Abstract
The climate-change and environmental literature, including that on aerosols, is replete with mention of black carbon (BC) and soot. The terms are used interchangeably in much of the literature, although BC and soot commonly have operational and source-based definitions, respectively, and reliable reference samples and aerosol standards do not exist for either one. The uncertainty about their exact chemical nature and properties can be decreased by materials-based measurement techniques and terminology. Here, we discuss ambiguities in common uses of BC and soot and propose the term ns-soot, where “ns” refers to carbon nanospheres, for a characteristic constituent of BC and soot. Based on its composition, morphology, and structure, we define ns-soot as particles that consist of nanospheres, typically with diameters <100 nm, that possess distinct structures of concentrically wrapped, graphene-like layers of carbon and with grape-like (aciniform) morphologies. We additionally propose that, because of their importance for climate modeling and health issues, distinctions are made among bare, coated, and embedded ns-soot particles.
Copyright 2014 American Association for Aerosol Research
1. INTRODUCTION
Carbonaceous aerosol particles are important for climate, visibility, and health. They are major contributors to light absorption and thus radiative heating of the atmosphere. Many papers discuss the various types of such particles (Bond et al. Citation2013; Petzold et al. Citation2013), the terminology of which is complex and largely based on either measurement methods or particle origins (, which appears at the end of the article). In this note, we propose that a material-based definition of light-absorbing carbonaceous particle types and improved terminology can be established based on probing fundamental physical structures of individual aerosol particles by using the results of transmission electron microscopy (TEM).
TABLE 1 Methods for measuring the concentrations or properties of ns-soot and BC
In the present study,Footnote we focus on major forms of light-absorbing carbonaceous aerosol particles that are commonly called black carbon (BC) or soot. These terms have uneven usage and different meanings (Pöschl Citation2003, Citation2005; Andreae and Gelencsér Citation2006; Bond and Bergstrom Citation2006). According to Charlson and Heintzenberg (Citation1995), and adopted by the IPCC (Citation2007), the term soot refers to “Particles formed during the quenching of gases at the outer edge of flames of organic vapours, consisting predominantly of carbon, with lesser amounts of oxygen and hydrogen present as carboxyl and phenolic groups and exhibiting an imperfect graphitic structure” and BC is an “Operationally defined aerosol species based on measurement of light absorption and chemical reactivity and/or thermal stability; consists of soot, charcoal and/or possible light absorbing refractory organic matter.” These definitions are based on origin and measurement type (), respectively, rather than on material properties. The most recent IPCC report refers to BC as “a distinct type of carbonaceous material formed from the incomplete combustion of fossil and biomass based fuels under certain conditions” (IPCC Citation2013, p. 595) but without details as to the nature of this “distinct” material.
BC in the atmospheric sciences is the term used for carbonaceous materials that absorb light, commonly determined by the attenuation of light by ensembles of particles collected onto a filter and measured by an aethalometer (Hansen et al. Citation1984), although it is also measured using several other methods (). A widely used approach for measuring BC is, contrary to its name, single particle soot photometry (SP2) (Schwarz et al. Citation2008). There are references in the SP2 literature to “refractory BC” or “rBC,” but even though these measured quantities exclude non-refractory phases, they still do not represent a clearly identifiable material. Although BC brings to mind a material, and many aerosol papers refer to it as if it were a specific material, laboratory samples of atmospheric BC that can be measured do not exist. In the absence of an accepted BC standard, surrogate materials such as fullerene, graphite, and Aquadag are used as standards (Gysel et al. Citation2011; Kondo et al. Citation2011; Baumgardner et al. Citation2012; Laborde et al. Citation2012).
Soot, on the other hand, according to wide usage in combustion research, is carbon-rich material that condenses from the vapor phase during combustion (; Ishiguro et al. Citation1997; Richter and Howard Citation2000; Watson and Valberg Citation2001). However, no consensus exists in the atmospheric science community about whether soot is a part of BC, BC is a part of soot, or BC is equivalent to soot. The definition used by IPCC indicates that soot is a part of BC. The usage of soot in electron microscopy studies () commonly agrees with that of the IPCC (Adachi et al. Citation2010). In contrast, in other papers soot consists of BC and other combustion-generated carbonaceous materials (Bond and Bergstrom Citation2006; Seinfeld and Pandis Citation2006; Ramanathan and Carmichael Citation2008; Salamanca et al. Citation2012).
FIG. 1. TEM images of ns-soot and carbon nanospheres. (a) An ns-soot particle from NIST Standard Reference Material (SRM) 1650b (diesel particulate matter). Scale bar = 200 nm. The inset shows a schematic image of carbonaceous nanospheres aggregated to form ns-soot. (b) Enlarged image of (a). Scale bar = 20 nm. (c) High-resolution image of ns-soot particle from (b). The lattice fringes of the ns-soot are evident. Scale bar = 5 nm.
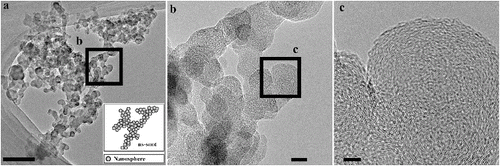
Elemental carbon (EC) is another term used in the aerosol community. EC refers either to the high-temperature fraction of carbonaceous substances determined by using thermal–optical measurements (Pöschl Citation2003, Citation2005) () or to the material that produces characteristic “C cluster ion peaks” in single-particle mass spectra (Prather Citation2012). Numerous methods have been developed and employed to characterize and quantify the light-absorbing carbonaceous particles (). However, since these methods exploit different properties and thus do not necessarily measure the same thing, differences can exist among entities having the same name (Petzold et al. Citation2013). An additional complication is that “the EC fraction is often referred to as soot or BC” (Spencer and Prather Citation2006).
