Abstract
Three different respirator filter media (two electrets and one fiberglass) were challenged with monodisperse multi-walled carbon nanotubes (MWCNTs) of mobility diameters 20–500 nm at 5.3 and 10.6 cm s−1 face velocities. The penetration data were compared with that of sphere-like NaCl particles. The MWCNT penetrations were generally lower than those of NaCl at both face velocities in all three filters. However, the MWCNTs had a slightly higher penetration than the NaCl in the fiberglass filter at 10.6 cm s−1 face velocity when their mobility diameters were lower than 50 nm and the alignment effect was expected to occur. Results from the scanning electron microscopic (SEM) analysis supported the hypothesis of the alignment effect, which showed that the MWCNTs tend to be straighter or with higher aspect-ratios at the mobility sizes less than 100 nm, leading them more readily to align with the flow. Therefore, caution should be exercised when respirators are used against the MWCNTs with the mobility diameters less than 100 nm. The single fiber theory predicted the penetration of both particles in the fiberglass filters well for the particles with below 100 nm mobility diameters but discrepancies occurred beyond 100 nm. The theory still predicted the NaCl penetration through the electret filters well for the sizes below 100 nm but only predicted the MWCNT penetration well for ∼20–30 nm. The Nuclepore filter and the corresponding capillary tube model were adopted to study the mechanical deposition mechanisms of MWCNTs. The model was found to predict MWCNT penetration very well when the effective length of the MWCNT was taken into account.
Copyright 2014 American Association for Aerosol Research
INTRODUCTION
Carbon nanotubes (CNTs) and carbon nanofibers (CNFs) are remarkable and widely used nanomaterials in commercialized nanotechnology products (NIOSH Citation2013). However, animal studies have shown asbestos-type pathology associated with exposure to long and straight CNTs (Poland et al. Citation2008). NIOSH currently proposed a recommended exposure limit (REL) for CNTs (also for carbon nanofibers, CNFs) of 1 μg m−3 of respirable (<4 μm) elemental carbon as an 8 h time-weighted average (TWA) concentration (NIOSH Citation2013). NIOSH suggested that efforts should be made to reduce the CNT exposure as much as possible because the effects of their shape, size, length, chemistry, functionalization, etc., on their toxicity have not been fully understood (Poland et al. Citation2008; Shvedova et al. Citation2008; Muller et al. Citation2009).
Because of the potential high toxicity of CNTs, EPA has promulgated Significant New Use Rules (SNURs) for premanufacture notice (PMN) substances for the multi-walled carbon nanotubes (MWCNTs, PMN P-08-177), single-walled carbon nanotubes (SWCNTs, PMN P-08-328), and certain multi-walled carbon nanotubes (certain MWCNTs, PMN P-08-199). In the notices, the EPA proposed a series test attempting to reduce their potential serious adverse health effects and environmental impacts (Federal Register Citation2010, Citation2011). Now, EPA further promulgated SNUR for functionalized MWCNTs (PMN P-12-44, Federal Register Citation2013), which could offer an improved dispersion capability for some solution-based nanotechnology applications such as to be the additive for rubber and batteries (Chen et al. Citation1998; Sun et al. Citation2002; Zhang et al. Citation2010). Usually, the functionalization of CNTs (including MWCNTs and SWCNTs) is conducted by attaching certain functional groups (e.g., –COOH and –OH) to their smooth sidewalls and open ends of tubes via harsh oxidative process. One of the mostly used functionalization methods is the nitric acid refluxing in which not only functionalization takes place but also purification is carried out by dissolving and removing the metal catalysts impurities. Such purified CNTs are also widely used in many nanotechnology chemical and biological sensors (Chen et al. Citation2003; Hu et al, Citation2003; Bahk et al. Citation2013). However, the functionalized MWCNTs were found to be more toxic than the pristine (raw) MWCNTs in human T cells toxicity tests (Bottini et al. Citation2006). Besides, Zhao and Liu (Citation2012) indicated that the functional groups can have a negative impact on cell viability. Therefore, in the new SNUR of the functionalized MWCNTs, in addition to the relevant toxicity tests (in vivo and in vitro), the measurements of their size distribution, shape, and length of the functionalized MWCNT were recommended to be the future research focus.
Respirators were suggested to be the final personal protective equipment for handling nanomaterials (NIOSH Citation2009). A NIOSH survey found that 77% carbonaceous nanomaterial companies were using respirator to mitigate the exposure of workers (Dahm et al. Citation2011; Vo and Zhuang Citation2013). However, the performance of filter media was usually determined by challenging with spherical or sphere-like particles such as dioctylpthalate (DOP) and sodium chloride (NaCl) (NIOSH Citation1995; Ozkan and Bradford Citation2013). Therefore, Vo and Zhuang (Citation2013) of NIOSH studied the penetration of pristine MWCNTs aerosolized by a nebulizer through six commercialized facepiece respirators (two of each were NIOSH approved N95, N99, and N100). The authors concluded that the penetration of the elongated MWCNTs was lower than the designated, or the allowed penetration of these respirators. This finding was in agreement with the results for elongated caffeine rods in electret filters (Tuinman Citation1999) and for CNTs in mechanical filters (Seto et al. Citation2010; Wang et al. Citation2011a,Citationb; Wang Citation2013). The reduced penetration was attributed to the higher interception deposition efficiency of the MWCNTs than the sphere-like particles. However, the details of the deposition mechanisms, such as the effects of shape (bending and curling), and length in mechanical filters and electrostatic effect in electret filters, of the MWCNTs have not been investigated.
We performed a mechanistic study for penetration of CNTs through fibrous and electret respirator filter media. The commercial respirator media possess complex structures including polydisperse fiber sizes and random fiber orientations (Bałazy et al. Citation2006, Rengasamy et al. Citation2009); in addition, the electret filter media possess non-uniform charge distributions (Kanaoka et al. Citation1987; Brown Citation1993), thus do not lend themselves easily to detailed studies on deposition mechanisms and accurate modeling. In contrast, filters with homogenous and well-defined micro-structure, such as the Nuclepore filters, can facilitate such studies (Chen et al. Citation2013a). Therefore, we also studied the deposition mechanism of MWCNTs in the Nuclepore filter, which provided complementary information to understand the results of the respirator media.
