Abstract
A novel monitor for online, in situ measurement of copper (Cu) in ambient fine and ultrafine particulate matter (PM) was developed based on a recent published high flow rate aerosol-into-liquid collector. This aerosol-into-liquid collector operates at 200 L/min flow and collects particles directly as highly concentrated slurry samples. The Cu concentration in slurry samples is subsequently determined by a cupric ion selective electrode (ISE). Laboratory tests were conducted to evaluate the performance of the cupric ISE. The calibration curve of the cupric ISE was determined using Cu(NO3)2 standard solutions prepared by serial dilution. As part of the evaluation, the effects of ionic strength, temperature and pH of the aerosol slurry sample on the cupric ISE measurement were also evaluated. The Cu measurement system performance was evaluated by collecting and measuring samples of lab-generated Cu(NO3)2 aerosols with known mass concentration. Overall, very good agreement between the theoretical and measured Cu concentrations was obtained, corroborating the excellent high overall collection efficiency and measurement accuracy of the Cu measurement system. Field evaluations of the online Cu monitor demonstrated very good agreement for total and water-soluble Cu concentrations with measurements performed by inductively coupled plasma mass spectrometry (ICP-MS), suggesting that interferences from other components of particulate matter are minimal under real world sampling conditions. Moreover, the field tests indicated that the new online Cu monitor could achieve near-continuous collection and measurements (at 2–4 h intervals) for at least 4 to 7 days without any obvious shortcomings in its operation. Both laboratory and field evaluations of the online Cu monitor indicate that it is an effective and valuable technology for PM collection and characterization of Cu in ambient aerosols and provides the foundation for the wider use of ISE for metal analysis and speciation of aerosols.
Copyright 2014 American Association for Aerosol Research
1. INTRODUCTION
Epidemiological and toxicological studies have demonstrated robust associations between ambient particulate matter (PM), especially fine and ultrafine PM, and adverse health outcomes (Pope and Dockery Citation2006; Delfino et al. Citation2011; Li et al. Citation2013). A broadly accepted hypothesis is that PM-induced toxicity is driven by the interaction of PM with cells and macrophages to generate reactive oxygen species (ROS), which change the redox status of the cells (Donaldson et al. Citation2002; Xia et al. Citation2004). There is increasing interest in exploring the emission and concentrations of redox-active metal species, such as Cu and Fe, due to the associations between these species and ROS activities of size-fractioned PM as described in previous studies (Nishida et al. Citation2002; Zheng et al. Citation2006; Shafer et al. Citation2010; Daher et al. Citation2012; Wang et al. Citation2013b). Therefore, measurement of atmospheric metal and elements in high time resolution remains an active topic of aerosol research.
Conventional PM sampling methodologies typically involve collection of particles onto substrates by filtration or inertial impaction. Although widely used and adopted to date, these approaches have many drawbacks, including long turn-around time for processed results of chemical analysis, incomplete extraction of insoluble PM-bound species, as well as potential sampling artifacts for labile species such as semivolatile organic compounds (SVOC). In order to overcome these drawbacks, advanced PM sampling technologies have been developed since 1990s, in which PM is directly collected in liquid phase as suspension samples (Buhr et al. Citation1995; Karlsson et al. Citation1997; Weber et al. Citation2001). These technologies have been well documented in achieving reliable measurements of bulk chemical species such as organic carbon (Sullivan et al. Citation2004) and secondary ions (Orsini et al. Citation2003). However, most of these technologies may be seemingly inadequate for the measurement of metals and trace elements due to their low signal-to-noise ratios. As an alternative technique, a high flow rate aerosol-into-liquid collector was developed to provide concentrated slurries of fine and/or ultrafine PM (Wang et al. Citation2013a). This system operates at a flow rate of 200 L/min and utilizes the saturation-condensation, particle-to-droplet growth component of the Versatile Aerosol Concentration Enrichment System (VACES) (Kim et al. Citation2001a, Citation2001b), growing fine or ultrafine PM to 3–4 μm droplets, in conjunction with a newly designed impactor, in which grown particles are collected gradually forming highly concentrated slurries. In addition, the new aerosol-into-liquid collector is highly adaptable and versatile for potential applications in long-term, in situ measurement of PM chemistry, especially for trace level metal and elements measurement since slurry samples collected by this system are highly concentrated.
Significant progress has been made toward advancing these sampling techniques into fully automated chemical composition measurement instruments (Orsini et al. Citation2003; Sullivan et al. Citation2004). Multiple techniques have been developed for metal and element analysis, such as X-ray fluorescence (Creatchman Citation1999), instrumental neutron activation (Ondov and Kelly Citation1992), and inductively coupled plasma mass spectrometry (ICP-MS) (Montaser Citation1998). Some of these techniques have been commercialized for rapid detection of the ambient aerosol particle's elemental composition. However, these techniques are not without drawbacks. Their application and flexibility are limited by complexities and high costs associated with installation and operation. There is a need for the development of a modular, relative inexpensive, easily operated and maintained platform for online metal and elements measurements. Ion selective electrodes (ISEs) are widely used for determining the concentration of selected ions in aqueous solution (Gupta and D’Arc Citation2000; Schwarz et al. Citation2000). ISEs are generally inexpensive, flexible, and have a high measurement accuracy (errors of less than 3% (Rundle Citation2000)). The usable concentration range over which most commercially available ISEs function can exceed several orders-of-magnitude. Thus, ISEs are potentially valuable instruments for continuous monitoring of selective metal species, especially in providing improved temporal resolution of elemental species concentrations in the atmosphere.