Our goal is to discuss and amplify differences among EC, BC, and soot, which are: a set, category, and broad set of substances that refer, respectively, to the independent concepts of composition, optical properties, and formation process (Schwartz and Lewis Citation2012). We believe that, as a step toward lessening the ambiguity, it would help to provide a material descriptor for an important subset of carbonaceous aerosol particles to which these terms have been applied. Accordingly, using information for determining chemical and physical properties from TEM, we define nanosphere-soot (ns-soot) as a major carbonaceous aerosol particle type that is a specific, well-defined material and compare it to the terms at the beginning of this paragraph. Additionally, we aim to provide new information about the detailed molecular/crystallographic structure of ns-soot.
2. NS-SOOT
As pointed out above, the term soot is used either for combustion products as a group or for a material with specific properties (Richter and Howard Citation2000). TEM has been widely used for studying this material with specific properties (). Although there is extensive mention of soot in TEM aerosol studies (Palotás et al. Citation1996; Ishiguro et al. Citation1997; Buseck and Schwartz Citation2003; Li et al. Citation2003; Wentzel et al. Citation2003; Patel et al. Citation2012), we believe it is appropriate to draw a clear distinction based on physical features rather than origin or optical properties for at least this one important type of aerosol particle. To provide greater specificity, reduce ambiguity, and to distinguish “soot” from BC, EC, and other terms that are commonly used as synonyms, we propose the term “ns-soot” for particles with grape-like (aciniform; Medalia and Rivin Citation1982) morphologies that consist of nanospheres that possess distinct internal structures of concentrically wrapped, graphene-like layers of carbon.
Based on TEM examinations of a large number of aerosol samples from across the globe, ns-soot is a widespread and readily identifiable aerosol type. For example, using TEM we found that in polluted air from Mexico City, ns-soot occurred in 62% of all particles with aerodynamic diameters between 50 and 300 nm (Adachi and Buseck Citation2008), and similar abundances were observed in Phoenix (Katrinak et al. Citation1993), Los Angeles (Adachi and Buseck Citation2013), and China (Li et al. Citation2010). In relatively clean air in Mexico and in the remote marine troposphere above the Southern Ocean, ns-soot occurred in 35% and between 10% and 45% of analyzed accumulation-mode particles, respectively (Pósfai et al. Citation1999; Adachi and Buseck Citation2008). In biomass burning smoke, the relative abundance of ns-soot ranged from 7% to 33%, depending on the type (flaming or smoldering) and age of the smoke (Abel et al. Citation2003; Pósfai et al. Citation2003; Adachi and Buseck Citation2011).
Regardless of source, from fewer than 10 to several hundred nanospheres typically occur in ns-soot particles in the atmosphere. For example, ns-soot from East Asia had 139 nanospheres on average (range: 7 to 509) and that from Arizona traffic had 203 (range: 47 to 792; Adachi et al. Citation2007). Samples from Mexico City had 40 ± 32 nanospheres in ns-soot (Adachi et al. Citation2010).
By considering the individual carbon nanospheres and understanding their chemical and physical characteristics, we can discuss the internal (crystallographic) structure and fundamental optical, chemical, and thermal properties of the basic building blocks of atmospheric ns-soot as a material. High-resolution TEM images of carbon nanospheres, also called “primary particles” or “monomers” in the literature (Vander Wal et al. Citation1999; Liu et al. Citation2008), show the poorly ordered, concentrically wrapped, wavy or curved graphene-like layers (). The irregular stacking of these layers has been called turbostratic (Palotás et al. Citation1996), consistent with the non-uniform stacking that is common in sheet silicate minerals. Such carbon structures, and their electron energy-loss spectrometry (EELS) signals (), indicate that carbon nanospheres are composed largely of layered, sp2-bonded carbon that is consistent with the light-absorbing and refractory properties of ns-soot.
The crystallographic structures of solids can be studied using electron diffraction. The imaging function of the TEM can be combined with selected-area electron diffraction (SAED), a technique that uses an aperture in the image plane of the objective lens to limit the area from which the diffracted electrons are collected (Williams and Carter Citation2009). The resulting SAED pattern contains averaged structural information from the selected object, both in the form of distinct intensity maxima (called “reflections”) in the case of crystalline substances and as diffuse scattering from defective crystalline and amorphous materials. If the material consists of randomly oriented small domains that have some periodicity in their structures, then rings of intensity maxima appear in the SAED pattern. The lack of distinct reflections in the SAED patterns from carbon nanospheres of ns-soot indicates their poorly ordered structures. However, three blurred rings typically occur in SAED patterns as a result of short-range (<1 nm) order within and between the graphene-like layers (). The ∼0.36 nm lattice spacing that corresponds to the first ring can be matched to but is larger than the (002) crystal spacing in graphite, the distance between two neighboring graphene layers. The other two observable spacings cannot be readily matched with those of graphite. First-neighbor atomic distances calculated from SAED patterns of carbon nanospheres indicate that the carbon atoms within the graphene-like layers are, on average, closer to one another than in graphite (Kis et al. Citation2006).
FIG. 3. (a) Typical SAED pattern of ns-soot from the emission of diesel engines from three buses that correspond to European Emission Standards Euro0, 3, and 4 (http://en.wikipedia.org/wiki/European_emission_standards). The rings correspond to lattice spacings of (1) ∼0.36, (2) 0.205, and (3) 0.12 nm. (b) Spacings measured in SAED patterns obtained from ns-soot particles (each column in the diagram refers to a distinct particle). The d-value shown for each particle corresponds to the second ring in their SAED patterns (numbered 2 in (a)). The circles above the columns represent the typical diameters of the carbon nanospheres that constitute the ns-soot particles: small (∼20 to 30 nm), medium (∼30 to 50), and large (>50 nm). Two lattice spacings for graphite are also shown by the horizontal lines. They refer to (010) (upper) and (011) graphite spacings, respectively; the measured spacings cluster around the graphite (011) value. No relationship is apparent between measured spacings and the sizes of constituent carbon nanospheres or the make and year of the buses.