One of the respirator media was the fiberglass filter, which did not have uniform fibers but the samples of this type had low coefficients of variation and good macroscale homogeneity (Wang et al. Citation2007). The single fiber theory was widely used for predicting particle penetration in the fibrous filtration. However, discrepancies between the prediction and data were commonly observed (Kim et al. Citation2009; Seto et al. Citation2010). For example, Kim et al. (Citation2009) found a discrepancy of ∼15% penetration between the theory and data at most penetrating particle size (MPPS) for silver spheres penetrating through the H&V HE1073 fiberglass filter (Hollingsworth & Vose, East Walpole, MA, USA). The authors believed that microscale inhomogeneity and random orientations of fibers in the filters were important reasons for the discrepancy. Besides, a discrepancy was also found by Seto et al. (Citation2010) in the MWCNT filtration through a fiberglass filter (2.8 μm fiber diameter, 0.049 solidity, and 0.38 mm thickness). The authors introduced orientation angles for the MWCNTs in the theory for fulfilling the comparison but the assumption needed independent verification and the authors suggested investigating the rotation dynamics of the MWCNTs in the filtration with fibrous filters in the future. Therefore, there remain research interests to clarify the reasons for the discrepancy. In comparison, the Nuclepore filters possess more uniform and well-defined microstructures, which can be rather accurately described by a modified capillary tube model (Chen et al. Citation2013a,Citationb). In our recent study, Nuclepore filter was used to study the mechanical (diffusion, interception, and impaction) deposition mechanisms of silver and soot agglomerates, which had a similarly high aspect-ratio shape as the MWCNTs (Chen et al. Citation2013b). The modified capillary tube model predicted the agglomerate penetration through Nuclepore filter very well. Therefore, usage of the Nuclepore filter allows this study to clarify and further understand the mechanical filtration mechanism of MWCNTs. However, so far, there is a lack of study on the electrostatic attraction mechanism of the CNTs in electret filters.
Previous filtration studies on CNTs (Seto et al. Citation2010; Wang et al. Citation2011a,Citationb; Vo and Zhuang Citation2013) or asbestos fiber (Cheng et al. Citation2006) indicated that the major difference between fiber and sphere-like particles was caused by the interception mechanism, for which the CNT length played a significant role. Conversely, the filtration method can be used to determine the CNT length (Bahk et al. Citation2013). Here, we confirmed that the effective length of CNTs can be determined reliably using the filtration method with the Nuclepore filter.
In this study, functionalized MWCNTs by nitric acid refluxing method were produced, aerosolized, and used to challenge two electret and a mechanical fiberglass filter media, which are commonly used in making respirators. The penetrations of the functionalized MWCNTs through these filter media were compared with those of using nearly spherical NaCl to examine the performance of the media. The single fiber theory was used to predict the penetrations of NaCl and MWCNT in both fiberglass and electret filters and compared with the measured penetrations. The mechanical deposition mechanisms of the MWCNTs were also studied experimentally and theoretically using Nuclepore filters and the capillary tube model. The effective lengths, which could be an important parameter of toxicity, of MWCNTs determined by the scanning electron microscope (SEM) analysis and capillary tube model were compared. The final goal is to better understand the filtration mechanisms of functionalized MWCNTs and the level of protection against them provided by regular respirator media.
EXPERIMENT AND THEORY
MWCNTs and Filter Media
Pristine MWCNTs having an outer diameter (tube diameter) of 10–20 nm and average length of 10–30 μm were purchased from Timesnano (China) and functionalized by the nitric acid refluxing method. Functionalized MWCNTs possessed a carboxyl functional group (COOH) and reduced length. The functionalized MWCNTs were prepared with a concentration of 0.002 wt% in water suspension, ultrasonicated for 30 min, and then aerosolized by a homemade nebulizer. The size distribution of the functionalized MWCNTs measured by the Scanning Mobility Particle Sizer (SMPS, Model 3936, TSI Inc., Shoreview, MN, USA) is shown in Figure S1 in the online supplementary information (SI). It is a bimodal distribution with the first mode peaks at around 20 nm, representing the water residues and a certain amount of small MWCNTs. The second mode peaks at around 100 nm, representing the majority of MWCNTs.
Three different respirator media were used for the MWCNT penetration tests. Two of them were electret filter media from 3M (3M Corp., Saint Paul, MN, USA) and the third one was the commercialized HD-2583 fiberglass filter media from H&V (Hollingsworth & Vose Company, East Walpole, MA, USA). The specifications of these filters are shown in . In general, electret filters are designed to reduce the breathing resistance and still to maintain high particle collection efficiency. This can be confirmed by the lower pressure drop at 5 cm s−1 face velocity in electret filters than that in the fiberglass filter as shown in . The electret #1 was highly charged with 7.0×10−5 C m−2 on average, whereas electret #2 had a lower charge of 2.5 × 10−5 C m−2 on average (Li et al. Citation2012a). The three respirator filter media were individually mounted and clamped in a filter holder with 11.3 cm2 filtration area (Particle Size Selector, Model 376060, TSI Inc., Shoreview, MN, USA) and tested for the MWCNT penetration at the face velocities of 5.3 and 10.6 cm s−1, which corresponded to the flow rates of 3.6 and 7.2 L min−1, respectively. The 10.6 cm s−1 is roughly the face velocity of a typical N95 respirator having a surface area of 135 cm2 at 85 L min−1, which is the flow rate for N95 certification (NIOSH 42 CFR Part 84). High efficiency non-woven P100 filters may have a face area of 270 cm2 at 85 L min−1, which gives a face velocity of 5.3 cm s−1. also shows the parameters of the 1 μm pore diameter Nuclepore filter (WHA-111112, GE Healthcare Biosciences, Pittsburgh, PA, USA) that was used to study the mechanical deposition mechanisms of the MWCNTs.
TABLE 1 Specifications of the tested filter media
MWCNT Penetration Tests
shows the experimental setup for the MWCNT generation and penetration tests, in which the atomization and electrostatic classification techniques were used to produce monodisperse MWCNTs (Wang and Tronville Citation2014). Polydisperse MWCNTs were first generated by atomizing 0.002 wt% functionalized MWCNTs solution using the homemade nebulizer as mentioned earlier. Aerosolized MWCNTs were dried by a diffusion dryer and then classified by a Differential Mobility Analyzer (DMA, Model 3081, TSI Inc., Shoreview, MN, USA) to produce monodisperse and singly charged MWCNTs in the size range of 20–500 nm. The valve located upstream of the first Po-210 neutralizer in front of the DMA was used to restrict the aerosol flow rate in order to keep the ratio of sheath to the aerosol flow rate at ∼10. The aerosol flow rate needed to be reduced to 0.5–1.0 L min−1 when the DMA classified particles with sizes larger than 300 nm. The sheath flow rate used was 5.0–10 L min−1. Another Po-210 neutralizer located at downstream of DMA and right in front of the filter holder was used to bring particle charges to Boltzmann equilibrium to avoid unwanted electrostatic effects in the Nuclepore filter (Chen et al. Citation2013a,Citationb). However, the charging distribution should be taken into account in the modeling of particle penetration through electret filters (shown later). By adjusting the valve in front of the system pump (bottom left corner) and the flow mode of the Condensation Particle Counter (CPC, Model 3775, TSI Inc., Shoreview, MN, USA) with low (0.3 L min−1) or high flow (1.5 L min−1), the face velocities from 2 to 30 cm s−1 for the particle penetration tests could be obtained. By switching the three-way valve located in front of the CPC inlet, the experimental particle penetration, Pexp, was obtained by measuring the average particle concentration upstream, Cup, and downstream, Cdown, of the test filter as[1]
FIG. 1. Experimental setup of MWCNT penetration tests for respirator filter media and Nuclepore filter.
