Abstract
Understanding the filtration characteristics of fibrous particles is important since those particles have caused health and environmental concerns. Due to the straight morphology of metal nanowires, unlike carbon nanotube (CNT) particles nanowires can be considered as appropriate test material to evaluate existing filtration theory for cylindrical particles. We measured the penetration of silver nanowires in the size range of dm = 200 to 400 nm through screen mesh filter. By using Li et al. (2012)'s theory, we determined the orientation status of silver nanowires inside differential mobility analyzer (DMA) and calculated the dynamic shape factor of nanowires. Theoretical penetration was obtained by using single fiber theory with modified interception parameter including orientation angle between a filter wire and a particle. The orientation angle obtained by fitting experimental data into single fiber theory for the 1 layer of screen mesh filter is found to be close to 40° indicating random orientation of nanowires near filter. However, in the experiments with multi-layers of screen mesh, any tendency related to the orientation angle was not found. We performed numerical simulations for the filtration processes such as impaction, diffusion, interception, and interception of diffusing particles by introducing modified slip correction factor. Overall, when interception of diffusing particles is considered in addition to diffusion and interception, numerically simulation results and theoretical prediction agree better with experimental data regarding the penetration of silver nanowires through the 1 layer of screen mesh filter.
Copyright 2014 American Association for Aerosol Research
1. INTRODUCTION
Particles with high aspect ratio such as nanowires, carbon nanotubes (CNTs), and nanorods have been widely used with various applications because they have unique chemical, mechanical, electrical, and optical properties. But, it is known that those particles have caused health and environmental problems (Ji et al. Citation2012). Filtration technology for those particles has been used as one of the primary technologies for nanoparticle control. Especially, among fibrous particles, filtration of CNT particles (Seto et al. Citation2010; Wang et al. Citation2011a,b; Bahk et al. Citation2013; Wang and Pui Citation2013), and asbestos (Cheng et al. Citation2006; Vallero et al. Citation2008) have been mainly studied.
There are four main mechanisms for single fiber theory such as inertial impaction, interception, diffusion, and interception of diffusing particles (Hinds Citation1999). Particle size and morphology determine a dominant filtration mechanism. Diffusion is the most important filtration mechanism for particles below 100 nm (Wang et al. Citation2007). For the case of agglomerate particles, as the aspect ratio of the particles becomes higher (or the length of agglomerates becomes larger), the influence of interception on the collection efficiency becomes more significant (Kim et al. Citation2009). Similar to agglomerate particles, fibrous particles with higher aspect ratio can be more collected by the interception mechanism.
Penetration of CNT particles through air filters has been studied (Seto et al. Citation2010; Wang et al. Citation2011b; Bahk et al. Citation2013). In those previous studies, theoretical prediction was conducted based on single fiber theory by modeling CNT as a straight cylinder. However, CNT particles are not appropriate to evaluate existing filtration theory because CNT particles mostly have bents and kinks. Unlike CNT particles, metal nanowires can be considered as appropriate material to compare experimental data with theoretical prediction regarding the penetration of cylindrical particles through air filter since metal nanowires have straight morphology. The penetration of the fibrous particles through the fibrous filter or screen filter was numerically computed through computational fluid dynamics (CFD) simulations (Wang et al. Citation2007; Kim et al. Citation2009; Liu et al. Citation2011). Regarding the penetration of the fibrous particles through filter numerically predicted results were higher than experimental results. There can be two reasons. Commercial filter media is inhomogeneous while the model filter used in CFD simulations homogenous, which can result in discrepancy between the numerical and experimental results (Podgorski et al. Citation2011). Also, it is difficult to consider orientation angle that is the azimuth between the filter wire and the fibrous particle for each particle in CFD simulations for the prediction of particle penetration. For the reason, there can be deviation of numerical results from experimental data (Wang et al. Citation2011a).