Cu is commonly observed as an abundant metal species in atmospheric PM (Hueglin et al. Citation2005; Pastuszka et al. Citation2010; Cheung et al. Citation2012). It is primarily generated from various vehicular sources including fuel combustion, brake wear, and tire abrasion depending on PM size fractions (Manoli et al. Citation2002; Lin et al. Citation2005; Hjortenkrans et al. Citation2007), as well as copper mining/smelting sources (Prabhakar et al. Citation2014). More importantly, numerous recent studies have demonstrated strong associations between Cu and ROS activities of PM (Nishida et al. Citation2002; Shinyashiki et al. Citation2009; Charrier and Anastasio Citation2012; Daher et al. Citation2012; Wang et al. Citation2013b), suggesting that it may potentially contribute to overall PM-induced toxicity. Therefore, Cu was selected as the primary target metal species in the current study.
This manuscript describes the development of a novel technique for field online measurement of Cu in ambient PM2.5. The novel technique employs the aerosol-into-liquid collector (Wang et al. Citation2013a) as a PM sampling module, in which ambient PM2.5 are directly collected as concentrated slurry samples. Slurries collected in the sampling module are subsequently transferred into the Cu measurement module, in which the water-soluble/total Cu concentration is determined by a cupric ISE. This system can provide accurate and robust measurements of Cu concentration in ambient PM as demonstrated in this article that effectively equivalent data are obtained by the cupric ISE measurements and offline inductively coupled plasma mass spectrometry (ICP-MS) analysis. Moreover, the new system can be operated continuously for at least several days with minimal maintenance or supervision. Both laboratory and field evaluations of this new Cu measurement system suggest that it is an effective and valuable technology for PM collection and characterization of Cu in ambient aerosols.
2. METHODOLOGY
2.1 Ion Selective Electrodes (ISEs)
Ion selective electrodes measure the activity of ions in aqueous solution. Typically an ISE incorporates a membrane, which selectively permits target ions to migrate through it. The potential difference between internal phase of the membrane and a stable reference system is then measured by a high impedance millivolt meter. The relationship between ionic concentration (activity) and the electrode potential is given by the Nernst equation:
[1] where E is the total potential difference (in mV) between the sensing and reference electrodes. E0 is a constant which is characteristic of the particular ISE and n is the charge on the ion. T is the absolute temperature (K). R and F are gas constant and Faraday constant, the values of which are 8.314 J/degree/mole and 96,500 Coulombs, respectively. Log (A) is the logarithm of the activity of the measured ion.
The performance and accuracy of any ISE measurement can be affected by several factors, including ionic strength, temperature, and pH of samples. As discussed previously, the ISE measures activity of ions under an equilibrium condition at the membrane surface. Ion activity is a function of ionic strength. In order to minimize biases between samples (and standards), a buffer is added to both samples and standards to effectively “swamp-out” these biases. The potential difference measured by the ISE is also a function of temperature (refer to the Nernst equation above). Typically, a 1 degree change in temperature will result in 4% change in response for divalent ions such as Cu ions (Rundle Citation2000). Meanwhile, the formation of insoluble Cu(OH)2 limits the pH range over which cupric ISE measurements can be made (when pH is greater than 7), but this is not normally a factor for typical environmental samples, especially if an ionic strength adjuster (ISA) buffer is employed. Other factors such as interfering ions (i.e., Bromide and Silver ions for cupric ISE) may also affect the measurement accuracy of ISEs. However, the levels of these ions in ambient aerosols are well below the threshold at which they may bias the cupric ISE measurements.
2.2. Description of the System and Its Components
The online Cu measurement system utilizes the aerosol-into-liquid collector described previously (Wang et al. Citation2013a) as particle collection module and a cupric ISE as the Cu measurement module. Briefly, in an aerosol-into-liquid collector, the sampled aerosol is first drawn into a saturator tank filled with ultrapure water produced by lab water purification system (Millipore A-10, EMD Millipore, Billerica, MA, USA) and maintained at 30°C, to saturate the air. The particle-vapor mixture then enters two condensation tubes maintained at about −3°C to promote condensational particle growth. Sampled aerosol is cooled to about 21–22°C, and the resulting super saturation grows all particles to droplets in the 3–4 μm range. The grown droplets are then collected in a high flow rate impactor and form concentrated slurry sample. In the impactor, a micro liquid level detector (ML 101, Cosense Inc., Hauppauge, NY, USA) is deployed for automatic measurement of the liquid sample volume, a metric which is essential for converting aqueous PM concentrations to airborne PM concentrations. The volume of slurry sample was determined by converting level distance to the sensor to volume based on the dimension of slurry reservoir of aerosol-into-liquid collector. The collected samples were then transferred to measuring vials (polypropylene, Thermal Scientific, Rockwood, TN, USA) by a computer controlled syringe pump (Model C 3000, 3K step, TriContinent Inc., Grass Valley, CA, USA, using FEP laboratory tubing) with a four-way distribution valve and a 12.5 mL PTFE syringe automatically after each sample collection. Before Cu measurements, cupric ionic strength adjuster (ISA) (0.5 M NaNO3 solution) is transferred into the sample vial and mixed with the sample by a volume ratio of 2%, in order to provide a constant background ionic strength for sample and standards. Sample acidification can be added by mixing the slurry sample with 10% v/v nitric acid (resulting a pH value of 2–3), in order to distinguish water-soluble Cu measurement (without acidification) and total Cu measurement (with acidification). The Cu concentration is determined by a cupric combination ISE (Model 9629BNWP, Thermo Fisher Scientific Inc., Waltham, MA, USA) which is connected to a high impedance millivolt meter (Orion Star A214, Thermo Fisher Scientific, Waltham, MA, USA). The cupric combination ISE has the sensing and reference half-cells built into one electrode. In parallel of the cupric ISE, a stirrer probe (Orion stirrer probe, Thermo Fisher Scientific Inc., Waltham, MA, USA) is deployed to stir the samples thoroughly during Cu measurement. The Cu concentration was measured by the cupric ISE over a period of 5 min. After each measurement, the aerosol-into-liquid collector, the cupric ISE and system sample lines were purged with ultrapure water to remove any carryover from the previous sample. The cupric ISE was maintained in ultrapure water between measurements. The schematic of the system is presented in .
