Abstract
An existing differential mobility analyzer (DMA) of cylindrical electrodes and a novel DMA of rectangular plate electrodes are demonstrated for size fractionation of nanoparticles at high-aerosol flow rates in this work. The two DMAs are capable of delivering monodisperse size selected nanoparticles (SMPS σg < 1.1) at gas flow rates ranging from 200 slm to 500 slm. At an aerosol flow rate of 200 slm, the maximum attainable particle mean size is of about 20 nm for the cylindrical DMA and of nearly 50 nm for the rectangular plate DMA. The number concentration of the monodisperse nanoparticles delivered by the high-flow DMAs spans from 104 cm−3 to 106 cm−3 depending upon the particle mean size and particle size dispersion.
Copyright 2014 American Association for Aerosol Research
INTRODUCTION
The preparation of nanoparticles with uniform size, shape, and composition is intensively pursued because of their scientific and technological interests. As an example, high-purity size monodisperse metal nanoparticles are well suited for applications in a variety of areas such as catalysis, photonics, ultrahigh density magnetic storage, biomedicine, magnetic resonance imaging, medical diagnostics, and drug delivery (Peng Citation2009). Also, the availability of nanoparticle standards is of utmost importance for questions related to nanoparticle sizing or tracing and are commonly used for giving the basis for evaluation and standardization methods, technologies, and applications (Cook and Kaiser Citation2011). Size monodisperse nanoparticles are currently produced by synthetic routes or through selective separation methods in very small quantities in the laboratory and, therefore, there is a need to scale up such methods for high-yield production of nanoparticles of well-defined properties (Masala and Seshadri Citation2004; Cui et al. Citation2009).
This work is aimed at enhancing the production of monodisperse nanoparticles in the gas phase by means of differential mobility analyzer (DMA). Two flows take part in the DMA: the polydisperse aerosol flow, from which a fraction of particles within a narrow size range is separated and, then, extracted from the DMA; and a clean particle-free gas flow (sheath), which carries away the remaining nonselected aerosol particles. The maximum attainable aerosol and sheath flow rates are limited by the dimensions of the DMA, which must be easy to manufacture and assembly and affordable. The feasibility of a cylindrical DMA (high-flow DMA, HF-DMA) for increasing the yield of monodisperse nanoparticles was demonstrated in a previous work (Hontañón and Kruis Citation2009). Then, monodisperse nanoparticles in the size range of 5–20 nm and number concentrations in the order of 104 cm − 3 were generated at a gas flow rate of 90 slm. The goal of this work is to develop a DMA capable to supply monodisperse nanoparticles of up to 50 nm in size with number concentrations larger than 104 cm − 3 at a gas flow rate of 200 slm. The experience gained with the HF-DMA discouraged us from further scaling up the cylindrical DMA to cope with aerosol flow rates above 100 slm. On one side, the high-precision manufacturing of large cylindrical tubes is quite costly. On the other side, the assembly of cylindrical DMAs of large dimensions is troublesome; in particular, the alignment of the electrodes requires the development of dedicated tools and the assistance of skilled personnel. Then, the planar geometry comes into consideration. Several prototypes of planar DMAs both with circular disks (radial DMA) and rectangular plates as electrodes have been developed, which operate at aerosol flow rates of a few liters per minute. Planar DMAs are used in commercial equipment, like the NanoScan SMPS (Model 39010, TSI Inc., USA) based on a radial DMA (Zhang et al. Citation1995) and the IONER High Resolution IMS (RAMEM S.A., Spain) based on a DMA of rectangular plates (Santos et al. Citation2009). The rectangular plate is the preferred choice for this work since the manufacture of plates of 1 × 1 m2 is easier and cheaper than disks of about 0.3 m in diameter having a similar perimeter; also plates are easier to handle than disks.