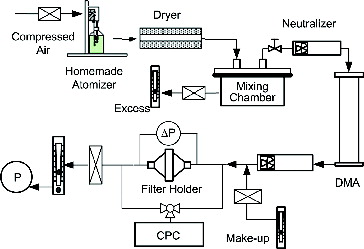
More than five different filter samples for the each electret #1, electret #2, fiberglass, and Nuclepore filter with a total of six penetration measurements for each sample were conducted to obtain the average penetrations. The error bars were calculated, which represented the 95% confidence interval.
Since the DMA was used to classify the polydisperse MWCNTs to monodisperse ones, it was important to know whether the alignment effect occurred for the elongated MWCNTs in the electrical field of DMA. Once it occurred, the monodisperse MWCNTs classified by DMA would not have a correct mobility size as desired. Shorter nanowires (<∼450 nm) with an aspect-ratio of <∼30 and wire diameter of 15 nm could randomly rotate in the electric field of DMA when the sheath flow rate was 10 L min−1 (Kim et al. Citation2007, Li et al. Citation2012b). This finding was in agreement with our previous work (Wang et al. Citation2011b). Besides, we observed the aspect-ratio of large mobility size MWCNTs tended to be significantly reduced due to the bending, curling, and formation of agglomerates (shown later). Therefore, the alignment effect of current MWCNTs in the DMA could be ignored.
SEM Analysis of the Effective Length of MWCNTs
Because the effective length of the MWCNTs could be an important parameter in the filtration tests and might be related to the toxicity in in vivo and in vitro tests, it was determined and measured by drawing a straight line, which is the longest between any two points on each MWCNT in SEM images (JEOL 6500, CharFac, University of Minnesota) with the image-processing software, Image J. No less than 200 monodisperse MWCNTs for each mobility diameter of 50, 100, 150, 200, 300, 400, and 500 nm were examined to obtain reliable length distributions. The obtained average effective length was then used as the interception length in the model for the penetration prediction of the tested filters. One important issue in the SEM analysis was that the projected effective length of the MWCNTs might be affected by a projected angle view by representing a 3D object on a 2D plane. If the end of the MWCNT hit the filter surface and led to a configuration with the MWCNT perpendicular to the surface, the SEM analysis might underestimate the length. To minimize this error, we collected the MWCNTs on the Nuclepore filters with a relatively low face velocity of 2 cm s−1 to minimize their alignment with the flow and reduce the depositions of MWCNTs with orientations close to the perpendicular configuration. Besides, MWCNTs deposited inside the pores with at least 20% of its length away from the filter surface were excluded from the analysis. To determine the aspect-ratio of the MWCNT for the calculation of the bipolar charging distribution for the filtration modeling of electret filters (shown later), the minor axis diameters (Wen et al. Citation1984) were also measured with the same approach as that of the effective length shown above.
Single Fiber Theory
This study investigated the theoretical particle penetration due to mechanical effect of NaCl and MWCNTs through the fiberglass filter using single fiber theory (Wang et al. Citation2007, Citation2011a; Bahk et al. Citation2013). To calculate the efficiency of NaCl and MWCNTs through electret filter, a superposition of mechanical and electrostatic collection effects should be carried out in the model (Lathrache and Fissan Citation1986; Lathrache et al. Citation1986). A detailed discussion of the models and charge distribution for calculating the electrostatic attraction deposition could be found in the SI.
Capillary Tube Model
The capillary tube models developed by Spurny et al. (Citation1969) and Manton (Citation1978, Citation1979) for spherical particle penetration through Nuclepore filters have been modified for applications to soot and Ag agglomerates in our previous works (Chen et al. Citation2013a,Citationb). In the models, the considered mechanisms of particle deposition included the diffusions on filter surface and inside the pore, interception at the pore opening, and impaction on the filter surface. We found that the major differences of the model application between spherical (named Spurny sphere model) and agglomerate (named Spurny agglomerate model) particles were on the interception and impaction, especially on interception, whereas the diffusion deposition did not differ significantly. In the interception model, the effective length should be used as the interception length instead of the mobility diameter to account for the elongated shapes of agglomerates.
In the model of MWCNT penetration through the Nuclepore filter, the effective length of MWCNTs determined by SEM analysis was directly used as the interception length (named Spurny CNT model) without orientation angle. Because the capillary tube was relatively long and the CNT might have enough time to rotate during the time flying through the tube, so the effective length provided the highest possibility for the CNT to be intercepted. The impaction mechanism of the MWCNT was neglected, because previous studies on soot agglomerates showed impaction was negligible under the similar situation (Chen et al. Citation2013b). The details of the modeling can be found elsewhere (Chen et al. Citation2013a,Citationb).
