Abstract
Copyright 2014 American Association for Aerosol Research
1. INTRODUCTION
Characterizing the organic composition of marine aerosol particles is important for understanding the sources of marine aerosol and their impact on cloud microphysical properties (de Leeuw et al. Citation2011). A variety of measurement techniques have been used to measure the organic composition of both ambient atmospheric and freshly emitted sea spray aerosol (Table S1 in the online supplementary information [SI]). Using Fourier transform infrared (FTIR) spectroscopy, atmospheric aerosol particles collected in marine regions on multiple shipboard campaigns were shown to be saccharide-like based on their functional group composition, with a high ratio of oxygen to carbon (O/C) (Russell et al. Citation2010). Similarly, using scanning transmission X-ray microscopy with near-edge X-ray absorption fine structure (STXM-NEXAFS), Russell et al. (Citation2010) and Hawkins and Russell (Citation2010) found chemically distinct ambient marine particle types including saccharide-like components on sea salt particles and protein particles. Model ocean systems have been used to generate nascent sea spray aerosol (SSA) from seawater (Keene et al. Citation2007; Bates et al. Citation2012), to determine the organic composition of particles directly emitted from wave breaking and bubble bursting at the sea surface (Table S1). FTIR spectroscopy showed the organic composition of these generated nascent SSA, hereafter referred to as generated marine particles, also to be highly oxidized (Bates et al. Citation2012). In contrast, using high resolution time of flight aerosol mass spectrometry (HR-ToF-AMS) in the same study, Bates et al. (Citation2012) found that generated marine aerosol particles were highly unsaturated and minimally oxidized (low O/C).
In this study, we resolve this apparent discrepancy by comparing the measured organic composition of ambient and generated marine aerosol particles using these three techniques as well as a light scattering module of the HR-ToF-AMS (LS-ToF-AMS). Each of these methods is used to calculate the level of oxidation of the organic mass (OM) and assess the differences in the composition of ambient and generated marine particles. Additionally, we consider the extent to which each method provides additional insight into the particle composition.
2. MEASUREMENTS OF THE ORGANIC COMPOSITION OF AMBIENT AND GENERATED MARINE PARTICLES
Ambient atmospheric, which frequently included non-marine particles from the U.S east coast and shipping sources, and generated marine aerosol particles were sampled aboard the research vessel Ronald H. Brown during the Western Atlantic Climate Study (WACS) 19–28 August 2012. Ambient particles were sampled through two side-by-side humidity and temperature controlled masts ∼18 m above sea level (Bates et al. Citation2002). The Sea Sweep model ocean system (Bates et al. Citation2012) was used to generate marine aerosol particles. Particles were characterized using four complementary techniques (): (i) FTIR spectroscopy (Russell et al. Citation2010); (ii) HR-ToF-AMS (DeCarlo et al. Citation2006); (iii) LS-ToF-AMS (Liu et al. Citation2013); (iv) STXM-NEXAFS (Hawkins and Russell Citation2010). The SI has details for particle collection and OM analysis, as well as organic carbon (OC) measurements by evolved gas analysis (EGA) of quartz filters. The range of particle aerodynamic diameters (da) measured by each method is shown in and Figure S1.
TABLE 1 Descriptions of the four techniques used to measure marine organic composition and the separation of the low and high O/C OM
Based on FTIR spectroscopy, the OM in generated marine particles contained on average 46% hydroxyl, 41% alkane, and 13% amine functional groups (). The average composition of the ambient OM was 18% hydroxyl, 45% alkane, 2% amine, and 35% carboxylic acid functional groups (). This corresponds to average O/C values of 0.55 ± 0.17 and 0.51 ± 0.22 for ambient and generated marine particles, respectively (Table S3). Carboxylic acid and hydroxyl functional groups have high O/C ratios, while alkane and amine functional groups contain no oxygen (O/C = 0) and are grouped here as low O/C (; and 1m). Using this distinction, the generated OM was 46% high O/C and 54% low O/C organic components (±12%), while the ambient OM was 53% and 47% ±10%, respectively (). This composition is similar to previous FTIR spectroscopy measurements of generated marine OM with 53% high O/C and 47% low O/C (Bates et al. Citation2012) and ambient marine OM with 55% high O/C and 45% low O/C (including 4% organosulfate functional groups) (Hawkins et al. Citation2010).