A novel DMA of parallel rectangular plates is presented here that was conceived to supply monodisperse size selected nanoparticles at a gas flow rate of 200 slm (hereinafter referred to as rectangular plate HF-DMA). The performance of the new rectangular plate HF-DMA is assessed and compared to the cylindrical HF-DMA for aerosol flow rates between 200 slm and 500 slm. To that aim, particle size fractionation experiments are conducted with the two HF-DMAs in a dedicated facility. Polydisperse aerosols, with adjustable particle size distribution and particle number concentrations well above 106 cm − 3, are generated at high gas flow rates by means of electrical discharge between two electrodes. The particles supplied by the cylindrical and the rectangular plate HF-DMAs reach the target in size dispersion (SMPS geometric standard deviation σg < 1.1) and particle throughput (SMPS particle number concentration N > 104 cm − 3).
HIGH-FLOW DMAs
The geometry and construction features of the cylindrical HF-DMA are reported in a previous work (Hontañón and Kruis Citation2009), whereas the new rectangular plate HF-DMA is described in this section. An intensive flow simulation study was accomplished by means of Cosmoflow (CFD module of the SolidWorks 3D CAD software) to support the design of the rectangular plate HF-DMA. The study was aimed at addressing key flow issues affecting the performance of the DMA. The classification zone of the rectangular plate DMA is a rectangular channel which top and bottom walls are the metallic electrodes, while the lateral walls are made of an electrical insulator (PTFE). The aerosol enters and exits the classification zone through rectangular slits carved in the electrodes. The flow and the electric field in the vicinity of the lateral walls are not uniform and, thus, the aerosol particles must not enter the region nearby the walls. The CFD simulations allowed determining a safe distance of 50 mm from the walls, so that the length of the aerosols slits was set to 450 mm (the width of the classification zone is 550 mm). The mixing of the aerosol and the sheath flows at the entrance of the classification zone was simulated also. It was found that 3.5 mm is an optimal width of the aerosol inlet slit, resulting in stationary laminar mixing of the two flows in the simulations. To achieve a homogeneous distribution of the flow within the classification region of the rectangular plate HF-DMA is a major engineering challenge. The sheath and the aerosol enter the DMA first through cylindrical DN100 (Øi 100 mm) and DN63 (Øi 70 mm) pipes and flow into the classification zone through rectangular cross sections of 550 × 25 mm2 and 450 × 3.5 mm2, respectively. Numerous engineering solutions were attempted in the simulations in order to uniformly distribute the sheath gas and aerosol flows over the rectangular openings.
shows a layout of the rectangular plate HF-DMA. The central body includes the classification zone together with a contraction and an expansion. The cylindrical tubes located, respectively, upstream and downstream of the contraction and the expansion are the sheath flow distributors. The smaller cylindrical tubes placed above and underneath the central body are the aerosol flow distributors. A three-dimensional drawing of the inlet distributor of the sheath gas is displayed in . It consists of two concentric cylindrical tubes. The inner tube through which the sheath enters the DMA shows circumferential slits along the entire tube length. The diameter of the outer tube is twice the diameter of the inner tube and has a single rectangular longitudinal opening. The slitted inner tube breaks the round jet flow of the sheath which then enters the annular space between the two tubes and flows finally toward the classification zone through the rectangular opening of the outer tube. The number and the width of the slits of the inner tube and the dimensions of the rectangular slot of the outer tube were optimized in order to attain a unidirectional uniform flow within the classification zone, as shown in . The outlet distributor of the sheath is similar to the inlet distributor previously described except for the number and the width of the slits. As a means of further damping the turbulence of the flow in the free volume between the tubes, it was decided to fill this with round hollow HEPA filters placed around the inner tubes of both the inlet and the outlet sheath distributors. The filters are also aimed at cleaning the sheath gas. The aerosol distributors rely on the same concept as the sheath distributors but the tubes have smaller diameters and do not include filters.
FIG. 1 The high-flow DMA of rectangular plate electrodes: (a) general layout, (b) sheath flow distributor, and (c) streamlines of the sheath flow within the distributor and the classification zone.