RESULTS AND DISCUSSION
Penetration of MWCNTs in Respirator Filter Media
The depositions of polydisperse MWCNTs with the mobility diameter of 20–500 nm on HD-2583 and 3M #1 filters are shown in Figure S2. To be noted, the deposition amount of MWCNTs in the figure was not indicative of the collection efficiency but meant for qualitative demonstration of characteristics of MWCNTs captured in filters. It can be observed that the functionalized MWCNTs generated in this study were well dispersed. This allowed us to obtain the penetration data of unagglomerated MWCNTs and facilitated the study of MWCNT filtration deposition mechanisms by comparing the data with that from model predictions.
shows the comparison of MWCNT penetration with that of NaCl at 5.3 and 10.6 cm s−1 face velocities in HD-2583 fiberglass (left), 3M electret #1 (middle), and 3M electret #2 (right) filters. The legends in the left-hand-side figure are also for the other two. It can be observed that the MPPSs of the fiberglass filter are larger than that of electret #1 and #2 for both particles, which is in agreement with the results in literature (Kanaoka et al. Citation1987; Bałazy et al. Citation2006; Li et al. Citation2012a; Vo and Zhuang Citation2013). For NaCl particles, the MPPSs were about 120 and 150 nm in the fiberglass, 30 and 40 nm in electret #1, and 40 and 60 nm in electret #2 at 10.6 and 5.3 cm s−1 face velocities, respectively. In each filter, the MPPSs of MWCNTs were about 20–50 nm smaller than that of NaCl, which was expected to be due to the enhancement of interception deposition of MWCNTs. However, a more detailed examination is needed by modeling to quantitatively study the enhanced deposition mechanisms since the penetration differences between the two different types of particles were more pronounced in electret than fiberglass, especially in electret #2. The penetration of MWCNTs was close to that of NaCl at ≦50 nm in the fiberglass, at ∼20 nm in electret #1 and at ≦30 nm in electret #2, where the diffusion dominated size regions were represented. Beyond the diffusion regime, interception dominated the deposition, the penetration of the two types of particles began differing and the difference increased with increasing sizes until the penetrations of both particles went down to near zero. Based on the penetration difference observed in the fiberglass filter, it can be concluded that the penetration difference between the two types of particles in the electret filters was not only due to the enhanced interception deposition of the elongated MWCNTs. Therefore, a more detailed examination is needed by modeling to quantitatively study the enhanced deposition mechanisms since the penetration differences between NaCl and MWCNTs were more pronounced in electret than fiberglass, especially in electret #2.
FIG. 2. Comparison of MWCNT penetrations with that of NaCl obtained by Li et al. (Citation2012a) in the three different respirator filter media.
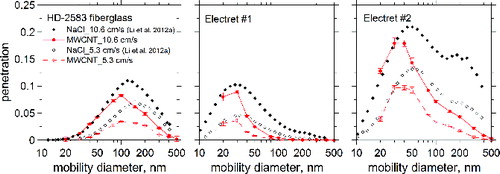
To be noted, the general idea that the elongated CNTs or engineered agglomerates could have a lower penetration than those of spherical or sphere-like particles (Vo and Zhuang Citation2013) and the NIOSH issued standard respirators were applicable to these particles may have exceptions. Because, in , it is observed that the penetration of MWCNTs is equal to or slightly higher than that of NaCl at ≦50 nm in the fiberglass for the 10.6 cm s−1 face velocity. A series of additional experiments and statistical analysis based on Rengasamy et al. (Citation2008) were conducted in order to confirm this observation. Monodisperse NaCl (by atomizing 0.1 wt% NaCl solution) particles and monodisperse MWCNTs with 20, 30, 40, 50, 80, 100, 150, and 200 nm were generated by using the setup shown in , then the penetrations of the two particles through the fiberglass filters were tested. In addition to 10.6 cm s−1, a higher velocity of 30 cm s−1 was also tested for examining the effect of increasing face velocity on the particle penetration. The 30 cm s−1 was chosen, because it is believed that the inhalation face velocity can reach 30 cm s−1 at a heavy work load (Mostofi et al. Citation2010). The comparison of penetrations between the MWCNTs and NaCl is shown in . As shown in in which the sphere-like NaCl had higher penetration than MWCNTs at ≧80 nm, similar results at both 10.6 and 30 cm s−1 velocities were observed in the additional experiments. Surprisingly, the further increase of the face velocity to 30 cm s−1 did not lead to a higher penetration of the MWCNTs than the NaCl for particles larger than 50 nm. For particles ≦50 nm except the 20 nm ones, the average penetrations of the MWCNTs were found to be higher than those of NaCl. The penetrations of the 20 nm particles were very close between the two materials due to the negligible shape effect. Statistical one-way analysis of variance, ANOVA (since particle type is the only one variable) analysis showed that there was a significant (p < 0.005) difference between NaCl and MWCNTs for 30 and 40 nm ones at both 10.6 and 30 cm s−1 face velocities, indicating the MWCNTs did have higher penetrations than NaCl for these two sizes. In comparison, for the 50 nm particle results, there was no significant difference where p value was 0.181 and 0.391 for the 10.6 and 30 cm s−1 face velocities, respectively. The higher MWCNT penetration than the NaCl for these small sizes was attributed to the high aspect-ratio and straight shape of these MWCNTs in the range of several tens of nanometer (shown later), for which the alignment effect was enhanced and the penetration increased. Therefore, more caution should be exercised when the respirators are used for mitigating MWCNTs with mobility diameter equal to or smaller than 50 nm especially when the face velocity is higher than 10.6 cm s−1.
Theoretical Particle Deposition in Fiberglass and Electret Filters
a and b compare the experimental penetration of NaCl and MWCNT particles through HD-2583 fiberglass filter with that predicted by single fiber theory (Equation (S1)) at the face velocities of 5.3 and 10.6 cm s−1, respectively. As can be seen, the theory predicted the penetrations well in the size region of <∼100 nm for both particles, with a slight deviation for 30–50 nm MWCNTs at 10.6 cm s−1 face velocities. For these small sizes, a lower penetration of MWCNTs than that of NaCl existed in the model due to the enhanced interception of MWCNTs with elongated shape, however, the data of the MWCNT presented a higher penetration than the model and also higher than the NaCl data. This was attributed to the effect of alignment. Larger than 100 nm, the theory started overestimating the penetration when the interception mechanism turned to be stronger than the diffusion. The discrepancies between theory and data were getting significant at about 150 nm for MWCNT and 250 nm for NaCl until the interception completely dominated the deposition at mobility size larger than 300 nm for MWCNTs and 500 nm for NaCl particles. To conclude, the agreement between theory and data at small sizes indicated that the theoretical term of diffusion was valid, in comparison; overestimation of penetration by theory in the size range of 100–500 nm revealed that the interception term was not accurate. A similar result of discrepancy between data and theory was found by Kim et al. (Citation2009) in which the penetration of Ag nanoparticle agglomerates through HE1073 fibrous filter was investigated and they attributed the discrepancy to the inhomogeneity and random orientations of the fiberglass filter. In contrast, Wang et al. (Citation2011a) found the theory was able to predict the polystyrene latex (PSL) penetration very well through the woven screen filter that possessed uniform microstructure. Therefore, we believe the discrepancy for the current fiberglass filter could be also due to the filter inhomogeneity and random orientations and the interception term may need some modification to improve the theory for the non-woven fibrous filters. Based on these results, it can be concluded that the single fiber theory does not account for microstructural inhomogeneity thus presents difficulty for one to investigate the deposition mechanisms of both sphere-like NaCl and elongated MWCNTs through fibrous filters; however, the current data may be useful for improving the theory.