FIG. 1 Normalized spectra of (a–d) generated and (e–h) ambient marine OM and (i–l) high O/C and (m–p) low O/C particle OM types, colored across the rows as dark blue, bronze, light pink, and dark green, respectively. Columns represent the four measurement techniques. FTIR spectroscopy pies show the average functional group composition including: carboxylic acid (lime green), hydroxyl (bright pink), amine (orange), and alkane (blue). The color bars show the functional group absorption regions, using the same colors. HR-ToF-AMS pies show the average OM mass fragment group composition with CXHYO>1 (gray), CXHYO1 (dark purple), and CXHY (teal). Specific m/z values are labeled in the HR-ToF-AMS and LS-ToF-AMS panels. In the STXM-NEXAFS panel, density maps (inset in l and p) illustrate the typical particle morphology and are colored as low (blue) to high (red) probability of carbon. Color bars (left to right) represent aromatic (black), alkyl (purple), carboxylic carbonyl (brown), and alcohol (red) functional group and potassium (yellow) absorption regions for the STXM-NEXAFS spectra.
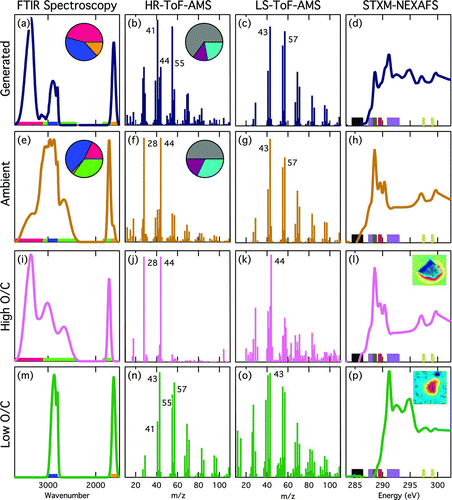
FIG. 2 Comparison of the OM composition for the (a) generated marine and (b) ambient atmospheric marine particles. The OM is separated into high O/C (pink) and low O/C (green). Gray shading in the FTIR pies represents uncertainty (see the SI). For the HR-ToF-AMS pies, the lighter shading shows the CEIC that was applied, gray shading is the uncertainty (see the SI), and the dark gray is the percent difference between the FTIR and HR-ToF-AMS OM. In the LS-ToF-AMS pies, the light gray area is the number based CELS. The individual circles that make up the STXM-NEXAFS pies represent the small number of particles measured by the technique, and the numbers of particles are written in each section of the pie. The light gray shading represents the overall lower particle statistics and higher uncertainty for the STXM-NEXAFS measurements. For the HR-ToF-AMS and FTIR spectroscopy pies, the percent of the total OM that is high and low O/C are included, and for the LS-ToF-AMS, the percent of total organic particles that are high and low O/C are included.
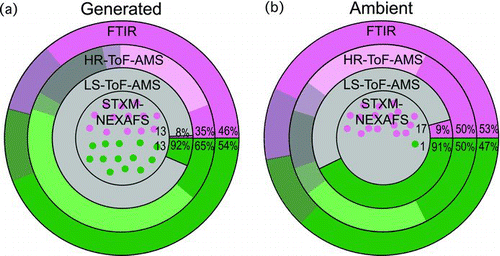
The organic composition measured by the HR-ToF-AMS was split into ion families based on high-resolution data analysis (SI). The first group is CXHY, which has the characteristic ion pattern from the alkane series CxH2y-1 + and CxH2y+1 +, with C3H5 + (m/z = 41), C3H7 + (m/z = 43), C4H7 + (m/z = 55) and C4H9 + (m/z = 57) among the main peaks. The second group is represented by CxHyO1 +, which is slightly oxidized and typically contains C2H3O+ (m/z = 43) and CHO+ (m/z = 29). The third group is CxHyO.>1 +, which contains the most oxidized ions and has the highest content of CO2 + (m/z = 44).
