Present in Wood Smoke and in the Ambient Atmosphere . Environ. Sci. Technol. , 35 : 1912 – 1919 .
- Nolte , C. G. , Schauer , J. J. , Cass , G. R. and Simoneit , B. R. T. 2002 . Trimethylsilyl Derivatives of Organic Compounds in Source Samples and in Atmospheric Fine Particulate Matter . Environ. Sci. Technol. , 36 : 4273 – 4281 .
- Phuleria , H. C. , Geller , M. D. , Fine , P. M. and Sioutas , C. 2006 . Size-Resolved Emissions of Organic Tracers from Light-and Heavy-Duty Vehicles Measured in a California Roadway Tunnel . Environ. Sci. Technol. , 40 : 4109 – 4118 .
- Phuleria , H. C. , Sheesley , R. J. , Schauer , J. J. , Fine , P. M. and Sioutas , C. 2007 . Roadside Measurements of Size-Segregated Particulate Organic Compounds Near Gasoline and Diesel-Dominated Freeways in Los Angeles, CA . Atmos. Environ. , 41 : 4653 – 4671 .
- Raabe , O. G. , Braaten , D. A. , Axelbaum , R. L. , Teague , S. V. and Cahill , T. A. 1988 . Calibration Studies of the Drum Impactor . J. Aerosol Sci. , 19 : 183 – 195 .
- Riddle , S. G. , Robert , M. A. , Jakober , C. A. , Hannigan , M. P. and Kleeman , M. J. 2008 . Size-Resolved Source Apportionment of Airborne Particle Mass in a Roadside Environment . Environ. Sci. Technol. , 42 : 6580 – 6586 .
- Rinehart , L. R. , Fujita , E. M. , Chow , J. C. , Magliano , K. and Zielinska , B. 2006 . Spatial Distribution of PM2.5 Associated Organic Compounds in Central California . Atmos. Environ. , 40 : 290 – 303 .
- Rogge , W. F. , Hildemann , L. M. , Mazurek , M. A. , Cass , G. R. and Simoneit , B. R. T. 1991 . Sources of Fine Organic Aerosol .1. Charbroilers and Meat Cooking Operations . Environ. Sci. Technol. , 25 : 1112 – 1125 .
- Rogge , W. F. , Hildemann , L. M. , Mazurek , M. A. , Cass , G. R. and Simoneit , B. R. T. 1993a . Sources of Fine Organic Aerosol .2. Noncatalyst and Catalyst-Equipped Automobiles and Heavy-Duty Diesel Trucks . Environ. Sci. Technol. , 27 : 636 – 651 .
- Rogge , W. F. , Hildemann , L. M. , Mazurek , M. A. , Cass , G. R. and Simoneit , B. R. T. 1993b . Sources of Fine Organic Aerosol .4. Particulate Abrasion Products from Leaf Surfaces of Urban Plants . Environ. Sci. Technol. , 27 : 2700 – 2711 .
- Rogge , W. F. , Hildemann , L. M. , Mazurek , M. A. , Cass , G. R. and Simoneit , B. R. T. 1998 . Sources of Fine Organic Aerosol. 9. Pine, Oak and Synthetic Log Combustion in Residential Fireplaces . Environ. Sci. Technol. , 32 : 13 – 22 .
- Schauer , J. J. and Cass , G. R. 2000 . Source Apportionment of Wintertime Gas-Phase and Particle-Phase Air Pollutants Using Organic Compounds as Tracers . Environ. Sci. Technol. , 34 : 1821 – 1832 .
- Schauer , J. J. , Kleeman , M. J. , Cass , G. R. and Simoneit , B. R. T. 2001 . Measurement of Emissions from Air Pollution Sources. 3. C-1-C-29 Organic Compounds from Fireplace Combustion of Wood . Environ. Sci. Technol. , 35 : 1716 – 1728 .
- Simoneit , B. R. T. 1984 . Organic-Matter of the Troposphere .3. Characterization and Sources of Petroleum and Pyrogenic Residues in Aerosols over the Western United-States . Atmos. Environ. , 18 : 51 – 67 .
- Simoneit , B. R. T. , Schauer , J. J. , Nolte , C. G. , Oros , D. R. , Elias , V. O. Fraser , M. P. 1999 . Levoglucosan, a Tracer for Cellulose in Biomass Burning and Atmospheric Particles . Atmos. Environ. , 33 : 173 – 182 .
- Watson , J. G. , Chow , J. C. , Chen , L. W. A. and Frank , N. H. 2009 . Methods to Assess Carbonaceous Aerosol Sampling Artifacts for IMPROVE and Other Long-Term Networks . J. Air & Waste Manage. Assoc. , 59 : 898 – 911 .
- Yu , H. and Yu , J. Z. 2012 . Polycyclic Aromatic Hydrocarbons in Urban Atmosphere of Guangzhou, China: Size Distribution Characteristics and Size-Resolved Gas–Particle Partitioning . Atmos. Environ. , 54 : 194 – 200 .