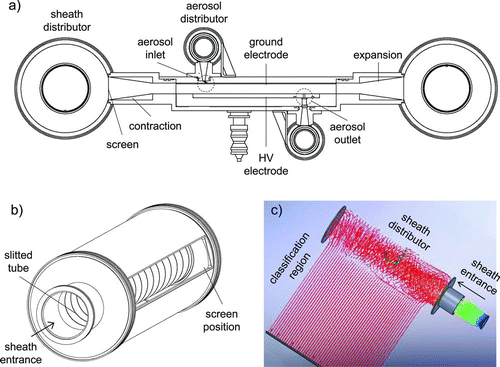
The transfer function of the cylindrical HF-DMA has been thoroughly discussed in a previous paper (Hontañón and Kruis Citation2009). The transfer function of the DMA of rectangular plates was numerically obtained and measured for a DMA that is part of a commercial ion mobility spectrometer (Santos et al. Citation2009). Ideally, the resolution of a DMA of any geometry is given by the sheath to aerosol flow ratio Q/q, where Q is the sheath flow rate and q is the aerosol flow rate (Knutson and Whitby 1975). For small nanoparticles, the diffusion broadening of the transfer function is proportional to Pe −1/2, where Pe is the particle Peclet number. This is the product of the flow Reynolds number and the particle Schmidt number, Pe = ReSc, and Sc is the ratio of the gas kinematic viscosity to the particle diffusion coefficient, Sc = νg/Dp . Therefore, the resolution of the DMA for highly diffusive nanoparticles can be improved by increasing the flow Reynolds number. At the nominal flow rates, the Reynolds number of the flow in the cylindrical and rectangular plate HF-DMAs is of about 2000 and 4400, respectively. Then, the Peclet number of particles of 3 nm reaches values of 5 × 104 and 105 for which diffusion broadening is not an issue (Rosell-Llompart et al. Citation1996).
Screens are used in DMAs to obtain a uniform velocity distribution of the mean flow and to reduce the turbulence of the sheath flow before it enters the classification zone. In general, the performance of a screen depends upon the flow pressure drop which in turn is a function of the screen porosity (ratio of open area to total area) and the Reynolds number based on the flow velocity through the pores and the diameter of the wire, ; where Q is the gas flow rate; A, α, and d are the total surface area, porosity, and wire diameter of the screen, respectively; and νg
is the gas kinematic viscosity. As a rule of thumb, when the Reynolds number Red
is less than 40, screens simply damp out flow fluctuations and turbulence is reduced (Groth and Johansson Citation1988). When Red
is larger than 40, however, screens induce additional small-scale turbulence into the flow (Laws and Livesey Citation1978; Oshinowo and Kuhn Citation2000). For any value of the sheath flow rate Q, there is a set of values of the screen parameters (d, α) that satisfy the condition Red
<40. Then, several polymeric screens were purchased and tested. The test consists in passing the aerosol flow through the DMA and progressively increasing the sheath flow rate at the same time that the number concentration of particles in the gas leaving the DMA is monitored by means of a CPC. When the flow within the DMA is laminar, the particle number concentration in the gas at the outlet is zero. In practice, for any aerosol flow rate q there is an optimal range of values of the sheath flow rate Q for which the flow in the DMA is laminar. The screens that showed the best performance in these tests were used later on in the experiments on particle size fractionation with the high-flow DMAs.
Values of the geometrical and flow parameters most relevant to particle size classification and the main features of the laminarization screens both of the cylindrical and the rectangular plate HF-DMAs are provided in the online supplementary information (SI) (high flow-DMAs). The performance with regard to particle size fractionation of the HF-DMAs is assessed in a large facility aimed at producing at high-yield monodisperse nanoparticles by means of DMA. The description and a sketch of the facility can be seen in the SI (Experimental Facility section).
RESULTS
Polydisperse aerosols are generated by electrical discharges between two electrodes of copper in nitrogen at atmospheric pressure in this work (Hontañón et al. Citation2013). The aerosol particle size distribution is tuned up by varying the current applied to the electrodes and the gas flow rate, and is measured by an SMPS system (TSI 3080) including a NanoDMA (TSI 3085) and a CPC (TSI 3775). Values of the geometric mean diameter dg , the geometric standard deviation σg , and the particle number concentration N of the copper aerosols are listed in . The copper aerosols generated by electrical discharges are bipolarly charged. Here, only positively charged particles are classified by applying an appropriate voltage. At given aerosol and sheath flow rates, the voltage on the DMA is scanned by using a high voltage power supply and, for any applied voltage, the size distribution of the particles selected by the DMA is measured with the SMPS.