The generated and ambient marine OM compositions were 22% CXHYO>1, 13% CXHYO1, and 65% CXHY and 32% CXHYO>1, 18% CXHYO1, and 50% CXHY, respectively ( and 1f). This composition is consistent with previous HR-ToF-AMS results from similar measurements (Bates et al. Citation2012). Other organic fragment groups, including CXHYO>1N, CXHYO1N, and CXHYN, contribute less than 2% to the total OM and are excluded from this analysis. When grouping CXHYO1 and CXHYO>1 as high O/C and CXHY as low O/C (and 1n), the composition of the generated OM is 35% high O/C and 65% low O/C, while the ambient OM is 50% high O/C and 50% low O/C (). This corresponds to average O/C values of 0.63 ± 0.11 for ambient aerosol particles and 0.20 ± 0.08 for generated marine particles, respectively (Table S3).
The HR-ToF-AMS collection efficiency (which includes lens transmission efficiency, detector efficiency, and losses due to particle bounce) was calculated by comparing HR-ToF-AMS sulfate to IC sulfate (CEIC, see the SI). The ambient CEIC is 0.35, and the generated CEIC is 0.17 (lighter shading in ). After the CEIC was applied to the HR-ToF-AMS measurements, the FTIR OM and HR-ToF-AMS OM agreed within the instrument uncertainties with an overall average percent difference of 12%. Correlations are shown in Figure S2. Because the CEIC may be size-dependent, the composition of the unmeasured OM may not be the same as the measured OM. Comparisons of the FTIR and HR-ToF-AMS OM with EGA OC are shown in the SI. The HR-ToF-AMS measurements were also used to calculate the mass of sea salt and the CEtotal, which includes sea salt (CESS), described in the SI.
The LS-ToF-AMS optically detected 7,300 generated and 360 ambient single particles, and their average spectra are shown in and 1g, respectively. Of the ambient single particles that were optically detected, 47% of the particles had corresponding mass spectra that had total signal to noise (S/N) exceeding 3 (LS+MS) (SI). This result is consistent with previously measured LS+MS fractions of 0.52 (Liu et al. Citation2013). This corresponds to a total ambient LS-ToF-AMS CE (CELSA) of 0.47 (gray area of ), which is consistent with the HR-ToF-AMS CEtotalA of 0.49 (which includes sea salt, see the SI). For the generated particle sampling, only 7% of the particles had corresponding mass spectra with total S/N exceeding 3 (LS+MS), which corresponds to a generated marine CELSG of 0.07 (gray area of ) and is consistent with CEtotalG of 0.07 (SI).
The LS+MS particle mass spectra were clustered based on their organic signal using the method outlined by Liu et al. (Citation2013). To identify organic types, only signals at selected organic m/z values were used (m/z 20, 22, 24–27, 29, 31, 40–45, 47, 49–59, 61–63, 65–79, 82–90, 92–97, 98–110). Particles with spectra that contained less than six organic ions (∼50% of the LS+MS particles) were not included in the clustering. The cluster analysis separated the particles with CXHY hydrocarbon-like signatures (low O/C), including m/z 41, 43, 55, 57 (), from those with m/z 44 (high O/C, due to the oxidized CO2 + fragment) (). The organic fraction of ambient particles is 9% high O/C and 91% low O/C, while the generated particles is 8% high O/C and 92% low O/C ().
The average STXM-NEXAFS spectra of the generated and ambient marine particles are shown in and 1h, respectively. The particle spectra were grouped using Ward cluster analysis, resulting in two types: high O/C and low O/C organics, based on functional group peaks. The high O/C group () contains particle spectra with high absorbance in the carboxylic carbonyl region (288.2–288.9 eV) and varying absorption in the alcohol region (289.5 eV), which are both oxidized and considered high O/C. The second type of particle spectra, low O/C group (), did not contain oxidized functional groups but instead showed absorbance only in the two alkyl regions (287.4–288.5 eV for C-H bonds and 290.8–293 eV for C-C bonds). One spectrum in this type also had absorbance from 284.4–286.4 eV, indicative of aromatic or alkene functional groups. Of the 26 generated particles, 13 had high O/C spectra. For the ambient particles, 17 of 18 particles had high O/C spectra (). The particle morphologies provided by STXM-NEXAFS are discussed in the SI. All of the generated high O/C particles were identified as similar to saccharides on sea salt, indicating that high O/C OM in the generated particles is associated with sea salt.