FIG. 4. Comparison of experimental data of NaCl and MWCNTs through HD-2583 fiberglass filter with the predictions by single fiber theory at the face velocity of 5.3 (a) and 10.6 cm s−1 (b).
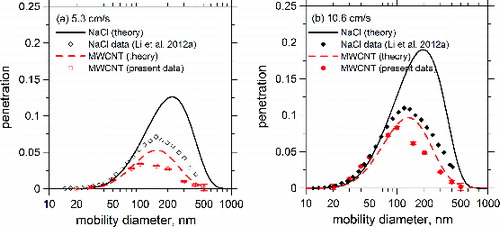
shows the comparison of NaCl particle penetrations in electret filters between data and model (Equation (S16)). The model was first validated by repeating the results of in Lathrache et al. (Citation1986) and a good agreement was obtained. Similar to , also reveals the model predicted the particle penetration well for small sizes (<∼70 nm in electret #2 and <∼150 nm in electret #1), indicating the model predicted a good electrostatic deposition at small sizes. However, beyond the agreement size regions, the model presented a significant second mode consistently for both electret filters at both face velocities. Meanwhile, the data started departing from the curve and a significant overestimation of penetration by model was shown. A significant second mode was not apparent in the experimental penetration curves; nevertheless, the shapes of the curves indicated existence of a minor second mode, most obvious in the experimental curve for electret #2 at 10.6 cm s−1. The bimodal penetration curves with a significant second mode predicted by model or the experimental ones only revealing a minor second mode have been found in the literature (Baumgartner and Löffler, Citation1986; Lathrache et al. Citation1986; Kanaoka et al. Citation1987; Tuinman, Citation1999; Bałazy et al. Citation2006).
FIG. 5. Comparison of NaCl penetration through electret filter between data and model (Lathrache and Fissan Citation1986).
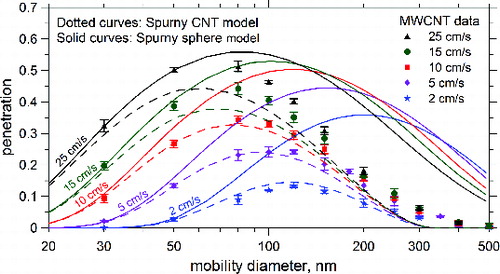
The existence of discrepancies between data and model beyond certain particle sizes for electret filters is similar with that shown for the fiberglass filter in . The model overestimated the NaCl penetration significantly starting right before the saddle point of the bimodal curve. However, Lathrache and Fissan (Citation1986) concluded that the model was able to predict the penetration well for particles with charge equilibrium through electret filter. That was because they only validated the model with data up to 200–300 nm and the comparison for larger particles was not available. Nevertheless, the present model is in better agreement with the data than the model developed by Brown (Citation1981), which would lead to a significant discrepancy between model and data in the whole size range of particles studied (12–410 nm). This study also compared the data by using the model shown in Bałazy et al. (Citation2006). A very good agreement between data and model could be obtained for all particle sizes, similarly to that shown in Figure 6 of Bałazy et al. (Citation2006). However, in the modeling, a manually generated fitting constant B (found to be 5.0 in this study and it was 0.21 in Bałazy et al. Citation2006) was applied to Equation (S14) and the charge density of the filter was a variable and was determined by matching the model calculation to the data rather than directly using the measured charge density. Therefore, there exist limitations of the model application of Bałazy et al. (Citation2006) in other electret filters with different properties.
For the modeling of MWCNT penetration through electret filters, the calculation results are not shown here but qualitative discussion is given in the following. The model also predicted bimodal penetration curves for both face velocities with significant second modes similar to that of NaCl as shown in . The experimental penetrations were only in agreement with the model for 20 and 30 nm mobility diameters and then the model overestimated the penetration significantly beyond 30 nm. The large discrepancy between data and model beyond 30 nm could be due to that Equations (S12)–(S15) were intended for spherical particles. The calculation in Equations (S13) and (S15) using mobility diameter showing significant discrepancy suggested that some other equivalent length related to the electrical property should be developed for MWCNTs. In addition, errors may exist for calculation of the electrical charges on elongated particles such as MWCNTs and open-structured agglomerates (Shin et al. Citation2010). It is to be mentioned, the model (Equation (S1), mechanical effect only) was able to predict the penetrations of MWCNTs through fiberglass well up to ∼100 nm as shown in . Therefore, the model started overestimating the MWCNT penetrations from 30 nm, indicating the electrostatic effect on MWCNT was underestimated from very small sizes. To summarize, although the single fiber theory was not able to predict the penetration very well for elongated MWCNTs through both fiberglass and electret filters, according to the results shown in Figures , , and , both filters were more efficient for MWCNT than NaCl. The electrostatic deposition mechanisms for elongated particles could be very complicated and it is worth to be a future study.
Deposition Mechanisms of MWCNTs in Nuclepore Filters
In order to better understand the mechanical filtration mechanisms of MWCNTs, Nuclepore filters and the corresponding capillary tube model were adopted. shows the comparison of experimental MWCNT penetrations with the theoretical results for both spherical (Spurny sphere model) and MWCNT (Spurny CNT model) particles at different face velocities from 2 to 25 cm s−1. The reason of presenting the penetrations of spherical particles was for comparison, so that more information on filtration dynamics of the MWCNTs could be obtained. In the model, the effective length of MWCNT determined from SEM analysis (shown later) was used as the interception length. The Spurny CNT model predicted the penetrations very well for face velocities at 2, 5, and 10 cm s−1. However, the model underestimated the penetration at 15 and 25 cm s−1, which might be due to the alignment effect and a detailed discussion will be shown in the following. The comparison of the penetration of MWCNTs with that of spheres showed that they are close at only 30 nm when diffusion mechanism dominated the particle collection. The penetration difference increased with increasing particle size from 30 nm to about 200 nm and then decreases slightly to 500 nm. This was due to the enhanced interception deposition of the MWCNTs. It is also seen the penetration of MWCNTs increased with increasing face velocity; at the same time, the most penetrating particle size shifted to smaller particle size.
FIG. 6. Comparison of experimental and theoretical penetrations of MWCNTs in Nuclepore filters at different face velocities. Penetrations of spheres were also shown for comparison purpose with MWCNTs.