TABLE 1 Parameters of the particle size distribution (SMPS) of the polydisperse aerosol source
First, experiments are conducted with the HF-DMA operating at aerosol and sheath flow rates of 200 slm and 400 slm, respectively. The size distributions of the particles selected by the cylindrical HF-DMA are plotted in ; the curves correspond to different values of the applied voltage. The geometric mean diameter dg of the particle size distribution varies between 5 nm and 17 nm. In general, the lower and the upper detectable particle sizes depend on the particle size distribution of the polydisperse aerosol. In addition, the lower particle size depends on the losses of small highly diffusive particles within the DMA, whereas the upper particle size is determined by the maximum attainable voltage. The geometric standard deviation σg is of roughly 1.04 regardless of the particle size, and the particle number concentration N lies between 1.4 × 104 cm − 3 and 6.2 × 105 cm − 3. Then, experiments are performed at higher aerosol flow rates keeping a sheath to aerosol flow ratio of 2. The results correspondent to aerosol flow rates of 350 slm and 500 slm are displayed in . In the two cases, dg ranges from 4 nm to 15 nm and the values of N are comparable to the ones obtained at an aerosol flow rate of 200 slm (). As for the experiment at 200 slm, σg is independent of the particle size but the values are larger; 1.06 and 1.08 in the experiments at 350 slm and 500 slm, respectively. A further experiment is carried out at an aerosol flow rate of 500 slm and a sheath to aerosol flow ratio of 3. The particle size distributions of the aerosols selected with the cylindrical HF-DMA in this experiment are depicted in . A value of σg of 1.065 is attained, which is lower than the value of 1.08 obtained with the same aerosol flow rate and a sheath to aerosol flow ratio of 2. The decrease in σg is accompanied by a reduction of the particle number concentration of the monodisperse aerosol as, for example, the concentration of particles of mean sizes 8 nm and 14 nm diminishes from 4.5 × 105 cm − 3 to 3.0 × 105 cm − 3 (33.3%) and from 4.4 × 104 cm − 3 to 3.0 × 104 cm−3 (32%), respectively. Also, show that σg increases with increasing aerosol flow rate when the cylindrical HF-DMA operates at a constant sheath to aerosol flow ratio of 2, indicating that σg is not solely determined by the ratio Q/q but depends also upon q. We attribute this to flow unsteadiness, degrading the resolution of the cylindrical HF-DMA with increasing aerosol flow rate. At a fixed aerosol flow rate, the resolution of the DMA enhances with increasing sheath gas flow rate. Lower values of σg are then attained, but the particle number concentration N diminishes too. With the polydisperse aerosol source used in his work, the concentration of monodisperse particles supplied by the cylindrical HF-DMA falls down below the target value of 104 cm−3 at sheath flow rates above 1500 slm and, thus, the results are not shown here.
FIG. 2 Size distributions of copper particles classified by the cylindrical HF-DMA at the indicated aerosol flow rates q and sheath gas flow rates Q. The geometrical standard deviation σg and the minimum and maximum values of the particle number concentration N are shown.
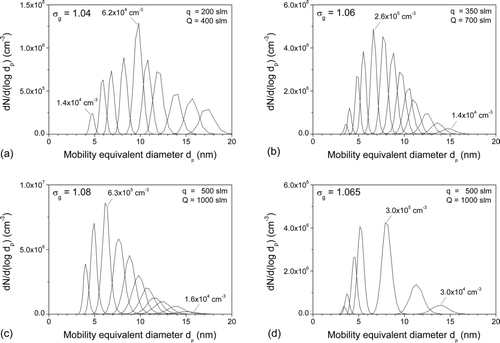
FIG. 3 Size distributions of copper particles classified by the rectangular HF-DMA of rectangular plates at the indicated aerosol flow rates q and sheath gas flow rates Q. The geometrical standard deviation σg and the minimum and maximum values of the particle number concentration N are shown.