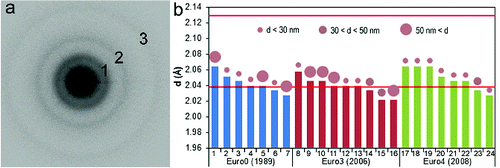
Two types of sizes for ns-soot can be specified: (a) for the individual nanospheres and (b) for the aggregates. The nanosphere diameters are typically between 10 and 100 nm (e.g., ∼44 nm for ambient ns-soot particles from Mexico City [Adachi and Buseck Citation2008] and 32 nm for those from one set of diesel exhaust particles [Park et al. Citation2004]). Geometric sizes or equivalent diameters can be determined for the aggregate particles. The easiest way to determine values is directly from TEM images (Katrinak et al. Citation1993; Adachi and Buseck Citation2008; Adachi et al. Citation2010). If desired, far more accurate three-dimensional (3D) values can be obtained using electron tomography (e.g., 138 ± 50 nm in volume-equivalent diameter for ns-soot particles from Mexico City; Adachi et al. Citation2010).
The basic structural features of carbon nanospheres, including their measurable “lattice spacings” and concentrically layered structures, appear to be independent of their sizes and are not affected by aggregation or mixing with other atmospheric constituents. Interestingly, both the nanosphere sizes and the repeat distances in their structures can vary by ±2% (as measured from the positions of blurred rings in SAED patterns) among ns-soot particles originating even from the same source. Ns-soot particles emitted by different vehicles could not be distinguished on the basis of the sizes and structures of their nanospheres () but differed in the amount of coating material.
Although both the interpretation of fine structure in EELS spectra and the quantitative evaluation of electron diffraction patterns are perhaps beyond the scope of standard TEM users, HRTEM images and SAED patterns (e.g., and , respectively) can be obtained routinely. They provide enough information for the unambiguous identification of ns-soot, which, in turn, is useful for assessing the relative concentration and mixing properties of this important particle type. In contrast, tar balls, which are also spherical, lack distinctive diffraction features and the wavy, graphene-like layers that are characteristic of ns-soot (Pósfai et al. Citation2004; Hand et al. Citation2005; Adachi and Buseck Citation2011). Other organic particles, either of primary or secondary origin, also lack structures that are readily identifiable in TEM measurements (Chen et al. Citation2006; Adachi and Buseck Citation2008). Thus, ns-soot particles can be easily distinguished from other carbonaceous aerosol particles.
3. ASSOCIATED MATERIALS, COATING, EMBEDDING, AND CORE–SHELL STRUCTURES
The term soot has been used inconsistently in the atmospheric and combustion literature. In some instances, it includes the embedding material (Salamanca et al. Citation2012), and in other instances it does not (Virtanen et al. Citation2004). Consistent with our definition of ns-soot above, we propose that only the aggregates of carbon nanospheres should be termed ns-soot, although many ns-soot particles are coated with or embedded within other materials (e.g., organic matter, sulfate), forming internally mixed particles (Adachi et al. Citation2010). Since the coating and embedding materials are chemically and structurally distinct from the ns-soot, they can be readily distinguished from ns-soot in TEM images (). We find this restrictive definition practical since it is independent of whether the mixing of ns-soot with organics, sulfate, or other materials occurred at the time of particle formation or during aging in the atmosphere.
FIG. 4. TEM images of internally mixed ns-soot particles from Mexico showing (a), (b) coating, and (c) embedding, although (b) shows that the distinction is gradational. The ribbon-like features are from the lacey-carbon substrate. Samples (a) and (b) were collected from Mexico City and (c) was collected from biomass burning in Mexico. All samples were collected during the MILAGRO campaign (Adachi and Buseck Citation2008).
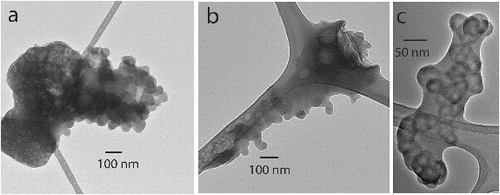
There is a continuous gradation between coated and embedded ns-soot. We propose a distinction such that coated ns-soot has a thin (<1 to ∼10 nm) outer layer of a different material, whereas embedded ns-soot is thickly “coated” or engulfed by another material ( and ). Outlines of embedded ns-soot tend to be smooth and round, whereas those of coated ns-soot tend to be irregular and similar to bare ns-soot.
FIG. 5. Schematic images of (left) ns-soot, (center) internally mixed ns-soot particle, and (right) an ensemble of particles, the total absorption of which would be referred to as from BC when measured with an aethalometer. The aggregated, black layered spherules indicate ns-soot, are evident as faint spherules within the large particle in the center part.
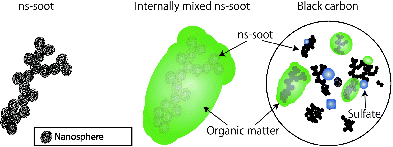
FIG. 6. High-resolution TEM images of (a) bare and (b) coated ns-soot particles from diesel emissions from buses. The arrows in (b) indicate an amorphous coating on carbon nanospheres.
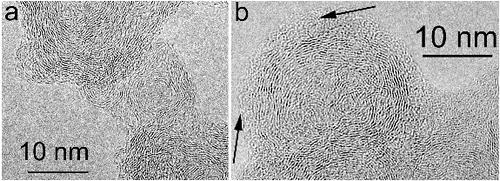
The frequently observed coatings of ns-soot raise the issue of core–shell structures, a concept that has been widely used in the literature because of its utility for Mie scattering calculations (Moteki and Kondo Citation2007). In spite of looking at innumerable individual particles, we have never observed ns-soot with a core–shell structure. Even had ns-soot occurred in the atmosphere within a liquid droplet that subsequently evaporated within the electron microscope, the residue core is not spherical, nor would one expect such a shape for the typical aciniform aggregates.