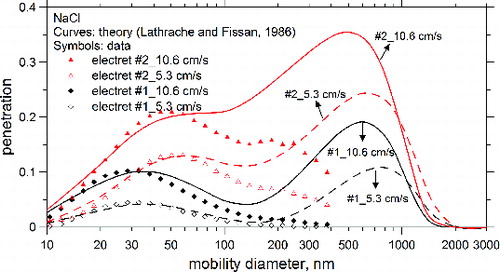
The tested face velocity as high as 15 or 25 cm s−1 was for examining whether the alignment of MWCNTs occurred in the filtration and we did see it occurred. To be noted, alignment effect was not observed for soot and silver agglomerates at the face velocity lower than 7.5 cm s−1 (Chen et al. Citation2013b), therefore, it is expected that the MWCNTs also would not experience the effect at the face velocity lower than 7.5 cm s−1. For instance, the penetration for 50 nm MWCNTs was very close to that of spherical particles at 2 cm s−1, which was attributed to that the diffusion mechanism (97.5% collection efficiency by adding the surface diffusion and diffusion in the pore) dominated the deposition while interception was much weaker. The penetration difference then increased with increasing face velocity at 5 and 10 cm s−1 when diffusion efficiency decreased to 81.4 and 63.2%, respectively, resulting the observation of the penetration difference. As the face velocity was increased further to 15 and 25 cm s−1, the difference was expected to be further increased when the diffusion efficiency was decreased to 52.1 and 41.0%, respectively. However, this trend was not found, instead, the difference became smaller and smaller. This is attributed to the occurrence of alignment. The alignment effect, similar trend as that found for 50 nm MWCNTs, was also observed for 80 and 100 nm MWCNTs. However, a question may be raised why the alignment was not significant for lager size MWCNTs. The reason can be found in that shows representative SEM images of DMA-classified monodisperse MWCNTs with different mobility diameters. It is seen that 50 and 100 nm MWCNTs compared to larger ones possess straighter and higher aspect-ratio structures and therefore were more readily available to align with the flow. In comparison, MWCNTs with mobility diameter of 200 to 500 nm were generally with a higher level of curling, bending, and agglomeration. These larger MWCNTs did not have as high aspect-ratio as smaller ones therefore the alignment effect was not obvious.
Effective Length of Different Mobility Diameter MWCNT
Since the effective length is the crucial parameter on the MWCNT filtration for the tested respirator filter media and Nuclepore filter and it usually dominates the filter deposition as shown earlier, here, we are presenting the SEM analysis result of their effective length as function of mobility diameter to help clarify the MWCNT deposition mechanisms. Figures S3a and b show the effective length distributions of the monodisperse MWCNTs with 50 and 100 nm mobility diameters and those for 150, 200, 300, 400, and 500 nm mobility diameters, respectively. More discussion could be found in the SI.
The mobility diameter and effective length determined by SEM of the MWCNTs had a very good quadratic polynomial correlation with r-squared of 0.997 as[2] Equation (2) was used in the earlier model calculations of MWCNT penetration in fiberglass, electret, and Nuclepore filters. Besides, it may be also used to predict the effective length of the functionalized MWCNTs with mobility diameter of 50–500 nm, which have a pristine tube diameter of about 10–20 nm.
This study also tried to use the filtration method of Nuclepore filter to determine the MWCNT effective length and compared with that of SEM analysis. In the method, the surface and pore diffusions and impaction (neglected) terms were treated as known, the interception length was the only unknown in the model and could be easily calculated when the experimental penetration was used in the modeling. In the modeling, the data of 5 cm s−1 was compared when no alignment effect occurred. Then the obtained interception length was assumed to be equal to the effective length. shows the calculation results of the effective lengths of MWCNTs with different mobility diameters and compares them with the average values determined by the SEM analysis. To be noted, the effective length for 500 nm MWCNTs could not be obtained because the penetration was zero and the effective length could be any value larger than 1000 nm. It is seen the average SEM effective length was very close to that by model with a relative difference of less than 13%. This indicates that the modified capillary tube model is able to predict the penetration of the MWCNTs very well as shown in when no alignment effect occurred. It is to be mentioned again, the effective length should be used as the interception length instead of the mobility diameter for the elongated MWCNTs in the filtration modeling of mechanical filters. Besides, the effective length might be related to the electrical property of MWCNTs in the electrostatic filtration modeling of electret filters.
TABLE 2 Effective length (nm) of different mobility diameter MWCNTs determined from SEM analysis and filtration method
CONCLUSION
Functionalized MWCNTs were produced by the nitric acid refluxing method and aerosolized using a homemade nebulizer. The generated MWCNTs were then used to challenge two electret and one fibrous respirator filter media with face velocities of 5.3 and 10.6 cm s−1 that are usually used in the N95 and P100 respirator tests. SEM analysis was conducted to obtain the effective length of the MWCNTs and to observe their characteristics at different mobility diameters. It is seen the 50 and 100 nm MWCNTs compared to larger diameter ones possess straighter and higher aspect-ratio structures. MWCNTs with 200 to 500 nm were generally with a higher level of curling, bending, and agglomeration. Based on the measured effective length, single fiber theory and the superposition of theoretical electrostatic deposition developed by Lathrache and Fissan (Citation1986) were adopted to predict the MWCNT penetration through the electret and fibrous filters. NaCl penetration data of these filters from Li et al. (Citation2012a) were used to compare the penetration difference with MWCNTs.
Filtration results showed that the MWCNTs generally had lower initial penetrations (clean filter condition) than that of NaCl at the same mobility size, which was due to the longer interception length of the MWCNTs and stronger electrostatic effect in the electret filters. Alignment effect caused by high face velocity (>10 cm s−1) was observed for the MWCNTs in this study. The effect is more significant for 30–50 nm in the respirator filter media and 50–100 nm in the Nuclepore filter and more caution should be exercised when the respirators are used to mitigate ultrafine MWCNTs especially with the face velocity higher than 10 cm s−1. The effective length of the MWCNTs determined by the capillary tube model was close to that obtained from SEM analysis. A good quadratic polynomial correlation between mobility diameter and effective length of the current MWCNTs was found for sizes with 50–500 nm. The effective length was an important parameter for respirator efficiency and might be a key factor for toxicity of the MWCNTs.