CNT particles have been used for experiments of fibrous particle filtration with consideration of orientation angle. In Seto et al. (Citation2010)'s study, comparison of single fiber theory related to inertial impaction, interception, diffusion, and gravity with experiments about the penetration of CNT particles through membrane filter was carried out in order to determine orientation angle according to each face velocity. Wang et al. (Citation2011a) carried out numerical simulations related to inertial impaction, interception and diffusion, and experimental filtration study for 1 layer screen mesh filter considering theoretical orientation angle (≈40°). Wang et al. (Citation2011b) compared theoretical prediction related to inertial impaction, interception, diffusion, and interception of diffusing particles with experimental data regarding the penetration of CNT particles through 20 layers of screen mesh filter. Wang et al. (Citation2011b) compared three interception parameter models for theoretical prediction of CNT penetration through screen mesh filter. Bahk et al. (Citation2013) estimated the length of CNT particles from experimental data related to the penetration of CNT particles through 20 layers of screen mesh filter by combining single fiber theory regarding inertial impaction, interception, diffusion, and interception of diffusing particles with the average diameter of CNT particles obtained from scanning electron microscope (SEM) image analysis.
For fibrous particles, it is important to determine orientation angle in order to calculate the collection of fibrous particles by interception. The fibrous particles can have different interception parameter depending on the orientation angle at every moment because particles rotate in flow field. The angle can be determined by combining theoretical prediction and experiment data (Seto et al. Citation2010). Seto et al. (Citation2010) experimentally obtained orientation angles according to face velocities. Wang et al. (Citation2011a) computed the average orientation angle based on the ratio of particle rotation time to time for the CNT to pass by the wire.
In this study, we carried out theoretical prediction, numerical prediction, and experimental measurement on the penetration of silver nanowires through a screen filter as a function of electrical mobility diameter. The length and diameter of monodisperse silver nanowires were obtained by image analysis according to their mobility diameters. For the calculation of theoretical and numerical penetration, we determined how silver nanowires were oriented inside DMA based on Li et al.'s (2012) theory. In order to calculate the drag force on silver nanowires outside DMA, the dynamic shape factor of nanowires is considered. Also, the effect of orientation angle on the interception parameter and particle collection by interception was also discussed. By matching experimental data with theoretical prediction regarding the penetration of silver nanowires through screen filter, average orientation angles between screen filter wires and silver nanowires were obtained. For the case of l layer, we also numerically predicted the penetration of nanowires through screen filter considering particle collection mechanisms such as diffusion, interception, interception of diffusing particles, and impaction in CFD simulations. CFD results were compared with theoretical prediction and experimental data.
2. EXPERIMENTAL METHODS
To evaluate existing filtration theory for cylindrical particles, it is necessary to obtain very well defined source material in an unaggregated state suitable for collision atomizer and classify monodisperse particles with DMA. Silver nanowires used in experiments were synthesized in a modified polyol reduction process. 45 mL of Glycerol and 1.83 g of poly vinyl pirrolidone (PVP) were injected to the clean flask, which was then immersed into oil bath to dissolve PVP at 100°C. While dissolving, 1.7 mM NaCl solution in 5 mL of glycerol was prepared and added drop wise into PVP solution after the temperature of the flask dropped to 50°C. After injecting 0.4 g of AgNO3 into the flask, the solution was gradually heated up to 160°C. The color of the solution turned from pale white into bright orange, red, and finally into gray-green. To control the length of silver nanowires, PVP:AgNO3 molar ratio was modulated, and silver nanowires synthesized in this way have a length of 1∼2 μm and a diameter of 70 nm. Methanol was added to as-obtained silver nanowire solution with 1:9 volume fraction to remove the PVP residue, and the mixture was centrifuged. After several centrifuge processes, the washed silver nanowires were collected and dispersed into a methanol solution of 0.02 g/mL. The nanowires were synthesized to have a very small distribution of diameter but a wide distribution of length.
In order to determine particle size distribution, silver nanowires in deionized water were generated by atomizer and passed through a diffusion dryer and a neutralizer (Po-210). Mono-disperse particles were classified by DMA (TSI 3081), and the concentration of particles was counted by condensation particle counter (TSI CPC Model 3775). As shown as , there are two peaks around mobility diameter dm = 30 nm and dm = 168 nm. One peak around 30 nm is attributed to residue in deionized water and the other peak around 168 nm to silver nanowires. Thus, particle size range of silver nanowires to be used in our experiments was determined to be 200 to 400 nm.
a shows a schematic diagram for set-up for sampling particles. In the set-up, nanowires in the size range of mobility diameter dm = 200 to 400 nm were classified by DMA where sheath flow rate was set to 6 lpm and then sampled on the silicon substrates using a nanoparticle collector (HCT-4650) where sampling flow rate was set to 1.5 lpm.