TEM studies are typically performed under high-vacuum conditions that may result in the loss of volatile components. Thus, one could argue that ns-soot may be a definition for particles that do not even exist in the atmosphere. However, atomic force microscopy (AFM) images obtained from vehicle emissions under ambient conditions clearly show that externally mixed, pure ns-soot particles do occur in the atmosphere, and both uncoated and coated ns-soot particles are emitted, depending on the combustion conditions ().
FIG. 7. Atomic force microscope images of (a) bare, (b) partly coated, and (c) embedded soot particles, all collected from the emissions of vehicles with internal combustion engines.
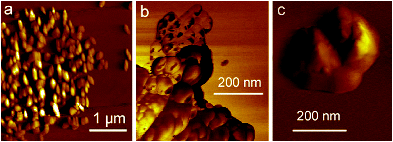
Morphology and the type and extent of internal mixing or embedding of ns-soot can readily be determined using TEM, with the limitation that volatile components remain undetected. Visual scanning is rapid and can be used to select a relatively small number of particles that appear to be representative and, if appropriate, can then be subjected to detailed studies using electron tomography to determine their 3D shapes (van Poppel et al. Citation2005; Adachi et al. Citation2007). Morphology and mixing state strongly influence the scattering cross-sections of ns-soot. For example, the fractal morphology of simulated particles was found to result in a 20 to 28 times enhancement with respect to unaggregated, spherical particles of the same mass (Adachi et al. Citation2007). Detailed information on the 3D morphology also improves the evaluation of the absorption cross-section of ns-soot (Adachi et al. Citation2007) and its enhancement by coating and embedding materials (Adachi et al. Citation2010; Cappa et al. Citation2012; Adachi and Buseck Citation2013).
4. NS-SOOT VS. BC
Although BC (as determined from absorption measurements) is widely used in the literature to refer to distinct particles, in most instances it is applicable to an ensemble of carbonaceous materials that strongly absorb visible light (; ). We suggest that even if BC is assumed to exist as a distinct material, one should speak cautiously of a “BC particle” (Buseck et al. Citation2012) unless the material properties of individual particles have been determined and measured optically—a combination that has not yet been achieved, although Table 1 in Petzold et al. (Citation2013) implies otherwise. BC can contain ns-soot, commonly as a major constituent, but it can also include other light-absorbing carbonaceous materials.
5. UNRESOLVED ISSUES RELATED TO NS-SOOT
Chemical analyses of combustion products are typically of bulk material, whether collected from flames or vehicle tailpipes, rather than the pure ns-soot considered here on both the micro- and nano-size scales. Interpretation of such analyses is a potential source of confusion. Although the dominant component of such bulk material is carbon, it also contains variable amounts of hydrogen, oxygen, and perhaps other elements. It is clear that ns-soot is a major component of this material, but it is less clear whether the elements other than carbon occur (a) within the internal structure of the ns-soot, (b) as coatings on its surfaces, (c) in interstitial areas, or as a combination thereof. This seems a potentially fruitful research subject as analytical developments evolve.
There seems to be a broad consensus in the literature that hydrogen is a component of carbon nanospheres, and both spectroscopic methods and electron diffraction indicate the presence of aromatics (Kis et al. Citation2006; Salamanca et al. Citation2012). However, it is unclear how much of the hydrogen, and perhaps small amounts of other non-carbon elements, are essential parts of the nanospheres or simply adventitious. Further analyses focusing on the carbon nanospheres and utilizing techniques such as TEM and secondary ion-mass spectroscopy (SIMS) are needed.
A related unresolved question is the origin of the curvature of the nanospheres. We assume that the fringes that are evident in high-resolution TEM images (, , and ) are edge-on views of graphene-like layers containing mostly hexagonal rings of carbon. Pentagonal rings cause the curvature of fullerenes and similar materials and presumably occur in the carbon nanospheres in ns-soot. Chemical inhomogeneities can also contribute to the curvature. Hydrogen-bearing aromatics can disrupt the periodicity of graphene, resulting in layers that consist of non-periodically attached, misaligned islands of a few hexagonal rings of carbon (). In the absence of a perfectly periodic crystalline structure, there is no constraint on the morphology so that its layers can readily assume a spherical shape, resulting in the characteristic structure of carbon nanospheres (Lahaye and Prado Citation1981; Pósfai et al. Citation1999).
FIG. 8. (a) Part of Figure 5b, with the contrast of graphene-like layers in ns-soot enhanced digitally. (b) A schematic model for three layers. They are graphene-like, but some C atoms are missing from the hexagonal nets. The missing atoms break the periodic order and presumably cause the misalignment of layer segments relative to one another, resulting in the layer curvature. H in ns-soot can be accommodated around the sites of missing C atoms, where some C atoms can be bonded to two C and one H.
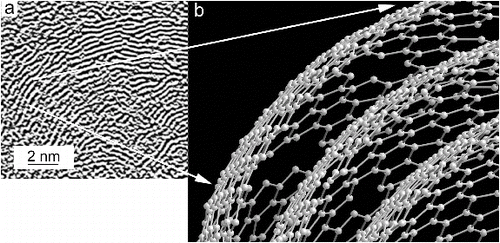
Organic carbon is chemically complex and typically undergoes chemical transformations during atmospheric aging. A contrast is provided by ns-soot. Although there is no evidence of significant chemical or structural changes of individual atmospheric carbon nanospheres as a function of aging or sampling location (Pósfai et al. Citation1999; Li et al. Citation2003; Tumolva et al. Citation2010), the detailed fractal morphology and mixing states of aggregated carbon nanospheres, i.e., ns-soot, varies from source to source and can change upon aging during transport (Abel et al. Citation2003; Adachi and Buseck Citation2013). Such aggregated shapes, which can be characterized using fractal dimensions and mixing states, largely influence the radiative scattering and absorption of ns-soot in the atmosphere (Mackowski Citation1995; Adachi et al. Citation2007).