2.3. Laboratory Evaluation of Cupric ISE and System Performance
The first part of laboratory test was the calibration of cupric ISE. The calibration standards (from 10 ppb to 1000 ppb) were prepared by series dilution of 1000 mg/L stock solution that was made in the laboratory using analytical grade salts of cupper (Cu(NO3)2, Fisher Scientific). Before Cu measurement, cupric ionic strength adjuster (ISA) was added to standard solutions by a volume ratio of 2%. Calibrations were performed multiple times to evaluate the consistency of cupric ISE response.
In the second part of laboratory tests, the impact of different factors on cupric ISE measurements, including ionic strength, temperature, and pH of samples were evaluated, as described in previous section of this manuscript. Typically it is suggested that cupric ionic strength adjuster (ISA) should be added into samples before measurements. In order to evaluate the impact of ionic strength, comparison of measurements using and without using ISA were performed by measuring same standard samples with and without adding ISA solution (the volume ratio of adding ISA was 2%). Second, to evaluate the measurement error introduced by changing sample temperature, Cu standard solution of 100 ppb was injected into the sample vial and temperature changes of sample was achieved by heating it in a water bath. The potential difference and standard solution temperature were measured in parallel by cupric ISE and a pH/temperature probe (Orion 9107BNMD, Thermo Fisher Scientific Inc., Waltham, MA, USA), respectively. Standard solutions (i.e., 10 ppb, 100 ppb, 1000 ppb) were acidified to different pH values ranging from 1.7 to 6.8 by adding 1% v/v nitric acid (trace metal grade, VWR) into standard solutions, and measurements were taken by cupric ISE in order to evaluate effect of pH on measurement accuracy. The pH of samples was determined by a pH/temperature probe (Orion 9107BNMD, Thermo Fisher Scientific Inc., Waltham, MA, USA) in parallel with Cu measurements.
Finally the online Cu monitor's performance was evaluated by collecting and measuring samples of lab generated Cu(NO3)2 aerosols with known mass concentration. Cu(NO3)2 particles were generated as test aerosols by atomizing Cu(NO3)2 solutions of known and variable concentrations using a HOPE nebulizer (B&B Medical Technologies, Carlsbad, CA, USA) and were collected directly as slurry samples in the aerosol-into-liquid collector. A small fraction (1.5 L/min) of the aerosols before entering the aerosol-into-liquid collector were diverted first into a diffusion dryer (Model 3062, TSI Inc., Shoreview, MN, USA) and then passed through a scanning mobility particle sizer (SMPS 3936, TSI Inc., Shoreview, MN, USA) coupled with a condensation particle counter (CPC 3022A, TSI Inc., Shoreview, MN, USA) to measure particle size distributions. The mass concentration of generated aerosols was estimated by multiplying the density of Cu(NO3)2 (i.e., 3.05 g/cm3) (Haynes Citation2013) with volume concentration obtained from the number-based particle size distributions, with the assumption of spherical particles. The Cu concentration in the slurry samples was measured by the cupric ISE after every 3–4 h collection (after sufficient volume of slurry was collected for the measurement). The measured Cu concentration was then compared with theoretical Cu concentration, which was calculated by mass concentration of generated aerosol, sampled air volume, and volume of slurry samples, as shown below:
[2]
2.4. Field Evaluation of Cu Measurement System
After completion of the laboratory evaluation, the system described in was deployed at the Particle Instrumentation Unit (PIU) of the University of Southern California for field evaluation tests from December 2013 to March 2014. The ambient atmospheric monitoring site is located in an urban area near downtown Los Angeles, California, within 150 m of a major freeway (I-110), and thus represents a typical urban mix of particles emitted by mostly traffic sources (Ning et al. Citation2007). Ambient PM2.5 samples were collected concurrently using the filter collection, in parallel with the aerosol-into-liquid collector system, in order to evaluate comparability of the novel and traditional sampling methods in terms of PM2.5 elemental composition. To implement the traditional filter sampling comparison, a third condensation tube coupled with a virtual impactor was added to the system and operated in parallel. For filter collection, the 5 L/min minor flow of the virtual impactor (VI) was directly connected to a diffusion dryer (Model 3062, TSI Inc., Shoreview, MN, USA), followed by a 37-mm Teflon filter (Teflo, Pall Corp., Life Sciences, 1-μm pore, Ann Arbor, MI, USA). Detailed information of system configuration was reported in a previous study (Wang et al. Citation2013a). For each set of samples the sampler was operated for 4 h to ensure adequate mass loading (primarily for the offline sample for subsequent comparative analysis). The slurry samples collected by aerosol-into-liquid collector were divided into two portions: in one portion, Cu was measured directly online using the cupric ISE. Measurements were taken before and after acidification of samples in order to acquire both water-soluble and total Cu data. The other portion (together with the parallel filter off-line samples) were stabilized and subsequently analyzed for major and trace elements by magnetic sector ICP-MS. All samples were kept frozen at −20°C after the sampling and prior to chemical analysis.
The slurry samples preserved for chemical analysis were processed as follows: A sub-sample was separated and ultrafiltered at 10 kilo-Dalton (kD) using centrifugal ultrafilters (Amicon Ultra-15 Centrifugal Filter, regenerated cellulose membrane in an all polypropylene device). The elements passing a 10 kD filter can be considered nearly truly dissolved and as such is an ideal fraction to compare with the ISE (which is sensitive only to ions and labile complexes). Another subsample of the slurry was left unfractionated and acid-digested to determine the total mass of chemical species in the PM samples collected by the aerosol-into-liquid collector. This fraction is directly comparable to the ISE data acquired postacidification. Filter samples were sectioned in quarters to provide subsamples for both total and water soluble analyses that paralleled the slurry processing. One section was digested (microwave-aided acid digestion in Teflon bombs) for total elemental analysis. The other filter sections were extracted with water to provide water-soluble samples.