3. COMPARISON OF MEASUREMENT TECHNIQUES
The OM composition of ambient atmospheric and generated marine aerosol was measured by four complementary techniques and classified into two types of OM: high O/C OM which is highly oxidized and low O/C OM which has little or no oxidization. For the four techniques, OM was separated by: (i) functional groups with high and low O/C (FTIR spectroscopy), (ii) mass fragments with CXHYO>1, CXHYO1, and CXHY (HR-ToF-AMS), (iii) single particles with high m/z 44 or CXHY signatures (LS-ToF-AMS), and (iv) single particles that were alkyl-like or oxidized (STXM-NEXAFS). The high and low O/C classification of OM provides a basis to compare the four measurement techniques (). While the techniques have different capabilities for sampling the high and low O/C OM compositions, the composition of the particles measured by HR-ToF-AMS and FTIR spectroscopy are generally consistent. The larger differences in the generated marine particle composition can be explained by the influence of the high fraction of larger diameter sea salt containing particles that were missed by the AMS techniques and by the uncertainties of both the FTIR and AMS techniques.
STXM-NEXAFS measurements provide examples of single-particle morphology for two generated marine particle types: high O/C OM on sea salt particles (similar to particles identified previously as saccharides on sea salt (Hawkins and Russell Citation2010)) and low O/C OM particles (l and 1p). Of the ambient particles, 13 of 17 of the high O/C particles were also similar to saccharides on sea salt (l). This morphology of a small fraction of saccharide-like organic components compared to sea salt (for particles with da of 0.47 to 6.4 μm that the STXM-NEXAFS sees) results in particles that are largely refractory at 650°C and, hence, may bounce off the vaporizer. The alkyl-like (low O/C OM) particle type has a more uniform morphology with OM throughout the particle and no evidence of sea salt (), consistent with these particles being vaporized more efficiently at 650°C than the high O/C OM on sea salt. The less than 100 particles analyzed with this technique were selected manually based on the carbon content detected and do not represent the entire OM particle population. However, the identification of both high and low O/C particle types provides consistency with the FTIR and AMS techniques, in addition to showing that the high O/C OM is typically present on the sea-salt-containing particles, especially in the generated marine aerosol.
The HR-ToF-AMS and FTIR measured compositions are similar for ambient particles both with approximately 50% high O/C OM, but there is a larger difference in the generated OM with a HR-ToF-AMS high O/C fraction of 35% and an FTIR high O/C fraction of 46% (). This larger difference is due to the high (89%) sea salt fraction of these particles (SI). The HR-ToF-AMS measures nonrefractory particles only and is influenced by particle bounce effects. Even at 650°C, sea salt is inefficiently vaporized in the AMS. Any OM present on sea salt particles, as observed in the STXM-NEXAFS morphology, is also inefficiently vaporized in the HR-ToF-AMS. The larger fraction of low O/C OM measured by HR-ToF-AMS, compared to FTIR is a result of the high O/C OM that is associated with sea salt and low transmission efficiency of the larger sea salt particles.
The OM composition as determined by FTIR spectroscopy and HR-ToF-AMS are more similar for the ambient particles (within 10%, ), due to the smaller fraction of sea salt in the ambient particles. If all of the sea salt (3% of the particle mass) is from sea spray and has the same ratio of OM to sea salt as the generated particles (11:89, Table S2), only 0.37% of the particle mass is associated with sea salt, which is less than 1% of the total OM. The OM that is not associated with sea salt is nonrefractory and less influenced by bounce effects and is sampled efficiently by both the FTIR and CE-corrected AMS techniques.