6. FINAL THOUGHTS
Important knowledge is to be gained by the visualization provided through direct imaging of individual aerosol particles. Of the several types of microscopy, perhaps the most powerful is TEM, which has revealed intimate details of many types of aerosol particles. Although more time consuming and, in some cases, more challenging than some of the other analytical techniques used to study aerosol particles, it nonetheless has provided important new insights that bear on the nature of and relation among ns-soot, BC, and EC and is the basis on which we present the following brief insights. We propose that strongly absorbing carbonaceous species in atmospheric aerosols can be considered as follows.
6.1. Ns-Soot
Carbonaceous particles consisting of nanospheres containing structures consisting of concentrically wrapped, graphene-like layers of carbon and with grape-like (aciniform) morphologies. Ns-soot has specific physical properties and is a distinct and recognizable material. Ns-soot is a convenient term for studies of material characterization and can be used without knowing its sources and optical or thermal properties. For climate modeling and health issues, we recommend distinguishing, when possible, between bare, coated, and embedded ns-soot since each has distinct optical and chemical properties.
6.2. Soot
In our prior studies, we used this term without a precise definition for the particles that we now refer to as ns-soot. Since the definitions of soot vary, we propose to use ns-soot for studies that characterize the particles as materials.
6.3. Black Carbon (BC)
BC absorbs shortwave radiation and thus has distinct optical properties. Although an operational term that is widely used to determine the mass of strongly light-absorbing carbonaceous particles, BC is not well defined as a material.
6.4. Elemental Carbon (EC)
Operational term for both (a) the high-temperature component of thermal–optical analysis and (b) the carbon fragments measured using aerosol mass spectrometry. Since pure EC probably does not exist as such in the atmosphere, the EC of aerosol mass spectrometry likely consists of disrupted graphene-like layers or “carbon clusters” of ns-soot.
ACKNOWLEDGMENTS
The authors acknowledge the use of TEM facilities within the LeRoy Eyring Center for Solid State Science at Arizona State University. We are grateful to R. Flagan, M. Gysel, E. Lewis, P. McMurry, A. Metcalf, K. Prather, S. Schwartz, and anonymous reviewers for helpful comments at various stages of this article.
FUNDING
Kouji Adachi acknowledges support from the global environment research fund of the Japanese Ministry of the Environment (A-1101). Mihály Pósfai acknowledges support from an EU-Hungarian joint grant (TÁMOP 4.2.1/B-09/1/KONV-2010-0003). This study was supported by NSF grants ATM‑0531926 and AGS-1032312.
Notes
1 An earlier version of the article with title “Are black carbon and soot the same?” appears as a discussion paper (Buseck et al. Citation2012).
REFERENCES
- Abel, S. J., Haywood, J. M., Highwood, E. J., Li, J., and Buseck, P. R. (2003). Evolution of Biomass Burning Aerosol Properties from an Agricultural Fire in Southern Africa. Geophys. Res. Lett., 30:1783, doi:10.1029/2003GL017342.
- Adachi, K., and Buseck, P. R. (2008). Internally Mixed Soot, Sulfates, and Organic Matter in Aerosol Particles from Mexico City. Atmos. Chem. Phys., 8:6469–6481.
- Adachi, K., and Buseck, P. R. (2011). Atmospheric Tar Balls from Biomass Burning in Mexico. J. Geophys. Res.-Atmos., 116:D05204, doi:10.1029/2010JD015102.
- Adachi, K., and Buseck, P. R. (2013). Changes of Ns-Soot Mixing States and Shapes in an Urban Area during CalNex. J. Geophys. Res.-Atmos., 118:3723–3730, doi:10.1002/jgrd.50321.
- Adachi, K., Chung, S. H., and Buseck, P. R. (2010). Shapes of Soot Aerosol Particles and Implications for their Effects on Climate. J. Geophys. Res.-Atmos., 115:D15206, doi:10.1029/2009JD012868.
- Adachi, K., Chung, S. H., Friedrich, H., and Buseck, P. R. (2007). Fractal Parameters of Individual Soot Particles Determined Using Electron Tomography: Implications for Optical Properties. J. Geophys. Res.-Atmos., 112:D14202, doi:10.1029/2006JD008296.
- Andreae, M. O., and Gelencsér, A. (2006). Black Carbon or Brown Carbon? The Nature of Light-Absorbing Carbonaceous Aerosols. Atmos. Chem. Phys., 6:3131–3148.
- Apicella, B., Alfe, M., Barbella, R., Tregrossi, A., and Ciajolo, A. (2004). Aromatic Structures of Carbonaceous Materials and Soot Inferred by Spectroscopic Analysis. Carbon, 42:1583–1589.
- Arnott, W. P., Hamasha, K., Moosmüller, H., Sheridan, P. J., and Ogren, J. A. (2005). Towards Aerosol Light-Absorption Measurements with a 7-Wavelength Aethalometer: Evaluation with a Photoacoustic Instrument and 3-Wavelength Nephelometer. Aerosol Sci. Tech., 39:17–29.
- Baumgardner, D., Popovicheva, O., Allan, J., Bernardoni, V., Cao, J., Cavalli, F., Cozic, J., Diapouli, E., Eleftheriadis, K., Genberg, P. J., Gonzalez, C., Gysel, M., John, A., Kirchstetter, T. W., Kuhlbusch, T. A. J., Laborde, M., Lack, D., Müller, T., Niessner, R., Petzold, A., Piazzalunga, A., Putaud, J. P., Schwarz, J., Sheridan, P., Subramanian, R., Swietlicki, E., Valli, G., Vecchi, R., and Viana, M. (2012). Soot Reference Materials for Instrument Calibration and Intercomparisons: A Workshop Summary with Recommendations. Atmos. Meas. Techniq. Discuss., 5:2315–2362.
- Bond, T. C., and Bergstrom, R. W. (2006). Light Absorption by Carbonaceous Particles: An Investigative Review. Aerosol Sci. Tech., 40:27–67.