Measurements of major and trace elements were conducted using high-resolution magnetic sector inductively coupled plasma mass spectrometry (SF-ICPMS, Thermo-Finnigan Element 2). The Thermo-Finnigan Element 2 (with fast scanning magnet and Pt guard electrode) was interfaced with a quartz cyclonic spray-chamber fitted with an ESI low-flow (80 μL/min) Teflon microconcentric nebulizer. The complete analytical system is located within a trace metal clean room. Instrumental detection limits (3-sigma) were in the range of 0.01 to 20 ng/L (0.01–20 pg/m3 of air) for most trace elements and in the range of 5 to 50 ng/L (5–50 pg/m3 of air) for major elements. Details of the ICP-MS analysis are presented in a previous study (Zhang et al. Citation2008).
Lastly, the Cu monitor was operated continuously and total Cu concentration was measured for periods ranging between 4–7 consecutive days in March 2014. PM2.5 slurry samples were collected every 4 h, with an approximate slurry accumulation rate of 4–5 mL/h. A one-point standard check (using 100 ppb Cu standard solution) was performed daily to confirm the cupric ISE performance. The sensing surface of the ISE was polished every two days and ISE was recalibrated after each polishing. The trends in ISE-determined Cu concentrations were also referenced to PM2.5 mass concentration data reported by the South Coast Air Quality Management District (AQMD), measured at a monitoring site located approximately 5 km north of our sampling site in downtown Los Angeles during the sampling period.
3. RESULTS AND DISCUSSION
3.1. Calibration of Cupric ISE
The calibration of ISE is normally carried out by immersing cupric ISE in a series of standard solutions with known concentration. Based on the Nernst equation (described in previous section), the potential difference (mV reading) obtained by cupric ISE is linearly correlated with the logarithmic of sample concentration. However, the response of ISE to varying concentrations becomes progressively lower as the concentration decreases below a certain level, resulting in a nonlinear correlation in the lower concentration range (Rundle Citation2000). Calibration results of cupric ISE are presented in . Generally the cupric ISE responds linearly between 50 ppb to 1000 ppb (corresponding to an airborne Cu concentration of 17 to 333 ng/m3), with a R2 value of 0.92, while response becomes nonlinear in the concentration range of 10–60 ppb, thus a more detailed calibration curve needs to be prepared separately at this lower range. Previous studies in the Los Angeles Basin have reported that the average Cu concentration in PM2.5 varies between 18.5–43.1 ng/m3 (Hu et al. Citation2008; Daher et al. Citation2011; Wang et al. Citation2013a,b), corresponding to a slurry concentration of 60–150 ppb (for lower concentration levels, a separate calibration curve in nonlinear ranges can be applied to achieve accurate measurements), respectively. This Cu concentration level is within the linear response range of the proposed cupric ISE, which ensures the accuracy of Cu measurement. Moreover, the slope of the calibration curve in linear response region is about 25.3 ± 0.1 mV/decade, which agrees with the recommended value of 25–28 mV/decade in the manual of cupric ISE. Multiple calibration tests were performed and the calibration curve was very reproducible, illustrating that the obtained calibration curve is reliable in determining the Cu concentration in real-world PM samples.
FIG. 2. Calibration curve of cupric ISE. The top x-axis represents the Cu concentration in standard solution. The bottom x-axis represents the corresponding airborne Cu concentration based on a sampling flow rate of 200 L/min and a slurry collection rate of 4 mL/h. Error bars represent the standard deviations of multiple calibration tests (at least five tests were performed).
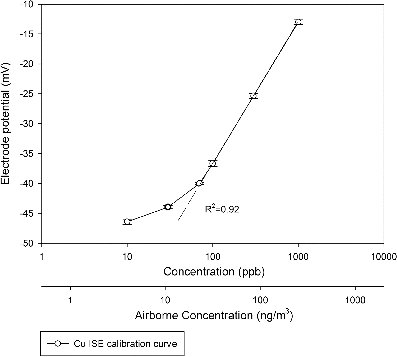
3.2. Effect of Ionic Strength, Temperature, and pH of Sample
As discussed earlier, the ionic strength of measured samples may significantly affect the cupric ISE measurement, especially in high concentration ranges. This is because the interionic interactions (both positive and negative) tend to reduce the mobility of ions thus fewer ions will diffuse through the membrane of ISE, resulting underestimation of real concentration in bulk solution (Rundle Citation2000; Schwarz et al. Citation2000). In most of applications, specific ISA solutions are added before measurements to provide constant ionic strength background. presents the comparison of Cu measurement results between adding/without adding ISA. As shown in , potential differences measure by cupric ISE are consistently lower when ISA are not added, comparing to those obtained after adding ISA. These results indicate that the error of underestimation may occur when ISA is not added to the samples. Moreover, the error introduced by ionic strength is more substantial in higher concentration range (i.e., 6.3% error in 10 ppb standard solution while 34.6% for 1000 ppb standard solution). Thus, adding ISA solution prior to Cu measurement is critical in obtaining accurate measurements, and was followed in all other tests described in this manuscript.
Table 1. Cu measurements showing the necessity of adding ISA
According to the Nernst equation, the potential difference measured by ISE can vary due to changes in temperature. The effect of sample temperature to cupric measurement is presented in . Generally the potential difference obtained by cupric ISE increases linearly (R2 = 0.98) with temperature which has an average slope of 0.53 mV/°C, illustrating that maintaining constant sample temperature is essential in Cu measurements. The temperature of PM2.5 slurry samples collected by aerosol-into-liquid collector are normally about 22.2 ± 0.2°C, resulting in about ±0.1 ∼ ±0.2 mV uncertainties. Therefore, the effect of sample temperature on cupric ISE measurements was neglected in all laboratory tests, as well as in field sample collection and measurements described in the following sections.