The LS-ToF-AMS measurements demonstrate that a large number (93%) of generated marine particles are not vaporized in the AMS and are refractory or bounce off the vaporizer, even though they are optically detected by light scattering. The large fraction of LS-only particles observed during sampling generated marine particles is consistent with a large fraction of sea salt in those particles, compared to the lower fraction of sea salt in the ambient particles. The submicron generated particles were 89% sea salt (Table S2), consistent with an LS-only fraction of 93%. Additionally, the ratio of LS+MS particles to total particles observed by light scattering is negatively correlated with sea-salt concentrations (r = −0.60). This result implies that the sea-salt particles detected by LS are not vaporized (no mass spectrum with total S/N exceeding 3 is obtained), consistent with the refractory nature of sea salt (which contributes to particle bounce) and the morphology measured by STXM-NEXAFS.
The composition of the LS-ToF-AMS particles includes a much higher low O/C fraction than the other techniques, for both generated and ambient particles (). This is the result of a disproportionately low representation of the high O/C OM associated with refractory sea salt. Additionally, the LS-ToF-AMS measures single particles in a limited size range, which may not be directly comparable to the other techniques that include larger (STXM-NEXAFS and FTIR) and smaller (HR-ToF-AMS) particles ( and Figure S1). See the SI for more discussion.
4. IMPLICATIONS FOR MARINE ORGANIC AEROSOL COMPOSITION
The results of the HR-ToF-AMS and FTIR measurement techniques show consistent OM concentrations and comparable high and low O/C fractions for ambient atmospheric aerosol. The discrepancy in the results was greater for the generated OM, consistent with the larger fraction of refractory particles. The sea-salt particles and associated OM do not vaporize at 650°C and are more prone to particle bounce. For both aerosol types, the high O/C OM consists of hydroxyl and carboxylic acid functional groups measured by FTIR spectroscopy and STXM-NEXAFS and mass fragments of the CXHYO1 and CXHYO>1 groups measured by LS-ToF-AMS and HR-ToF-AMS. The low O/C OM consists of alkane and alkyl functional groups measured by FTIR spectroscopy and STXM-NEXAFS and CXHY mass fragments measured by LS-ToF-AMS and HR-ToF- AMS.
The FTIR spectroscopy and HR-ToF-AMS high and low O/C fractional composition show good agreement for the ambient marine measurements, with O/C approximately 50% of the total OM. The generated marine OM fractions measured by the HR-ToF-AMS and FTIR spectroscopy have differences up to 31%. This is consistent with the larger fraction of sea-salt particles in the generated marine OM, compared to the ambient marine OM and the larger fraction of high O/C OM associated with the sea-salt particles. With the CEIC correction applied, the average ratio of HR-ToF-AMS to FTIR spectroscopy OM is 0.86, which is within the 20% uncertainty for these measurements.
STXM-NEXAFS measurements show both high O/C and low O/C particles are present in the generated marine OM, which is similar to the FTIR spectroscopy and HR-ToF-AMS measured compositions. The difference in the generated and ambient marine particle composition compared to FTIR spectroscopy and HR-ToF-AMS is likely the result of the low counting statistics and the selection of particles for analysis. The LS-ToF-AMS measurements demonstrate that a large number of the optically detected marine generated particles are not vaporized in the AMS. The result is a disproportionately high representation of the low O/C particles in the mass spectra for the LS-ToF-AMS (SI).
ACKNOWLEDGMENTS
The authors gratefully acknowledge Leah Williams, William Brooks, Timothy Onasch, Donna Sueper, and Shang Liu for their assistance in preparing the HR-ToF-AMS and LS-ToF-AMS for deployment and for guidance with the LS-ToF-AMS analysis. They thank the captain, crew, and scientists aboard the Ronald H. Brown for their support. The authors thank Derek Coffman, Drew Hamilton, and Janin Guzman-Morales for assistance with sample collection. They acknowledge David Kilcoyne at the Lawrence Berkeley National Laboratory Advanced Light Source and Satoshi Takahama for assistance with STXM-NEXAFS and beamline operation.