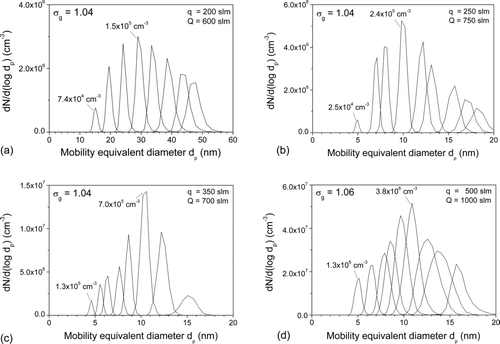
Next, experiments on particle size fractionation are carried out with the rectangular plate HF-DMA. The particle size distributions of the selected aerosols are displayed in . They correspond to aerosol flow rates of 200 slm and 250 slm with a sheath to aerosol flow ratio of 3, and to aerosol flow rates of 350 slm and 500 slm with a sheath to aerosol flow ratio of 2. A value of σg of 1.04 is obtained in all the cases except for the experiment at an aerosol flow rate of 500 slm and a sheath to aerosol flow rate of 2, with a σg of 1.08. Also, higher number concentrations of monodisperse particles are attained at an aerosol flow rate of 500 slm. This result, however, cannot be attributed to the increase in σg , as explained by comparison of for the cylindrical HF-DMA operating at an aerosol flow rate of 500 slm and sheath flow rates of 1000 slm and 1500 slm. display the particle size distributions of monodisperse aerosols selected by the rectangular plate HF-DMA operating at different aerosol flow rates and, hence, with different aerosol sources (). The comparison in terms of particle number concentration of the monodisperse aerosols shown in is, therefore, misleading.
It can be concluded from the comparison of and that the two HF-DMAs perform similarly with respect to particle size fractionation. It is worthy to mention, however, the larger particle sizes attainable with the rectangular plate DMA. As can be seen in and , the cylindrical and the rectangular plate HF-DMAs supply particles of dg up to 17 nm and 48 nm, respectively, with a σg of 1.04 at a flow rate of 200 slm. On one side, the maximum attainable particle size increases with decreasing sheath flow rate. On the other side, at a given sheath flow rate, the highest particle size susceptible to be classified with the DMA increases both with the length of the classification column and the maximum attainable voltage. The rectangular plate HF-DMA has a classification column of 230 mm in length, while the length of the column of the cylindrical HF-DMA is of 100 mm. In the experiments, the maximum attainable voltage is of 15 kV in the cylindrical HF-DMA and 20 kV in the rectangular plate HF-DMA. So far monodisperse nanoparticles of sizes less than 20 nm have been produced at flow rates ranging from 90 slm to 500 slm with the cylindrical HF-DMA. In this work, monodisperse particles of up to 61 nm and 72 nm are produced at a flow rate of 100 slm with the rectangular plate DMA, as shown in . Furthermore, at given aerosol and sheath flow rates, the rectangular plate HF-DMA leads to larger concentrations of monodisperse particles. This is concluded from the comparison of the aerosols supplied by the two HF-DMAs operating at aerosol and sheath flow rates of 350 slm and 750 slm, respectively; higher number concentrations are attained with the rectangular plate HF-DMA despite of the narrower particle size distribution (, σg = 1.04), with respect to the cylindrical HF-DMA (, σg = 1.06). Also, in and , the aerosols delivered by the HF-DMAs operating at aerosol and sheath flow rates of 500 slm and 1000 slm show the same σg (1.08), while larger particle number concentrations are reached with the rectangular plate HF-DMA. We attribute these results to lower particle losses in the rectangular plate HF-DMA. The planar geometry makes it possible to reduce the residence time of the aerosol in the inlet and outlet aerosol passages of the DMA, with respect to the cylindrical DMA (Santos et al. Citation2009).
FIG. 4 Size distributions of copper particles classified by the rectangular plate DMA of rectangular plates at an aerosol flow rate q of 100 lpm and sheath gas flow rates Q of 300 lpm and 400 lpm. The geometrical standard deviation σg and the maximum attainable values of the geometric diameter dg are shown.