FIG. 3. Effect of sample temperature on Cu measurements. Standard solution of 100 ppb was used as sample solution. Error bar represents the standard deviation of multiple reading (three readings for each data point).
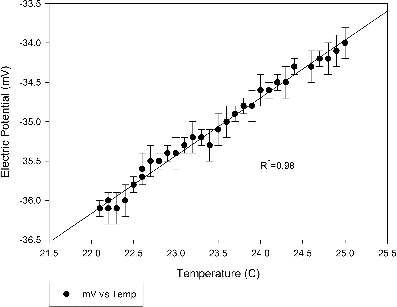
Moreover, the pH of measured sample also has substantial impact on cupric ISE measurements due to the formation of the formation of insoluble Cu(OH)2, which reduces the level of free cupric ion concentration. Results of cupric ISE measurements under different pH values are summarized in . Generally the cupric ISE measurements remained very consistent when sample pH varied between 1.7 and 6.8, indicating the impact of pH on Cu measurement is relatively low in this pH range, especially after sample acidification. The pH of PM2.5 slurry samples collected by aerosol-into-liquid collector is normally about 6.7–7.2. Therefore, the proposed system is capable of measuring both water-soluble Cu (if samples are not acidified), as well as total Cu (if samples are acidified) in ambient PM, an additional advantage compared to other online element monitors.
Table 2. Cupric ISE measurements under different pH values
It is noted that the performance of cupric ISE is also influenced by interference metal species, such as bromide and silver ions. Considering that concentrations of these metal species are usually below detection limit in ambient PM2.5 samples (Hu et al. Citation2008; Saffari et al. Citation2013; Wang et al. Citation2013b), the measurement error introduced by interference species are negligible in the experiments described in this manuscript.
3.3. Collection and Measurement of Lab Generated Cu(NO3)2 Aerosols
In order to demonstrate system collection and measurement performance, Cu(NO3)2 aerosols were generated with known mass concentration (calculated based on particle size distribution and density, as described in previous sections) and subsequently collected as slurry samples in aerosol-into-liquid collector. The Cu concentrations in collected slurry samples were determined by the cupric ISE. Comparison between theoretical and measured Cu concentration is presented in , showing very good agreement between generated and measured Cu concentration, with correlation slope close to 1 and with high R2 value (R2 > 0.9). This agreement is obtained over a concentration range from 100 ppb to over 3000 ppb, indicating an excellent collection efficiency and measurement accuracy of the Cu monitor. It must be noted that by the nature of this study design, the lowest Cu concentration generated in this test is still slightly higher than typical Cu concentration in ambient PM2.5 samples reported previously (i.e., 63–147 ppb). However, samples in this test covered a very wide concentration range while the agreement between generated and measured Cu remains consistent, corroborating the effectiveness of this technology for measuring Cu in ambient PM.
3.4. Field Evaluations
3.4.1. Chemical Results for PM2.5 Samples
present a comparison for total and water-soluble elements between the filter-based collections and the aerosol-into-liquid collector samples. Overall, excellent agreement for most total elements is observed, with a correlation slope close to 1 and with high R2 value (R2 = 0.85). The median concentration ratio of the aerosol-into-liquid collector versus filter across all measured total elements was 1.02 ± 0.12. Similarly for the water-soluble element comparison, very good overall agreement is observed with an average aerosol-into-liquid collector versus filter concentration ratio of 1.08 ± 0.14. Species are also well correlated with R2 = 0.71. A few species (i.e., Br, Pb, etc.) were excluded from these correlations because the measured concentrations were very close to the method detection limit and the associated relative uncertainties were very high. These outcomes are consistent with observations reported in a previous study (Wang et al. Citation2013a) and confirm that the aerosol-into-liquid collector is a robust and versatile tool for aerosol collection with great potential for applications in long-term, in situ measurement of PM chemistry. Moreover, the overall agreement obtained suggests that the aerosol-into-liquid collector is a platform that could be modified to monitor many other metal species in airborne PM.
FIG. 5. Metals and trace elements comparison between filter and slurry samples: (a) total metal and trace elements; (b) water-soluble metal and trace elements. Error bars represent the standard deviation of multiple samples (seven sets of samples). Inserted plot shows the correlations on log-scale.
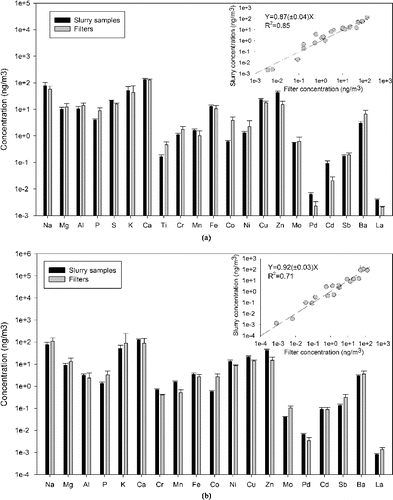
To validate the equivalence between the cupric ISE measurements and the other elemental analysis techniques, a comparison of Cu measurement obtained by the cupric ISE (total after acidification and water-soluble without acidification) and ICP-MS for total and water-soluble Cu was performed (presented in ). Generally very good agreement between cupric ISE and ICP-MS measurements were observed (R2 > 0.87 in both comparisons). Given that ICP-MS measurements can detect some Cu-bound complexes as well, it is generally reasonable that slopes are slightly less than 1. The very good agreement also indicates that there is no evidence of interference from other species on the cupric ISE measurements. In summary, these results indicate that this system is capable of providing Cu measurements that are virtually equivalent to those obtained by ICP-MS for both total and water-soluble portions of ambient PM.