FIG. 2 Schematic diagram: (a) set-up for sampling particles and (b) set-up for measuring penetration.
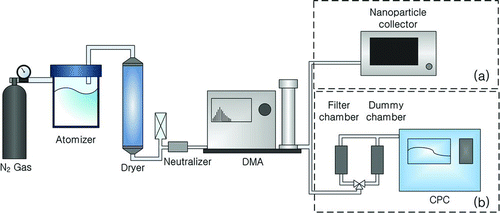
shows a set-up for the measurement of experimental penetration of silver nanowires through screen mesh filter. Silver nanowires generated by the atomizer are classified depending on mobility diameter by the DMA and their penetration through a screen filter is defined as the ratio of particle concentration of nanowires through filter holder chamber to that through dummy chamber:
For the determination of particle morphology such as the diameter and length of silver nanowires, SEM images analysis was conducted for polydisperse (unclassified) and monodisperse silver nanowires (dm = 200 nm, 250 nm, 300 nm, 350 nm, 400 nm) using Image J s/w. As shown in , nanowires have straight shape unlike CNT particles with bents and kink. Thus, it is advantageous to use nanowires for a comparison between theoretical and numerical predictions and experimental data for the penetration of nanowire through filter. In , histograms of average length and diameter of monodisperse silver nanowires are plotted. The average diameter is 70 nm and the average length is from 1005 to 2169 nm.
FIG. 3 SEM images: (a) polydisperse (unclassified) silver nanowires, (b) monodisperse silver nanowire (dm = 200 nm), (c) monodisperse silver nanowire (dm = 250 nm), (d) monodisperse silver nanowire (dm = 300 nm), (e) monodisperse silver nanowire (dm = 350 nm), and (f) monodisperse silver nanowire (dm = 400 nm).
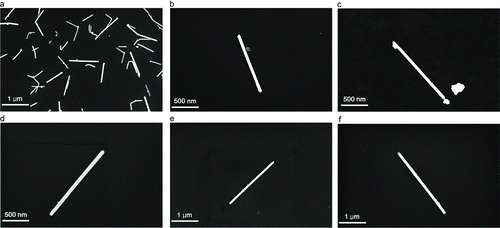
3. DETERMINATION OF CYLINDRICAL PARTICLE ORIENTATION
Li et al. (Citation2012) proposed an analytical model that can determine the orientation status of cylindrical particles from relation between the geometrical length and electrical mobility diameter. The average electrical mobility in DMA is defined as (Li et al. Citation2012):
Li et al. (Citation2012) developed an orientation-averaged electrical mobility theory for rigid axis symmetric particles undergoing Brownian motion by considering the electrical polarization of the particles in an electric field. The theory was validated by experimental results of monodisperse gold rods (Li et al. Citation2013). Since silver nanowires are conducting rod like gold rods, we considered only the induced dipole polarization energy in present study. ⟨cos2θ⟩ can be calculated from complicated equation (Equation (22) in Li et al. [Citation2012]). |Zp −Z p,random|/Z p,random of silver nanowire according to their morphology can be calculated by combining Equation (2) and Equation (22) in Li et al. (Citation2012) using Mathematica as shown in . In the calculation of |Zp −Z p,random|/Z p,random, we considered the average length and diameter of silver nanowires obtained from SEM images analysis. From Knutson and Whitby's (Citation1975) expression for the mean electrical mobility of particles exiting a DMA, the size-selecting voltage of DMA is expressed as:
4. THEORY FOR FILTRATION
One can theoretically compute the penetration of the nanowires using single fiber filtration theory (Hinds 1999).