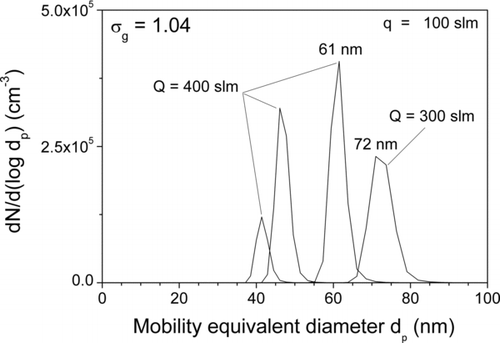
DISCUSSION
The high monodispersity (SMPS σg ≤ 1.08) of the aerosols delivered by the two HF-DMAs in the experiments is a surprising result. In the original work on the cylindrical HF-DMA, size selected particles with a σg of 1.04 were produced at a flow rate of 90 slm and a sheath flow rate of 1100 slm (Q/q = 12). By increasing the aerosol flow rate and/or reducing the sheath flow rate the resolution of the DMA (given by the ratio Q/q) decreases and, hence, the width of the particle size distribution of the classified particles increases. It is not expected a priori to attain values of σg as low as 1.04 at the low sheath to aerosol flow ratios (Q/q = 2, 3) at which the HF-DMAs operate in the experiments. The analytical work undertaken in order to explain this result is described in the SI (Analytical Work section). The conclusion is that an ideal DMA operating with balanced flow at a sheath to aerosol ratio Q/q of 2 delivers size selected nanoparticles with a geometric standard deviation σg of 1.1. The reason lies in the relationship between the diameter dp and the electrical mobility Z for spherical nanoparticles (dp ∼ Z −0.5183).
It is noticed that the calculated value of σg (1.1) is larger than the value of σg (1.04–1.08) of the aerosol particles measured by the SMPS at the outlet of the HF-DMAs in the experiments. The SMPS measurements were performed with the NanoDMA operating at aerosol and sheath flow rates of 1.5 lpm and 15 lpm, respectively. The reasons for such discrepancy are not further investigated in this work. It is worthy to mention that similar values of σg (1.04–1.08) were obtained by Chen and Chein (Citation2006) when measuring with an SMPS the particle size distribution of monodisperse nanosized aerosols of sodium chloride (NaCl) and oleic acid selected by a reference DMA. Standalone DMAs and SPMS systems have been used to measure nanoparticle size standards and their accuracy in particle size has been assessed (Vasiliou Citation2005; Mulholland et al. Citation2006; Yook et al. Citation2008); however, values of σg are not reported in these works. It is claimed (Vasiliou Citation2005) that the limitations of the software render it difficult to accurately analyze the width of the particle size distribution of monodisperse aerosols measured by means of SMPS.
CONCLUSIONS
It has been analytically proven that an ideal DMA operating with balanced flow and a sheath to aerosol flow ratio of two classifies singly charged spherical particles with a geometric standard deviation in size σ g of 1.1. Two DMAs of cylindrical and rectangular plate electrodes have been demonstrated for producing monodisperse nanoparticles (SMPS σ g < 1.1) at gas flow rates from 200 slm to 500 slm when operating at a sheath to aerosol flow ratio of 2. The mean size (SMPS d g) and number concentration of the monodisperse particles vary between 5 nm and 50 nm and from 104 cm−3 to 106 cm−3, respectively, depending upon the aerosol source and the sheath flow rate used in the DMAs. These results are relevant to the cost of scaling up the production of monodisperse size selected nanoparticles by using multiple DMAs in parallel. By increasing the operational aerosol flow rate, the number of DMAs needed to reach a given particle yield is reduced. In addition, low sheath to aerosol flow ratios lead to higher particle yields and allow reducing the sheath gas pumping capacity.
FUNDING
This work was financially supported by the Deutsche Forschungsgemeinschaft (DFG) in the framework of the Collaborative Research Centre on “Nanoparticles from the gas phase: formation, structure and properties” (SFB 445). The rectangular plate DMA was funded by the Government of the Community of Madrid (FITA 49/2008). The assessment of the high-flow DMAs was partially financed by the European Union's Seventh Framework Program (EU FP7) under Grant Agreement No. 280765 (BUONAPART-E).