The single fiber theory predicted the penetration of both sphere-like NaCl and elongated MWCNT through the fiberglass and electret filters well at small sizes but consistently overestimated the penetration at larger sizes due to the underestimation of interception and electrostatic depositions. The electrostatic effect was significant on the MWCNTs in the electret filters. The current data could be used to improve the single fiber theory on both interception deposition and electrostatic deposition mechanisms in fibrous and electret filters in the future. We investigated successfully the mechanical deposition mechanisms of the MWCNTs by using Nuclepore filters and the modified capillary tube model. The model covered the depositions of diffusion, interception, and impaction, which related the filtration dynamics of the MWCNTs to their mobility diameter, effective length, morphology, and density.
CNT_filtration_mechanism_Supplementary_Information.zip
Download Zip (1.4 MB)ACKNOWLEDGMENTS
The authors thank the support of members of the Center for Filtration Research: 3M Corporation, BASF Corporation, Boeing Commercial Airplanes, Cummins Filtration Inc., Donaldson Company, Inc.; Entegris, Inc.; H.B. Fuller Company; Hollingsworth & Vose Company; MANN+HUMMEL GMBH; MSP Corporation; Samsung Electronics Co., Ltd.; Shigematsu Works Co., Ltd.; TSI Inc.; and W. L. Gore & Associates, Inc., and the affiliate member National Institute for Occupational Safety and Health (NIOSH). Parts of this work were carried out in the Characterization Facility, University of Minnesota, a member of the NSF-funded Materials Research Facilities Network (www.mrfn.org) via the MRSEC program. One of the authors, Heinz Fissan, acknowledges the support by the Institute of Energy and Environmental Technology (IUTA), Duisburg, Germany.
Additional information
Funding
REFERENCES
- Bahk, Y. K., Buha, J., and Wang, J. (2013). Determination of Geometrical Length of Airborne Carbon Nanotubes by Electron Microscopy, Model Calculation and Filtration Method. Aerosol Sci. Technol., 47:776–784.
- Bałazy, A., Toivola, M., Reponen, T., Podgorski, A., Zimmer, A., and Grinshpun, S. A. (2006). Manikin-Based Performance Evaluation of N95 Filtering-Facepiece Respirators Challenged with Nanoparticles. Ann. Occup. Hyg., 50:259–269.
- Baumgartner, H., and Löffler, F. (1986). The Collection Performance of Electret Filters in the Particle Size Range 10 nm-10 μm. J. Aerosol Sci., 17:438–445.
- Bottini, M., Bruckner, S., Nika, K., Bottini, N., Bellucci, S., Magrini, A., et al. (2006). Multi-Walled Carbon Nanotubes Induce T Lymphocyte Apoptosis. Toxicol. Lett., 160:121–126.
- Brown, R. C. (1981). Capture of Dust Particles in Filters by Line-Dipole Charged Fibres. J. Aerosol Sci., 12:349–356.
- Brown, R. C. (1993). Air Filtration. Pergamon Press, London.
- Chen, J., Hamon, M. A., Hu, H., Chen, Y., Rao, A. M., Eklund, P. C., et al. (1998). Solution Properties of Single-Walled Carbon Nanotubes. Science, 282:95–98.
- Chen, R. J., Bangsaruntip, S., Drouvalakis, K. A., Kam, N. W. S., Shim, M., Li, Y., et al. (2003). Noncovalent Functionalization of Carbon Nanotubes for Highly Specific Electronic Biosensors. Proc. Natl. Acad. Sci. USA, 100:4984–4989.
- Chen, S. C., Wang, J., Fissan, H., and Pui, Y. H. D. (2013a). The Use of Nuclepore Filters for Ambient and Workplace Nanoparticle Exposure Assessment—Spherical Particles. Atmos. Environ., 77:385–393.
- Chen, S. C., Wang, J., Fissan, H., and Pui, Y. H. D. (2013b). Exposure Assessment of Nanosized Engineered Agglomerates and Aggregates Using Nuclepore Filter. J. Nanoparticle Res., 15:1955–1969.
- Cheng, Y. S., Holmes, T. D., and Fan, B. (2006). Evaluation of Respirator Filters for Asbestos Fibers. J. Occup. Environ. Hyg., 3:26–35.
- Dahm, M., Yencken, M., and Schubauer-Berigan, M. (2011). Exposure Control Strategies in the Carbonaceous Nanomaterial Industry. J. Occup. Environ. Med., 53:S68–S73.
- Federal Register. (2010). Multi-Walled Carbon Nanotubes and Single-Walled Carbon Nanotubes Significant New Use Rules. Federal Register, 75(180), September 17, 2010 / Rules and Regulations.
- Federal Register. (2011). Multi-Walled Carbon Nanotubes Significant New Use Rule. Federal Register, 76(88), May 6, 2011 / Rules and Regulations.
- Federal Register. (2013). Functionalized Multi-Walled Carbon Nanotubes Significant New Use Rule. Federal Register, 78(90), May 9, 2013/ Rules and Regulations.
- Hu, H., Zhao, B., Itkis, M. E., and Haddon, R. C. (2003). Nitric Acid Purification of Single-Walled Carbon Nanotubes. J. Phys. Chem. B, 107:13838–13842.
- Kanaoka, C., Emi, H., Otani, Y., and Iiyama, T. (1987). Effect of Charging State of Particles on Electret Filtration. Aerosol Sci. Technol., 7:1–13.
- Kim, S. C., Wang, J., Emery, M., Shin, W. G, Mulholland, G., and Pui, D. Y. H. (2009). Structural Property Effect of Nanoparticle Agglomerates on Particle Penetration Through Fibrous Filter. Aerosol Sci. Technol., 43:344–355.
- Kim, S. H., Mulholland, G. W., and Zachariah, M. R. (2007). Understanding Ion-Mobility and Transport Properties of Aerosol Nanowires. J. Aerosol Sci., 38:823–842.
- Lathrache, R., and Fissan, H. J. (1986). Enhancement of Particle Deposition in Filters due to Electrostatic Effects, in Extended Abstracts, 4th World Filtration Congress, Filtration Society, Antwerpen, Belgium, pp. 7.55–7.63.
- Lathrache, R., Fissan, H. J., and Neumann, S. (1986). Deposition of Submicron Particles on Electrically Charged Fibers. J. Aerosol Sci., 17:446–449.
- Li, L., Zuo, Z., Japuntich, D. A., and Pui, D. Y. H. (2012a). Evaluation of Filter Media for Particle Number, Surface Area and Mass Penetrations. Ann. Occup. Hyg., 56:581–594.
- Li, M., Mulholland, G. W., and Zachariah, M. R. (2012b). The Effect of Orientation on the Mobility and Dynamic Shape Factor of Charged Axially Symmetric Particles in an Electric Field. Aerosol Sci. Technol., 46:1035–1044.