Equation for the efficiency by inertial impaction, EI , is shown in where
TABLE 1 Equations depending on deposition mechanism (see Bahk et al. Citation2013)
is Stokes number,
is Kuwabara hydrodynamic parameter, ρ
p
is the density of silver, dw
is the diameter of the silver nanowire, and R
ae is the aerodynamic radius of fibrous particle depending on aspect ratio defined by Equation (14) in Bahk et al. (Citation2013).
Equation for the efficiency by diffusion, ED
, is also shown in where (Peclet number) is defined as:
FIG. 6 Screen filter made of 300-mesh type 304 stainless steel: (a) SEM image and (b) modeled screen filter.
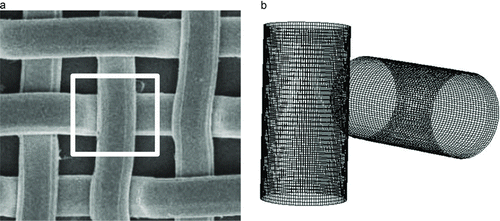
Filtration efficiency by interception of spherical particles onto filter fiber is expressed as a function of interception parameter, R=(dp /df ) like Equation (6c). However, for the case of silver nanowires, the filtration efficiency by interception is obtained with corrected interception parameter R' expressed as (Bahk et al. Citation2013):
5. NUMERICAL METHOD
(a) shows the SEM image of screen filter used in our experiments. The thickness of filter was measured to be 95 μm using a micrometer and the weight of screen filter per 100 cm2 to be 2.17 g using a micro balance. From SEM image analysis the average diameter of wires on screen filter is measured to be 37.5 μm. The solidity (α) of screen filter is defined as (Cheng and Yeh Citation1980):
The solidity of screen filter used in our experiments is equal to 0.288. As shown in (b), the screen filter is modeled for CFD simulations by placing two perpendicular cylinders with the diameter of 37.5 μm and a gap of 27 μm between those two cylinders to satisfy the solidity of 0.288 following Wang et al.'s (Citation2011a) approach. We used commercial CFD code FLUENT solver (steady-state and laminar flow) to obtain the flow field and particle trajectories around the screen mesh filter. For simulation of the flow field, boundary conditions are face velocity 5 cm/s at inlet, symmetry on four sides of the domain and outflow at outlet. We checked the grid convergence by comparing pressure drop Δp after passing by the screen mesh filter from CFD simulations with that from experimental model by Davies (Citation1973):
Mechanism of particle capture from filter is inertial impaction, diffusion, interception and interception of diffusing particles. Particle collection by diffusion can be obtained by solving the convection diffusion equation defined as:
FIG. 7 Particle trajectories of silver nanowire (dm = 200 nm): (a) PDR with Brownian diffusion and (b) PR without Brownian diffusion.
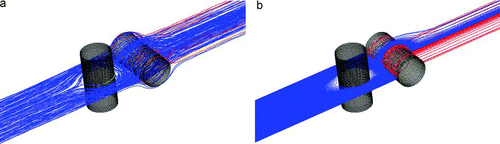
Particle collection by inertial impaction, interception, and interception of diffusing particles can be obtained from discrete phase model (DPM) by solving equation of the particle motion defined as:
As earlier mentioned, the silver nanowires in the range of dm = 200–400 nm under our experimental condition are fully aligned with the electrical field inside DMA. However, in the filter section, the silver nanowire can freely rotate. Thus, following Wang et al.'s (Citation2011a) approach the drag force in the filter section should be recalculated considering the effective dynamic shape factor of silver nanowires. The effective dynamic shape factor x is defined as (Li et al. Citation2012):


By considering particle length in user-defined function (Wang and Pui Citation2009), interception effect can be taken into account. Wang et al. (Citation2011a) obtained the theoretical value of θ′. equal to 40°. But, in Wang and Pui Citation2009, Wang et al.'s (Citation2011a) study, the orientation angle θ′ was not considered in numerical simulations. On the other hand, in our study, the particle length in DPM trajectories is assumed to be Lw sin θ′. We uniformly injected 22,500 particles over the inlet surface. The inertial impaction effect was found to be negligible. The total penetration obtained from numerical simulations, P nu is defined as (Hinds 1999):
6. RESULTS AND DISCUSSIONS
While silver nanowires pass through the screen mesh filter, the distance between filter wire and silver nanowire can be affected by the orientation angle θ′ in the filter section. In other words, the penetration characteristic of silver nanowires can be affected by the orientation angle . Thus, it is important to determine the orientation angle for the silver nanowire. For determination of orientation angle, the relation between the time to pass 1 layer of screen filter (Tt