- Bond, T. C., Doherty, S. J., Fahey, D. W., Forster, P. M., Berntsen, T., DeAngelo, B. J., Flanner, M. G., Ghan, S., K¨archer, B., Koch, D., Kinne, S., Kondo, Y., Quinn, P. K., Sarofim, M. C., Schultz, M. G., Schulz, M., Venkataraman, C., Zhang, H., Zhang, S., Bellouin, N., Guttikunda, S. K., Hopke, P. K., Jacobson, M. Z., Kaiser, J. W., Klimont, Z., Lohmann, U., Schwarz, J. P., Shindell, D., Storelvmo, T., Warren, S. G., and Zender, C. S. (2013). Bounding the Role of Black Carbon in the Climate System: A Scientific Assessment. J. Geophys. Res.-Atmos., 118:5380–5552, doi:10.1002/jgrd.5017.
- Buseck, P. R., Adachi, K., Gelencsér, A., Tompa, É., and Pósfai, M. (2012). Are Black Carbon and Soot the Same? Atmos. Chem. Phys. Discuss., 12:24821–24846. Available at: http://www.atmos-chem-phys-discuss.net/12/24821/2012, doi:10.5194/acpd-12-24821–2012.
- Buseck, P. R., and Schwartz, S. E. (2003). Tropospheric Aerosols, in Treatise on Geochemistry, Vol. 4, K. K. Turekian and H. D. Holland, eds., Elsevier Science Ltd., San Diego, CA, pp. 91–142.
- Cachier, H., Bremond, M. P., and Buat-Menard, P. (1989). Determination of Atmospheric Soot Carbon with a Simple Thermal Method. Tellus B, 41B:379–390.
- Cappa, C. D., Onasch, T. B., Massoli, P., Worsnop, D. R., Bates, T. S., Cross, E. S., Davidovits, P., Hakala, J., Hayden, K. L., Jobson, B. T., Kolesar, K. R., Lack, D. A., Lerner, B. M., Li, S.-M., Mellon, D., Nuaaman, I., Olfert, J. S., Petäjä, T., Quinn, P. K., Song, C., Subramanian, R., Williams, E. J., and Zaveri, R. A. (2012). Radiative Absorption Enhancements due to the Mixing State of Atmospheric Black Carbon. Science, 337:1078–1081.
- Charlson, R. J., and Heintzenberg, J. (eds.) (1995). Aerosol Forcing of Climate. John Wiley & Sons, New York, NY, pp. 91–108.
- Chen, Y. Z., Shah, N., Huggins, F. E., and Huffman, G. P. (2006). Microanalysis of Ambient Particles from Lexington, KY, by Electron Microscopy. Atmos. Environ., 40:651–663.
- Chow, J. C., Watson, J. G., Pritchett, L. C., Pierson, W. R., Frazier, C. A., and Purcell, R. G. (1993). The DRI Thermal-Optical Reflectance Carbon Analysis System - Description, Evaluation and Applications in United States Air Quality Studies. Atmos. Environ., 27A:1185–1201.
- Gysel, M., Laborde, M., Olfert, J. S., Subramanian, R., and Gröhn, A. J. (2011). Effective Density of Aquadag and Fullerene Soot Black Carbon Reference Materials used for SP2 Calibration. Atmos. Meas. Techniq., 4:2851–2858.
- Hand, J. L., Malm, W. C., Laskin, A., Day, D., Lee, T., Wang, C., Carrico, C., Carrillo, J., Cowin, J. P., Collett, Jr., J., and Iedema, M. J. (2005). Optical, Physical, and Chemical Properties of Tar Balls Observed during the Yosemite Aerosol Characterization Study. J. Geophys. Res.-Atmos., 110:D21210, doi:10.1029/2004JD005728.
- Hansen, A. D. A., Rosen, H., and Novakov, T. (1984). The Aethalometer — An Instrument for the Real-Time Measurement of Optical Absorption by Aerosol Particles. Sci. Total Environ., 36:191–196.
- Horvath, H. (1997). Experimental Calibration for Aerosol Light Absorption Measurements Using the Integrating Plate Method – Summary of the Data. J. Aerosol Sci., 28:1149–1161.
- Intergovernmental Panel on Climate Change (IPCC) (2007). Changes in Atmospheric Constituents and in Radiative Forcing, in Climate Change 2007: The Physical Science Basis, Contribution of Working Group I to the Fourth Assessment Report of the Intergovernmental Panel on Climate Change, S. Solomon, D. Qin, M. Manning, Z. Chen, M. Marquis, K. B. Averyt, M. Tignor, and H. L. Miller, eds., Cambridge University Press, Cambridge, U.K. and New York, NY, pp. 129–234.
- Intergovernmental Panel on Climate Change (IPCC) (2013). Clouds and Aerosols, in Climate Change 2013: The Physical Science Basis. Contribution of Working Group I to the Fifth Assessment Report of the Intergovernmental Panel on Climate Change, T. F. Stocker, D. Qin, G.-K. Plattner, M. Tignor, S. K. Allen, J. Boschung, A. Nauels, Y. Xia, V. Bex, and P. M. Midgley, eds., Cambridge University Press, Cambridge, U.K. and New York, NY, pp. 571–657.
- Ishiguro, T., Takatori, Y., and Akihama, K. (1997). Microstructure of Diesel Soot Particles Probed by Electron Microscopy: First Observation of Inner Core and Outer Shell. Combust. Flame, 108:231–234.
- Katrinak, K. A., Rez, P., Perkes, P. R., and Buseck, P. R. (1993). Fractal Geometry of Carbonaceous Aggregates from an Urban Aerosol. Environ. Sci. Technol., 27:539–547.
- Kis, V. K., Pósfai, M., and Lábár, J. L. (2006). Nanostructure of Atmospheric Soot Particles. Atmos. Environ., 40:5533–5542.
- Kondo, Y., Sahu, L., Moteki, N., Khan, F., Takegawa, N., Liu, X., Koike, M., and Miyakawa, T. (2011). Consistency and Traceability of Black Carbon Measurements made by Laser-Induced Incandescence, Thermal-Optical Transmittance, and Filter-Based Photo-Absorption Techniques. Aerosol Sci. Technol., 45:295–312.