SUPPLEMENTAL MATERIAL
Supplemental data for this article can be accessed on the publisher's website.
Supplemental
Download PDF (303.4 KB)REFERENCES
- Bates , T. S. 2012 . Measurements of Ocean Derived Aerosol off the Coast of California . J. Geophys. Res.–Atmos. , 117 ( D00V15 ) doi: 10.1029/2012JD017588
- Bates , T. S. , Coffman , D. J. , Covert , D. S. and Quinn , P. K. 2002 . Regional Marine Boundary Layer Aerosol Size Distributions in the Indian, Atlantic, and Pacific Oceans: A Comparison of INDOEX Measurements With ACE-1, ACE-2, and Aerosols99 . J. Geophys. Res.-Atmos. , 107 ( D19 ) doi: 10.1029/2001jd001174
- DeCarlo , P. F. 2006 . Field-Deployable, High-Resolution, Time-of-Flight Aerosol Mass Spectrometer . Anal. Chem. , 78 ( 24 ) : 8281 – 8289 . doi: 10.1021/ac061249n
- de Leeuw , G. , Andreas , E. L. , Anguelova , M. D. , Fairall , C. W. , Lewis , E. R. O’Dowd , C. 2011 . Production Flux of Sea Spray Aerosol . Rev. Geophys. , 49 doi: 10.1029/2010rg000349
- Gussman , R. A. , Kenny , L. C. , Labickas , M. and Norton , P. 2002 . Design, Calibration, and Field Test of a Cyclone for PM1 Ambient Air Sampling . Aerosol Sci. Technol. , 36 ( 3 ) : 361 – 365 . doi: 10.1080/027868202753504461
- Hawkins , L. N. and Russell , L. M. 2010 . Polysaccharides, Proteins, and Phytoplankton Fragments: Four Chemically Distinct Types of Marine Primary Organic Aerosol Classified by Single Particle Spectromicroscopy . Adv. Meteorol. , doi: 10.1155/2010/612132
- Hawkins , L. N. , Russell , L. M. , Covert , D. S. , Quinn , P. K. and Bates , T. S. 2010 . Carboxylic Acids, Sulfates, and Organosulfates in Processed Continental Organic Aerosol Over the Southeast Pacific Ocean During VOCALS-REx 2008 . J. Geophys. Res.-Atmos. , 115 ( D13201 ) doi: 10.1029/2009jd013276
- Jayne , J. T. , Leard , D. C. , Zhang , X. F. , Davidovits , P. , Smith , K. A. , Kolb , C. E. and Worsnop , D. R. 2000 . Development of an Aerosol Mass Spectrometer for Size and Composition Analysis of Submicron Particles . Aerosol Sci. Technol. , 33 ( 1–2 ) : 49 – 70 .
- Keene , W. C. 2007 . Chemical and Physical Characteristics of Nascent Aerosols Produced by Bursting Bubbles at a Model Air-Sea Interface . J. Geophys. Res.-Atmos. , 112 ( D21202 ) doi: 10.1029/2007jd008464
- Liu , S. , Russell , L. M. , Sueper , D. T. and Onasch , T. B. 2013 . Organic Particle Types by Single-Particle Measurements Using a Time-of-Flight Aerosol Mass Spectrometer Coupled With a Light Scattering Module . Atmos. Meas. Tech. , 6 ( 2 ) : 187 – 197 . doi: 10.5194/amt-6-187-2013
- Russell , L. M. , Hawkins , L. N. , Frossard , A. A. , Quinn , P. K. and Bates , T. S. 2010 . Carbohydrate-Like Composition of Submicron Atmospheric Particles and Their Production From Ocean Bubble Bursting . Proc. Natl. Acad. Sci. USA , 107 ( 15 ) : 6652 – 6657 . doi: 10.1073/pnas.0908905107
- Williams , L. R. 2013 . Characterization of an Aerodynamic Lens for Transmitting Particles Greater Than 1 Micrometer in Diameter Into the Aerodyne Aerosol Mass Spectrometer . Atmos. Meas. Tech. , 6 : 3271 – 3280 . doi: 10.5194/amt-6-3271-2013