3.4.2. Field Operation of the Online Cu Monitor
The online monitor was deployed at PIU and ambient PM2.5 was continuously sampled and Cu concentration was measured for 4–7 consecutive days (shown in ) to evaluate the system's ability to operate for prolonged periods with minimal supervision. The accumulation rate of the slurry from the aerosol droplets inside the aerosol-into-liquid collector varied from 4 to 6 mL/h, depending on ambient temperature and RH, as well as the ambient particle number concentrations. As shown in , the measured total Cu concentration during the test period varied between 15.3 and 65.5 ng/m3, a range which is generally consistent with PM2.5 Cu concentrations reported in previous studies in the same location (Hu et al. Citation2008; Daher et al. Citation2011; Wang et al. Citation2013a,b). Overall the ISE-determined Cu concentrations have similar diurnal trends as the PM2.5 mass concentration data monitored by AQMD at a nearby site. It is noted that Cu concentrations in PM2.5 may be affected by the local meteorological conditions. During the sampling period, wind speed, and ambient temperature (obtained from the same AQMD site) were highest in the midday (12 PM–4 PM) and afternoon (4 PM–8 PM) periods. This phenomenon may to some degree explain the relative lower Cu concentrations during these time periods comparing to morning (8 AM–12 PM) period, as presented in . In summary, the field measurements data indicate that the online Cu monitor is an effective technology for characterization of Cu in ambient aerosols, capable of prolonged field operation and measurements (4–7 days) with minimal supervision.
4. SUMMARY AND CONCLUSIONS
This study describes the development, laboratory, and field evaluation of a new monitor for online, in situ measurement of total and water-soluble Cu in ambient PM2.5. This novel technique employs the aerosol-into-liquid collector (Wang et al. Citation2013a) to provide concentrated slurry samples of ambient PM2.5. Slurries collected in the sampling module are subsequently measured for water-soluble/total Cu concentration by a cupric ISE. Laboratory Cu(NO3)2 aerosol collection tests demonstrated an excellent system measurement performance. The field experiments demonstrated that proposed system provides Cu concentration measurements that are equivalent to those obtained by ICP-MS for both total and water-soluble components of ambient PM. In addition, the very good agreement obtained for Cu indicates that interferences from other elements in the slurry are minimal. The online Cu monitor could achieve near-continuous measurements (at 2–4 h intervals) for at least 4–7 days without any obvious limitations in its operation and with minimal supervision. Overall, this monitor is versatile with potential applications in long-term, in situ measurement of many other chemical species in ambient PM. Although this study reports only Cu measurements, applications of this system could be extended to other important metal species by deploying different types of ISEs, and eventually provide more comprehensive measurement and evaluation of important redox active metal species in ambient PM.
FUNDING
This study was supported by South Coast Air Quality Management District (AQMD) through award number #11527, and the National Institutes of Health (NIH) through grants R01AI065617-13 and R21AG040753-02 to the University of Southern California (USC). The research described herein has not been subjected to the agency's required peer and policy review and, therefore, does not necessarily reflect the views of the agency, and no official endorsement should be inferred. Mention of trade names or commercial products does not constitute an endorsement or recommendation for use.
REFERENCES
- Buhr, S. M., Burr, M. P., Fehsenfeld, F. C., Holloway, J. S., Karst, U., Norton, R. B., Parrish, D. D., and Sievers, R. E. (1995). Development of a Semi-Continuous Method for the Measurement of Nitric Acid Vapor and Particulate Nitrate and Sulfate. Atmos. Environ., 29:2609–2624. doi: 10.1016/1352-2310(95)00171-T
- Charrier, J. G., and Anastasio, C. (2012). On Dithiothreitol (DTT) as a Measure of Oxidative Potential for Ambient Particles: Evidence for the Importance of Soluble Transition metals. Atmos. Chem. Phys. Discuss., 12:11317–11350. doi: 10.5194/acpd-12-11317-2012
- Cheung, K., Shafer, M. M., Schauer, J. J., and Sioutas, C. (2012). Diurnal Trends in Oxidative Potential of Coarse Particulate Matter in the Los Angeles Basin and Their Relation to Sources and Chemical Composition. Environ. Sci. Technol.. 46:3779–3787. doi: 10.1021/es204211v
- Creatchman, M. (1999). Elemental Analysis of Airborne Particles. CRC Press, Boca Raton, FL.
- Daher, N., Ning, Z., Cho, A. K., Shafer, M., Schauer, J. J., and Sioutas, C. (2011). Comparison of the Chemical and Oxidative Characteristics of Particulate Matter (PM) Collected by Different Methods: Filters, Impactors, and BioSamplers. Aerosol Sci. Technol., 45:1294–1304. doi: 10.1080/02786826.2011.590554
- Daher, N., Ruprecht, A., Invernizzi, G., De Marco, C., Miller-Schulze, J., Heo, J. B., Shafer, M. M., Shelton, B. R., Schauer, J. J., and Sioutas, C. (2012). Characterization, Sources and Redox Activity of Fine and Coarse Particulate Matter in Milan, Italy. Atmos. Environ., 49:130–141. doi: 10.1016/j.atmosenv.2011.12.011
- Delfino, R. J., Gillen, D. L., Tjoa, T., Staimer, N., Polidori, A., Arhami, M., Sioutas, C., and Longhurst, J. (2011). Electrocardiographic ST-Segment Depression and Exposure to Traffic-Related Aerosols in Elderly Subjects with Coronary Artery Disease. Environ. Health Perspect., 119:196–202. doi: 10.1289/ehp.1002372
- Donaldson, K., Brown, D., Clouter, A., Duffin, R., MacNee, W., Renwick, L., Tran, L., and Stone, V. (2002). The Pulmonary Toxicology of Ultrafine Particles. J. Aerosol Med., 15:213–220. doi: 10.1089/089426802320282338
- Gupta, K. C., and D’Arc, M. J. (2000). Performance Evaluation of Copper Ion Selective Electrode Based on Cyanocopolymers. Sens. Actuators B Chem., 62:171–176. doi: 10.1016/S0925-4005(99)00362-7
- Haynes, W. M. (2013). Handbook of Chemistry and Physics, 94th ed. CRC Press, Ann Arbor, MI.