- Manton, M. J. (1978). The Impaction of Aerosols on a Nuclepore Filter. Atmos. Environ., 12:1669–1675.
- Manton, M. J. (1979). Brownian Diffusion of Aerosols to the Face of a Nuclepore Filter. Atmos. Environ., 13:525–531.
- Mostofi, R., Wang, B., Haghighat, F., Bahloul A., and Jaime, L. (2010). Performance of Mechanical Filters and Respirators for Capturing Nanoparticles-Limitation and Future Direction. Ind. Health, 48:296–304.
- Muller, J., Delos, M., Panin, N., Rabolli, V., Huaux, F., and Lison, D. (2009). Absence of Carcinogenic Response to Multiwall Carbon Nanotubes in 2-year Bioassay in the Peritoneal Cavity of the Rat. Toxicol. Sci., 110:442–448.
- NIOSH (1995). Approval of Respiratory Protective Devices, Code of Federal Regulations Title 42, Part 84. NIOSH, Cincinnati, OH, USA. Available at: http://www.cdc.gov/niosh/npptl/resources/certpgmspt/pdfs/SAPJul2005.pdf
- NIOSH (2009). Approaches to Safe Nanotechnology: Managing the Health and Safety Concerns with Engineered Nanomaterials. DHHS (NIOSH) Publication No. 2009-125. U.S. Department of Health and Human Services, Centers for Disease Control, National Institute for Occupational Safety and Health, Cincinnati, OH.
- NIOSH (2013). NIOSH Current Intelligence Bulletin 65: Occupational Exposure to Carbon Nanotubes and Nanofibers. DHHS (NIOSH) Publication No. 2013-145. U.S. Department of Health and Human Services, Centers for Disease Control, National Institute for Occupational Safety and Health, Cincinnati, OH.
- Ozkan, Y., and Bradford, P. D. (2013). Aligned Carbon Nanotube Sheet high Efficiency Particulate Air Filters. Carbon, 64:295–304.
- Poland, C. A., Duffin, R., Kinloch, I., Maynard, A., Wallace, W. A. H., Seaton, A., et al. (2008). Carbon Nanotubes Introduced into the Abdominal Cavity of Mice Show Asbestos-Like Pathogenicity in a Pilot Study. Nat. Nanotechnol., 3:423–428.
- Rengasamy, S., Eimer, B. C., and Shaffer, R. (2009). Comparison of Nanoparticle Filtration performance of NIOSH-Approved and CE-Marked Particulate Filtering Facepiece Respirators. Ann. Occup. Hyg., 53:117–128.
- Rengasamy, S., King, W. P., Eimer, B. C., and Shaffer, R. (2008). Filtration Performance of NIOSH-Approved N95 and P100 Filtering Facepiece Respirators Against 4 to 30 Nanometer-Size Nanoparticles. J. Occup. Environ. Hyg., 5:556–564.
- Seto, T., Furukawa, T., Otani, Y., Uchida, K., and Endo, S. (2010). Filtration of Multi-Walled Carbon Nanotube Aerosol by Fibrous Filters. Aerosol Sci. Technol., 44:734–740.
- Shin, W. G., Wang, J., Mertler, M., Sachweh, B., Fissan, H., and Pui, D. Y. H. (2010). The Effect of Particle Morphology on Unipolar Diffusion Charging of Nanoparticle Agglomerates in the Transition Regime. J. Aerosol Sci., 41:975–986.
- Shvedova, A. A., Kisin, E., Murray, A. R., Johnson, V. J., Gorelik, O., Arepalli, S., et al. (2008). Inhalation versus Aspiration of Single Walled Carbon Nanotubes in C57BL/6 mice: Inflammation, Fibrosis, Oxidative Stress and Mutagenesis. Am. J. Physiol., Lung Cell Mol. Physiol., 295:L552–565.
- Spurny, K. R., Lodge, J. P., Frank, E. R., and Sheesley, D. C. (1969). Aerosol Filtration by Means of Nuclepore Filters: Structural and Filtration Properties. Environ. Sci. Technol., 3:453–464.
- Sun, Y. P., Fu, K., Lin, Y., and Huang, W. (2002). Functionalized Carbon Nanotubes: Properties and Applications. Acc. Chem. Res., 35:1096–1104.
- Tuinman, I. L. (1999). The Influence of Particle Shape on the Penetration through Fibrous and Electret Filters, in Electret Filters, Production and Properties, J. I. T. Stenhouse, L. Gradon, and J. C. M. Marijnissen, eds., Delft University Press, The Netherlands, pp. 119–128.
- Vo, E., and Zhuang, Z. (2013). Development of a New Test System to Determine Penetration of Multi-Walled Carbon Nanotubes through Filtering Facepiece Respirators. J. Aerosol Sci., 61:50–59.
- Wang, J. (2013). Effects of Particle Size and Morphology on Filtration of Airborne Nanoparticles. KONA Powder Particle J., 30:256–266.
- Wang, J., Chen, D. R., and Pui, D. Y. H. (2007). Modeling of Filtration Efficiency of Nanoparticles in Standard Filter Media. J. Nanoparticle Res., 9:109–115.
- Wang, J., Kim, S. C., and Pui, D. Y. H. (2011a). Measurement of Multi-Wall Carbon Nanotubes Penetration Through a Screen Filter and Single-Fiber Analysis. J. Nanopart. Res., 13:4565–4573.
- Wang, J., Kim, S. C., and Pui, D. Y. H. (2011b). Carbon Nanotube Penetration Through a Screen Filter: Numerical Modeling and Comparison with Experiments. Aerosol Sci. Technol., 45:443–452.
- Wang, J., and Tronville, P. (2014) Toward Standardized Test methods to Determine the Effectiveness of Filtration Media against Airborne Nanoparticles. J. Nanoparticle Res., 16:2417–2449. DOI: 10.1007/s11051-014-2417-z.
- Wen, H. Y., Reischl, G. P., and Kasper, G. (1984). The Model of Bipolar Diffusion Charging of Fibrous Aerosol Particles—I. Charging Theory. J. Aerosol Sci., 15:89–101.
- Zhang Y., Bai, Y., and Yan, B. (2010). Functionalized Carbon Nanotubes for Potential Medicinal Applications. Drug Discov. Today, 15:428–435.
- Zhao, X. and Liu, R. (2012). Recent Progress and Perspectives on the Toxicity of Carbon Nanotubes at Organism, Organ, Cell, and Biomacromolecule Levels. Environ. Int., 40:244–256.