SUPPLEMENTAL MATERIAL
Supplemental data for this article can be accessed on
Final_Supplemental_Information.zip
Download Zip (178.2 KB)REFERENCES
- Chen , T. M. and Chein , H. M. 2006) . Generation and Evaluation of Monodisperse Sodium Chloride and Oleic Acid Nanoparticles . Aerosol Air Qual. Res. , 6 : 305 – 321 .
- Cook , R. F. and Kaiser , D. L. 2011) . “ Standards development for nanomaterials measurement ” . VA , , USA : National Institute of Standards and Technology (NIST), NanoRelease Steering Committee Workshop .
- Cui , H. , Feng , Y. , Wanzhong , R. , Zeng , T. , Lv , H. and Yanfei , P. 2009 . Strategies of Large Scale Synthesis of Monodisperse Nanoparticles . Recent Pat. Nanotechnol., , 3 : 32 – 41 .
- Groth , J. and Johansson , A. V. 1988 . Turbulence Reduction by Screens . J. Fluid Mech. , 197 : 139 – 155 .
- Hontañón , E. and Kruis , F. E. 2009 . A DMA for Size Selection of Nanoparticles at High Flow Rates . Aerosol Sci. Technol. , 43 : 25 – 37 .
- Hontañón , E. , Palomares , J. M. , Stein , M. , Guo , X. , Engeln , R. Nirschl , H. 2013 . The Transition from Spark to arc Discharge and its Implications with Respect to Nanoparticle Production . J. Nanop. Res , 15 : 1957 Article No
- Knutson , E. O. and Whitby , K. T. 1975 . Aerosol Classification by Electric Mobility: Apparatus, Theory, and Applications . J. Aerosol Sci. , 6 : 443 – 451 .
- Laws , E. M. and Livesey , J. L. 1978 . Flow Through Screens . Ann. Rev. Fluid Mech. , 10 : 247 – 266 .
- Masala , O. and Seshadri , R. 2004 . Synthesis Routes for Large Volumes of Nanoparticles . Annu. Rev. Mater. Res. , 34 : 41 – 81 .
- Mulholland , G. W. , Donnelly , M. K. , Hagwood , C. R. , Kukuck , R. S. and Hackley , V. A. 2006 . Measurement of 100 nm and 60 nm Particle Standards by Differential Mobility Analysis . J. Res. Natl. Inst. Stand. Technol. , 111 : 257 – 312 .
- Oshinowo , L. and Kuhn , D. C. S. 2000 . Turbulence Decay Behind Expanded Metal Screens . Can. J. Chem. Eng. , 78 : 1032 – 1039 .
- Peng , S. 2009 . Novel Monodisperse Nanoparticles: Synthesis, Characterization and Applications , Rhode Island , , USA : PhD Thesis, Brown University .
- Rosell-Llompart , J. , Loscertales , I. G. , Bingham , D. and Fernández de la Mora , J. 1996 . Sizing Nanoparticles and Ions with a Short Differential Mobility Analyzer . J. Aerosol Sci. , 5 : 695 – 719 .
- Santos , J. P. , Hontañón , E. , Ramiro , E. and Alonso , M. 2009 . Performance Evaluation of a High Resolution Parallel Plate Differential Mobility Analyzer . Atmos. Chem. Phys. Discuss. , 9 : 2419 – 2429 .
- Vasiliou , J. 2005 . An Evaluation of a Scanning Mobility Particle Sizer with NIST-Traceable Particle Size Standards . 2 : 1 – 4 . NSTI-Nanotech, ISBN 0–9767985
- Yook , S. J. , Fissan , H. , Engelke , T. , Asbach , C. , van der Zwaag , T. Kim , J. H. 2008 . Classification of Highly Monodisperse Nanoparticles of NIST. Traceable Sizes by TDMA and Control of Deposition Spot Size on a Surface by Electrophoresis . J. Aerosol Sci. , 39 : 537 – 548 .
- Zhang , S. H. , Akutsu , Y. , Rusell , L. M. , Flagan , R. C. and Seinfeld , J. H. 1995 . Radial Differential Mobility Analyzer . Aerosol Sci. Technol. , 23 : 357 – 372 .