- Hjortenkrans, D. S. T., Bergbäck, B. G., and Häggerud, A. V. (2007). Metal Emissions from Brake Linings and Tires: Case Studies of Stockholm, Sweden 1995/1998 and 2005. Environ. Sci. Technol., 41:5224–5230. doi: 10.1021/es070198o
- Hu, S., Polidori, A., Arhami, M., Shafer, M. M., Schauer, J. J., Cho, A., and Sioutas, C. (2008). Redox Activity and Chemical Speciation of Size Fractioned PM in the Communities of the Los Angeles-Long Beach Harbor. Atmos. Chem. Phys., 8:6439–6451. doi: 10.5194/acp-8-6439-2008
- Hueglin, C., Gehrig, R., Baltensperger, U., Gysel, M., Monn, C., and Vonmont, H. (2005). Chemical Characterisation of PM2.5, PM10 and Coarse Particles at Urban, Near-City and Rural Sites in Switzerland. Atmos. Environ., 39:637–651. doi: 10.1016/j.atmosenv.2004.10.027
- Karlsson, A., Irgum, K., and Hansson, H.-C. (1997). Single-Stage Flowing Liquid Film Impactor for Continuous On-Line Particle Analysis. J. Aerosol Sci., 28:1539–1551. doi: 10.1016/S0021–8502(97)00013–X
- Kidwell, C. B., and Ondov, J. M. (2001). Development and Evaluation of a Prototype System for Collecting Sub-Hourly Ambient Aerosol for Chemical Analysis. Aerosol Sci. Technol., 35:596–601. doi: 10.1080/02786820118049
- Kidwell, C. B., and Ondov, J. M. (2004). Elemental Analysis of Sub-Hourly Ambient Aerosol Collections. Aerosol Sci. Technol., 38:205–218. doi: 10.1080/02786820490261726
- Kim, S., Jaques, P. A., Chang, M., Barone, T., Xiong, C., Friedlander, S. K., and Sioutas, C. (2001a). Versatile Aerosol Concentration Enrichment System (VACES) for Simultaneous in vivo and in vitro Evaluation of Toxic Effects of Ultrafine, Fine and Coarse Ambient Particles Part II: Field Evaluation. J. Aerosol Sci., 32:1299–1314. doi: 10.1016/S0021-8502(01)00058-1
- Kim, S., Jaques, P. A., Chang, M., Froines, J. R., and Sioutas, C. (2001b). Versatile Aerosol Concentration Enrichment System (VACES) for Simultaneous in vivo and in vitro Evaluation of Toxic Effects of Ultrafine, Fine and Coarse Ambient Particles Part I: Development and Laboratory Characterization. J. Aerosol Sci., 32:1281–1297. doi: 10.1016/S0021-8502(01)00057-X
- Li, R., Navab, M., Pakbin, P., Ning, Z., Navab, K., Hough, G., Morgan, T. E., Finch, C. E., Araujo, J. A., Fogelman, A. M., Sioutas, C., and Hsiai, T. (2013). Ambient Ultrafine Particles Alter Lipid Metabolism and HDL Anti-Oxidant Capacity in LDLR-Null Mice. J. Lipid Res., 54:1608–1615. doi: 10.1194/jlr.M035014
- Lin, C.-C., Chen, S.-J., Huang, K.-L., Hwang, W.-I., Chang-Chien, G.-P., and Lin, W.-Y. (2005). Characteristics of Metals in Nano/Ultrafine/Fine/Coarse Particles Collected Beside a Heavily Trafficked Road. Environ. Sci. Technol., 39:8113–8122. doi: 10.1021/es048182a
- Manoli, E., Voutsa, D., and Samara, C. (2002). Chemical Characterization and Source Identification/Apportionment of Fine and Coarse Air Particles in Thessaloniki, Greece. Atmos. Environ., 36:949–961. doi: 10.1016/S1352-2310(01)00486-1
- Montaser, A. (1998). Inductively Coupled Plasma Mass Spectrometry. Wiley, New York.
- Ning, Z., Geller, M. D., Moore, K. F., Sheesley, R., Schauer, J. J. and Sioutas, C., 2007. Daily Variation in Chemical Characteristics of Urban Ultrafine Aerosols and Inference of Their Sources. Environ. Sci. Technol., 41:6000–6006. doi: 10.1021/es070653g
- Nishida, S., Teramoto, K., Kimoto-Kinoshita, S., Tohda, Y., Nakajima, S., Tomura, T. T., and Irimajiri, K. (2002). Change of Cu,Zn-Superoxide Dismutase Activity of Guinea Pig Lung in Experimental Asthma. Free Radic. Res., 36:601–606. doi: 10.1080/10715760210872
- Ondov, J., and Kelly, W. (1992). Tracing Fly Ash Emitted from a Coal-Fired Power Plant with Enriched Rare-Earth Isotopes: An Urban Scale Test. Atmospheric Environ. Part B Urban Atmosphere 26:453–462. doi: 10.1016/0957-1272(92)90052-T
- Orsini, D. A., Ma, Y., Sullivan, A., Sierau, B., Baumann, K., and Weber, R. J. (2003). Refinements to the Particle-Into-Liquid Sampler (PILS) for Ground and Airborne Measurements of Water Soluble Aerosol Composition. Atmos. Environ., 37:1243–1259. doi: 10.1016/S1352-2310(02)01015-4
- Pastuszka, J. S., Rogula-Kozłowska, W., and Zajusz-Zubek, E. (2010). Characterization of PM10 and PM2.5 and Associated Heavy Metals at the Crossroads and Urban Background Site in Zabrze, Upper Silesia, Poland, During the Smog Episodes. Environ. Monit. Assess., 168:613–627. doi: 10.1007/s10661-009-1138-8
- Pope, C. A., and Dockery, D. W. (2006). Health Effects of Fine Particulate Air Pollution: Lines that Connect. J. Air Waste Manag. Assoc., 56:709–742. doi: 10.1080/10473289.2006.10464485
- Prabhakar, G., Sorooshian, A., Toffol, E., Arellano, A. F., and Betterton, E. A. (2014). Spatiotemporal Distribution of Airborne Particulate Metals and Metalloids in a Populated Arid Region. Atmos. Environ., 92:339–347. doi: 10.1016/j.atmosenv.2014.04.044
- Rundle, C. C. (2000). A Beginners Guide to Ion-Selective Electrode Measurements. Nico2000 Ltd Lond.