) and the particle rotation period (Tr
) is compared. Tt
and Tr
are defined by approximating the silver nanowires using ellipsoids and given by the formula (Jeffery Citation1922):
TABLE 2 The orientation angle depending on the number of layers (obtained by fitting the experimental data)
TABLE 3 The penetration of silver nanowire by theoretical and numerical method according to deposition mechanisms
We obtained the penetration of nanowires through the 1 layer of screen mesh filter with theoretical prediction and numerical simulations with the orientation angle 40°. Penetration results for 1 layer of screen mesh filter from theoretical prediction, numerical simulations, and experiments are plotted together in . shows that the magnitude of penetration does not decrease as mobility diameter increases similar to Wang et al. (Citation2011a). It is expected that as mobility diameter increases, the value of PR decreases in both theoretical and numerical predictions because the magnitude of interception parameter increases with the length of nanowires. However, for silver nanowires used in our experiments, PR through theoretical and numerical methods decreases only by 0.1–0.2% as the mobility diameter increases by 50 nm like . It is because the average length of silver nanowires increases only by 300 nm as the mobility diameter increases by 50 nm, which is not helpful to advance the interception effect. It should be noted that in both theoretical prediction and numerical simulation results, taking into account of the interception of diffusing particles effect P DR shows a better agreement with experimental results compared to when P DR is not considered.
7. CONCLUSIONS
We carried out theoretical, numerical and experimental study related to fibrous particle filtration by using the silver nanowires, which are more favorable for evaluating the filtration theory for cylindrical particles than CNTs because the shape of silver nanowire is not bent and curled but stiff and straight. In experiments, we measured the penetration of silver nanowires in the size range of dm = 200 to 400 nm through screen mesh filter. According to Li et al.'s (Citation2012) theory, silver nanowires inside DMA were found to be fully aligned to the electric field and the dynamic shape factor for silver nanowires was obtained. In case of 1 layer of screen mesh filter, we obtained the orientation angle between the filter wire and the particle by fitting experimental results into filtration theory and the value is very close to 40°, which is the average orientation angle Wang et al. (Citation2011a) theoretically predicted. Thus, in theoretical prediction and numerical simulation for the 1 layer of screen mesh filter, the orientation angle is assumed to be equal to 40°. In our numerical simulations, the interception of diffusing particles was also investigated in addition to diffusion, impaction, and interception. From numerical simulation results, it was found that impaction is negligible. Overall, theoretical and numerical predictions by filtration mechanisms including inertial interception, diffusion, and interception of diffusing particles with the orientation angle 40° agree very well with experimental results. For multiple layers of the screen mesh filter, any tendency regarding orientation angle was not found and this tendency would be proved out by further study.
FUNDING
This research was supported by the Basic Science Research Program through the National Research Foundation of Korea (NRF) funded by the Ministry of Science, ICT and Future Planning (2011-0014649).
REFERENCES
- Bahk , Y. K. , Buha , J. and Wang , J. 2013 . Determination of Geometrical Length of Airborne Carbon Nanotubes by Electron Microscopy, Model Calculation, and Filtration Method . Aerosol Sci. Technol. , 47 ( 7 ) : 776 – 784 .
- Cheng , Y. S. , Holmes , T. D. and Fan , B. 2006 . Evaluation of Respirator Filters for Asbestos Fibers . J. Occupat. Environ. Hyg. , 3 ( 1 ) : 26 – 35 .
- Cheng , Y. S. and Yeh , H. C. 1980 . Theory of a Screen-Type Diffusion Battery . J. Aerosol Sci., , 11 ( 3 ) : 313 – 320 .
- Davies , C. N. , ed. 1973 . Air Filtration , 36 London : Academic Press .
- Hinds , W. C. 1999 . Aerosol Technology: Properties, Behavior, and Measurement of Airborne Particles , 190 – 195 . New York and Chichester : Wiley .