- Laborde, M., Mertes, P., Zieger, P., Dommen, J., Baltensperger, U., and Gysel, M. (2012). Sensitivity of the Single Particle Soot Photometer to Different Black Carbon Types. Atmos. Meas. Techniq., 5:1031–1043.
- Lahaye, J., and Prado, G. (1981). Morphology and Internal Structure of Soot and Carbon Blacks, in Particulate Carbon, Formation During Combustion, D. C. Siegla, G. W. Smith, eds., Plenum Press, New York, pp. 33–55.
- Li, J., Pósfai, M., Hobbs, P. V., and Buseck, P. R. (2003). Individual Aerosol Particles from Biomass Burning in Southern Africa: 2. Compositions and Aging of Inorganic Particles. J. Geophys. Res.-Atmos., 108:8484, doi: 10.1029/2002JD002310.
- Li, W. J., Shao, L. Y., and Buseck, P. R. (2010). Haze Types in Beijing and the Influence of Agricultural Biomass Burning. Atmos. Chem. Phys., 10:8119–8130.
- Liu, L., Mishchenko, M. I., and Arnott, W. P. (2008). A Study of Radiative Properties of Fractal Soot Aggregates Using the Superposition T-Matrix Method. J. Quant. Spectrosc. Ra., 109:2656–2663.
- Mackowski, D. W. (1995). Electrostatics Analysis of Radiative Absorption by Sphere Clusters in the Rayleigh Limit: Application to Soot Particles. Appl. Optics, 34:3535–3545.
- Medalia, A. I., and Rivin, D. (1982). Particulate Carbon and Other Components of Soot and Carbon Black. Carbon, 20:481–492.
- Mertes, S., Dippel, B., and Schwarzenböck, A. (2004). Quantification of Graphitic Carbon in Atmospheric Aerosol Particles by Raman Spectroscopy and First Application for the Determination of Mass Absorption Efficiencies. J. Aerosol Sci., 35:347–361.
- Moosmüller, H., Arnott, W. P., and Rogers, C. F. (1997). Methods for Real-Time, In Situ Measurement of Aerosol Light Absorption. J. Air Waste Manage. Assoc., 47:157–166.
- Moteki, N., and Kondo, Y. (2007). Effects of Mixing State on Black Carbon Measurements by Laser-Induced Incandescence. Aerosol Sci. Technol., 41:398–417, doi:10.1080/02786820701199728.
- Murphy, D. M., Cziczo, D. J., Froyd, K. D., Hudson, P. K., Matthew, B. M., Middlebrook, A. M., Peltier, R. E., Sullivan, A., Thomson, D. S., and Weber, R. J. (2006). Single-Particle Mass Spectrometry of Tropospheric Aerosol Particles. J. Geophys. Res.-Atmos., 111:D23S32, doi:10.1029/2006JD007340.
- Novakov, T., and Corrigan, C. E. (1995). Thermal Characterization of Biomass Smoke Particles. Microchim. Acta, 119:157–166.
- Onasch, T. B., Trimborn, A., Fortner, E. C., Jayne, J. T., Kok, G. L., Williams, L. R., Davidovits, P., and Worsnop, D. R. (2012). Soot Particle Aerosol Mass Spectrometer: Development, Validation, and Initial Application. Aerosol Sci. Technol., 46:804–817.
- Palotás, Á. B., Rainey, L. C., Feldermann, C. J., Sarofim, A. F., and Vander Sande, J. B. (1996). Soot Morphology: An Application of Image Analysis in High-Resolution Transmission Electron Microscopy. Microsc. Res. Techniq., 33:266–278.
- Park, K., Kittelson, D. B., and McMurry, P. H. (2004). Structural Properties of Diesel Exhaust Particles Measured by Transmission Electron Microscopy (TEM): Relationships to Particle Mass and Mobility. Aerosol Sci. Technol., 38:881–889.
- Patel, M., Azanza, R., Cristy, L., Scardi, P., and Aswath, P. B. (2012) Morphology, Structure and Chemistry of Extracted Diesel Soot: Part I: Transmission Electron Microscopy, Raman Spectroscopy, X-ray Photoelectron Spectroscopy and Synchrotron X-ray Diffraction Study. Tribol. Int., 52:29–39.
- Petzold, A., Ogren, J. A., Fiebig, M., Laj, P., Li, S.-M., Baltensperger, U., Holzer-Popp, T., Kinne, S., Pappalardo, G., Sugimoto, N., Wehrli, C., Wiedensohler, A., and Zhang, X.-Y. (2013). Recommendations for Reporting “Black Carbon” Measurements. Atmos. Chem. Phys., 13:8365–8379, doi:10.5194/acpd-13-8365-2013.
- Petzold, A., and Schönlinner, M. (2004). Multi-Angle Absorption Photometry – A New Method for the Measurement of Aerosol Light Absorption and Atmospheric Black Carbon. J. Aerosol Sci., 35:421–441.
- Pöschl, U. (2003). Aerosol Particle Analysis: Challenges and Progress. Anal. Bioanal. Chem., 375:30–32.
- Pöschl, U. (2005). Atmospheric Aerosols: Composition, Transformation, Climate and Health Effects. Angew. Chem. Int. Ed., 44:7520–7540.
- Pósfai, M., Anderson, J. R., Buseck, P. R., and Sievering, H. (1999). Soot and Sulfate Aerosol Particles in the Remote Marine Troposphere. J. Geophys. Res.-Atmos., 104:21,685–21,693.
- Pósfai, M., and Buseck, P. R. (2010). Nature and Climate Effects of Individual Tropospheric Aerosol Particles. Annu. Rev. Earth Pl. Sc., 38:17–43, doi:10.1146/annurev.earth.031208.100032.