- Saffari, A., Daher, N., Shafer, M. M., Schauer, J. J., and Sioutas, C. (2013). Seasonal and Spatial Variation of Trace Elements and Metals in Quasi-Ultrafine (PM0.25) Particles in the Los Angeles Metropolitan Area and Characterization of Their Sources. Environ. Pollut., 181:14–23. doi: 10.1016/j.envpol.2013.06.001
- Schwarz, J., Kaden, H., and Pausch, G. (2000). Development of Miniaturized Potentiometric Nitrate- and Ammonium Selective Electrodes for Applications in Water Monitoring. Fresenius J. Anal. Chem., 367:396–398. doi: 10.1007/s002160000367
- Shafer, M. M., Perkins, D. A., Antkiewicz, D. S., Stone, E. A., Quraishi, T. A., and Schauer, J. J. (2010). Reactive Oxygen Species Activity and Chemical Speciation of Size-Fractionated Atmospheric Particulate Matter from Lahore, Pakistan: An Important Role for Transition Metals. J. Environ. Monit., 12:704. doi: 10.1039/b915008k
- Shinyashiki, M., Eiguren-Fernandez, A., Schmitz, D. A., Di Stefano, E., Li, N., Linak, W. P., Cho, S.-H., Froines, J. R., and Cho, A. K. (2009). Electrophilic and Redox Properties of Diesel Exhaust Particles. Environ. Res., 109:239–244. doi: 10.1016/j.envres.2008.12.008
- Sullivan, A. P., Weber, R. J., Clements, A. L., Turner, J. R., Bae, M. S., and Schauer, J. J. (2004). A Method for On-Line Measurement of Water-Soluble Organic Carbon in Ambient Aerosol Particles: Results from an Urban Site. Geophys. Res. Lett., 31:n/a–n/a. doi: 10.1029/2004GL019681
- Wang, D., Pakbin, P., Saffari, A., Shafer, M. M., Schauer, J. J., and Sioutas, C. (2013a). Development and Evaluation of a High-Volume Aerosol-into-Liquid Collector for Fine and Ultrafine Particulate Matter. Aerosol Sci. Technol., 47:1226–1238. doi: 10.1080/02786826.2013.830693
- Wang, D., Pakbin, P., Shafer, M. M., Antkiewicz, D., Schauer, J. J., and Sioutas, C. (2013b). Macrophage Reactive Oxygen Species Activity of Water-Soluble and Water-Insoluble Fractions of Ambient Coarse, PM2.5 and Ultrafine Particulate Matter (PM) in Los Angeles. Atmos. Environ., 77:301–310. doi: 10.1016/j.atmosenv.2013.05.031
- Wang, D., Pakbin, P., Shafer, M. M., Antkiewicz, D., Schauer, J. J., and Sioutas, C. (2013c). Macrophage Reactive Oxygen Species Activity of Water-Soluble and Water-Insoluble Fractions of Ambient Coarse, PM2.5 and Ultrafine Particulate Matter (PM) in Los Angeles. Atmos. Environ., 77:301–310. doi: 10.1016/j.atmosenv.2013.05.031
- Wang, D., Pakbin, P., Shafer, M. M., Antkiewicz, D., Schauer, J. J., and Sioutas, C. (2013d). Macrophage Reactive Oxygen Species Activity of Water-Soluble and Water-Insoluble Fractions of Ambient Coarse, PM2.5 and Ultrafine Particulate Matter (PM) in Los Angeles. Atmos. Environ., 77:301–310. doi: 10.1016/j.atmosenv.2013.05.031
- Weber, R. J., Orsini, D., Daun, Y., Lee, Y.-N., Klotz, P. J., and Brechtel, F. (2001). A Particle-Into-Liquid Collector for Rapid Measurement of Aerosol Bulk Chemical Composition. Aerosol Sci. Technol., 35:718–727. doi: 10.1080/02786820152546761
- Xia, T., Korge, P., Weiss, J. N., Li, N., Venkatesen, M. I., Sioutas, C., and Nel, A. (2004). Quinones and Aromatic Chemical Compounds in Particulate Matter Induce Mitochondrial Dysfunction: Implications for Ultrafine Particle Toxicity. Environ. Health Perspect., 112:1347–1358.
- Zhang, Y., Schauer, J. J., Shafer, M. M., Hannigan, M. P., and Dutton, S. J. (2008). Source Apportionment of in vitro Reactive Oxygen Species Bioassay Activity from Atmospheric Particulate Matter. Environ. Sci. Technol., 42:7502–7509. doi: 10.1021/es800126y
- Zheng, L. - F., Wei, Q. - Y., Cai, Y. - J., Fang, J. - G., Zhou, B., Yang, L., and Liu, Z. - L. (2006). DNA Damage Induced by Resveratrol and Its Synthetic Analogues in the Presence of Cu (II) Ions: Mechanism and Structure-Activity Relationship. Free Radic. Biol. Med., 41:1807–1816. doi: 10.1016/j.freeradbiomed.2006.09.007