- Jeffery , G. B. 1922 . The Motion of Ellipsoidal Particles Immersed in a Viscous Fluid . Proc. R. Soc. A. , 102 ( 715 ) : 161 – 179 .
- Ji , Z. , Wang , X. , Zhang , H. , Lin , S. , Meng , H. , Sun , B. and Zink , J. I. 2012 . Designed Synthesis of CeO2 Nanorods and Nanowires for Studying Toxicological Effects of High Aspect Ratio Nanomaterials . Acs Nano , 6 ( 6 ) : 5366 – 5380 .
- Kim , S. C. , Wang , J. , Emery , M. S. , Shin , W. G. , Mulholland , G. W. and Pui , D. Y. H. 2009 . Structural Property Effect of Nanoparticle Agglomerates on Particle Penetration Through Fibrous Filter . Aerosol Sci. Technol. , 43 ( 4 ) : 344 – 355 .
- Knutson , E. O. and Whitby , K. T. 1975 . Aerosol Classification by Electric Mobility: Apparatus, Theory, and Applications . J. Aerosol Sci., , 6 ( 6 ) : 443 – 451 .
- Li , M. , Mulholland , G. W. and Zachariah , M. R. 2012 . The Effect of Orientation on the Mobility and Dynamic Shape Factor of Charged Axially Symmetric Particles in an Electric Field . Aerosol Sci. Technol., , 46 ( 9 ) : 1035 – 1044 .
- Li , M. , You , R. , Mulholland , G. W. and Zachariah , M. R. 2013 . Evaluating the Mobility of Nanorods in Electric Fields . Aerosol Sci. Technol., , 47 ( 10 ) : 1101 – 1107 .
- Liu , J. , Pui , D. Y. H. and Wang , J. 2011 . Removal of Airborne Nanoparticles by Membrane Coated Filters . Sci. Total Environ., , 409 ( 22 ) : 4868 – 4874 .
- Podgorski , A. , Maißer , A. , Szymanski , W. W. , Jackiewicz , A. and Gradon , L. 2011 . Penetration of Monodisperse, Singly Charged Nanoparticles through Polydisperse Fibrous Filters . Aerosol Sci. Technol., , 45 ( 2 ) : 215 – 233 .
- Seto , T. , Furukawa , T. , Otani , Y. , Uchida , K. and Endo , S. 2010 . Filtration of Multi-Walled Carbon Nanotube Aerosol by Fibrous Filters . Aerosol Sci. Technol., , 44 ( 9 ) : 734 – 740 .
- Vallero , D. A. , Kominsky , J. R. , Beard , M. E. and Crankshaw , O. S. 2008 . Efficiency of Sampling and Analysis of Asbestos Fibers on Filter Media: Implications for Exposure Assessment . J. Occupat. Environ. Hyg., , 6 ( 1 ) : 62 – 72 .
- Wang , J. , Chen , D. R. and Pui , D. Y. H. 2007 . Modeling of Filtration Efficiency of Nanoparticles in Standard Filter Media . J. Nanopart. Res. , 9 ( 1 ) : 109 – 115 .
- Wang , J. , Kim , S. C. and Pui , D. Y. H. 2011a . Carbon Nanotube Penetration Through a Screen Filter: Numerical Modeling and Comparison with Experiments . Aerosol Sci. Technol., , 45 ( 3 ) : 443 – 452 .
- Wang , J. , Kim , S. C. and Pui , D. Y. H. 2011b . Measurement of Multi-Wall Carbon Nanotube Penetration Through a Screen Filter and Single-Fiber Analysis . J. Nanopart. Res. , 13 ( 10 ) : 4565 – 4573 .
- Wang , J. and Pui , D. Y. H. 2009 . Filtration of Aerosol Particles by Elliptical Fibers: A Numerical Study . J. Nanopart. Res. , 11 ( 1 ) : 185 – 196 .
- Wang , J. and Pui , D. Y. H. 2013 . Dispersion and Filtration of Carbon Nanotubes (CNTs) and Measurement of Nanoparticle Agglomerates in Diesel Exhaust . Chem. Eng. Sci., , 85 : 69 – 76 .