- Pósfai, M., Gelencsér, A., Simonics, R., Arató, K., Li, J., Hobbs, P. V., and Buseck, P. R. (2004). Atmospheric Tar Balls: Particles from Biomass and Biofuel Burning. J. Geophys. Res.-Atmos., 109:D06213, doi:10.1029/2003JD004169.
- Pósfai, M., Simonics, R., Li, J., Hobbs, P. V., and Buseck, P. R. (2003). Individual Aerosol Particles from Biomass Burning in Southern Africa: 1. Compositions and Size Distributions of Carbonaceous Particles. J. Geophys. Res.-Atmos., 108:8483, doi:10.1029/2002JD002291.
- Prather, K. (2012). Interactive Comment on “Are black carbon and soot the same?” by P. R. Buseck et al. Atmos. Chem. Phys. Discuss., 12:C7311–C7312. www.atmos-chem-phys-discuss.net/12/C7311/2012/.
- Ramanathan, V., and Carmichael, G. (2008). Global and Regional Climate Changes due to Black Carbon. Nat. Geosci., 1:221–227, doi:10.1038/ngeo156.
- Richter, H., and Howard, J. B. (2000). Formation of Polycyclic Aromatic Hydrocarbons and their Growth to Soot —A Review of Chemical Reaction Pathways. Prog. Energ. Combust., 26:565–608.
- Salamanca, M., Mondragón, F., Agudelo, J. R., Benjumea, P., and Santamaría, A. (2012). Variations in the Chemical Composition and Morphology of Soot Induced by the Unsaturation Degree of Biodiesel and a Biodiesel Blend. Combust. Flame, 159:1100–1108.
- Schnaiter, M., Linke, C., Möhler, O., Naumann, K.-H., Saathoff, H., Wagner, R., Schurath, U., and Wehner, B. (2005). Absorption Amplification of Black Carbon Internally Mixed with Secondary Organic Aerosol. J. Geophys. Res.-Atmos., 110:1–11.
- Schwarz, J. P., Gao, R. S., Spackman, J. R., Watts, L. A., Thomson, D. S., Fahey, D. W., Ryerson, T. B., Peischl, J., Holloway, J. S., Trainer, M., Frost, G. J., Baynard, T., Lack, D. A., de Gouw, J. A., Warneke, C., and Del Negro, L. A. (2008). Measurement of the Mixing State, Mass, and Optical Size of Individual Black Carbon Particles in Urban and Biomass Burning Emissions. Geophys. Res. Lett., 35:L13810, doi:10.1029/2008GL033968.
- Schwartz, S. E., and Lewis, E. R. (2012). Interactive Comment on “Are Black Carbon and Soot the Same?” by P. R. Buseck et al.: Disagreement on Proposed Nomenclature. Atmos. Chem. Phys. Discuss., 12:C9099–C9109. www.atmos-chem-phys-discuss.net/12/C9099/2012/.
- Seinfeld, J. H., and Pandis, S. N. (2006). Atmospheric Chemistry and Physics - From Air Pollution to Climate Change. 2nd ed., John Wiley & Sons, New York, NY.
- Smith, D. M., and Chugtai, A. R. (1995). The Surface Structure and Reactivity of Black Carbon. Colloid Surface A, 105:47–77.
- Spencer, M. T., and Prather, K. A. (2006). Using ATOFMS to Determine OC/EC Mass Fractions in Particles. Aerosol Sci. Technol., 40:585–594.
- Sullivan, R. C., and Prather, K. A. (2005). Recent Advances in our Understanding of Atmospheric Chemistry and Climate Made Possible by On-Line Aerosol Analysis Instrumentation. Anal. Chem., 77:3861–3885.
- Tivanski, A. V., Hopkins, R. J., Tyliszczak, T., and Gilles, M. K. (2007). Oxygenated Interface on Biomass Burn Tar Balls Determined by Single Particle Scanning Transmission X-Ray Microscopy. J. Phys. Chem. A, 111:5448–5458.
- Tumolva, L., Park, J.-Y., Kim, J.-S., Miller, A. L., Chow, J. C., Watson, J. G., and Park, K. (2010). Morphological and Elemental Classification of Freshly Emitted Soot Particles and Atmospheric Ultrafine Particles using the TEM/EDS. Aerosol Sci. Technol., 44:202–215, doi:10.1080/02786820903518907.
- Vander Wal, R. L., Ticich, T. M., and Stephens, A. B. (1999). Can Soot Primary Particle Size be Determined Using Laser-Induced Incandescence? Combust. Flame, 116:291–296.
- van Poppel, L. H., Friedrich, H., Spinsby, J., Chung, S. H., Seinfeld, J. H., and Buseck, P. R. (2005). Electron Tomography of Nanoparticle Clusters: Implications for Atmospheric Lifetimes and Radiative Forcing of Soot. Geophys. Res. Lett., 32:L24811, doi:10.1029/2005GL024461.
- Virtanen A. K. K., Ristimäki, J. M., Vaaraslahti, K. M., and Keskinen, J. (2004). Effect of Engine Load on Diesel Soot Particles. Environ. Sci. Technol., 38:2551–2556.
- Watson, A. Y., and Valberg, P. A. (2001). Carbon Black and Soot: Two Different Substances. Am. Ind. Hyg. Assoc. J., 62:218–228.
- Wentzel, M., Gorzawski, H., Naumann, K. H., Saathoff, H., and Weinbruch, S. (2003) Transmission Electron Microscopical and Aerosol Dynamical Characterization of Soot Aerosols. J. Aerosol Sci., 34:1347–1370.
- Wexler, A. S., and Johnston, M. V. (2011). Real-Time Particle Analysis by Mass Spectrometry, in Aerosol Measurement: Principles, Techniques, and Applications, P. Kulkarni, P. A. Baron, K. Willeke, eds., John Wiley & Sons, New York, pp. 233–254.
- Williams, D. B., and Carter, C. B. (2009). Transmission Electron Microscopy: A Textbook for Materials Science. 2nd ed., Springer, New York, NY.