Abstract
A procedure is described to electrospray the relatively large ions tetrahexadecylammonium (C16H33)4N+ and tetraoctadecylammonium (C18H37)4N+ from their commercially available bromide salts. Their low solubility and the corresponding low conductivity of their alcohol solutions complicates their electrospraying by the convenient method of applying the high voltage to the solution reservoir (rather than to the electrospraying tip). If the capillary bore is widened to reduce the reservoir-tip electrical resistance, the solvent evaporates at its tip, leading to solute precipitation and spray destabilization. These difficulties are overcome via conventional nanospray: we use a wide (∼100 μm ID) capillary whose tip is pulled in a flame down to <20 μm OD. Mobility spectra result where the dominant and most mobile peaks are (C16H33)4N+ or (C18H37)4N+. Their electrical mobilities in air at room temperature and pressure are 0.63 and 0.60 cm2/V/s (±1%).
Copyright 2015 American Association for Aerosol Research
1. INTRODUCTION ON LARGE ION STANDARDS
Electrosprayed tetraalkylammonium ions (of composition (CnH2n+1)4N+) have been used as mobility standards for drift tube ion mobility studies coupled to mass spectrometry (IMS-MS: Viidanoja et al. Citation2005; May et al. Citation2014) and aerosol instrument calibration (Ude and Fernández de la Mora Citation2005; Steiner et al. Citation2010; Jiang et al. Citation2011a,Citationb; Kangasluoma et al. Citation2013). Since many DMAs used in aerosol research (Knutson and Whitby Citation1975) are run at moderate Reynolds numbers (Re < 2000, typically much less), they have limited resolving power with ∼1 nm particles. Therefore, the larger the standard, the easier it would be to resolve one of these ions in the generally complex mobility spectrum of an electrospray. Also, even for supercritical DMAs developed for high-resolution analysis of small ions, clusters and nanoparticles smaller than 3–5 nm (Rosser and Fernández de la Mora Citation2005; Fernández de la Mora Citation2011a; Attoui et al., Citation2013; Fernández de la Mora and Kozlowski Citation2013), the resolving power is difficult to probe at the highest voltages attainable due to lack of monomobile standards of low mobility (Attoui et al. Citation2013). This problem is particularly acute in the case of long supercritical DMAs able to classify not just 1–3 nm particles, but covering also singly charged particles with diameters up to 30 nm and beyond. Furthermore, pure ion standards are of considerable interest in other applications, including ion-induced nucleation studies (Fernández de la Mora Citation2011b; Winkler et al. Citation2012). There is accordingly an interest in identifying monomobile ion standards of increasingly large sizes. The fact that tetra-alkylammonium ions provide a chemically homogeneous commercially available series of nanoparticles going at least from n = 1 to n = 18 is particularly useful when studying size effects, for instance in ion induced nucleation research. However, the salts of the larger members of this series are far less soluble than those more commonly used [up to (C12H25)4N+], which poses certain practical electrospraying issues presently addressed.
Although (C16H33)4N+Br− and (C18H37)4N+Br− salts are almost insoluble in conventional electrospraying (polar) solvents, their solubility in alcohol (methanol-propanol) is sufficient to have already led to their use as mass and mobility standards in tandem mobility mass spectrometry (IMS-MS) instruments (May et al. Citation2014; based on a 50% methanol/50% isopropanol solvent). In these instruments, the high resolution of the MS permits observing trace quantities of solutes, as well as distinguishing them from other far more abundant species even in rather complex mixtures. May et al. (Citation2014) record the mobilities in N2 of 594 different substances producing singly charged ions, including carbohydrates, lipids, and peptides with molecular masses m going up to 2147.7 g/mol. Interestingly, the tetraalkylammonium ion series offers the largest size for a given mass, such that the mobility of (C18H37)4N+ (m = 1027.16 g/mol) is almost as low as the lowest in their list. The ions (C16H33)4N+ and (C18H37)4N+ considered here are therefore ideal from the point of view of providing a relatively large mobility standard.
In view of the fact that the practical use of these large molecular ions in aerosol applications (not enjoying the benefit of a MS) would require a relatively strong and isolated peak, in preliminary experiments, we used just a DMA to examine the electrosprays from a saturated solution of (C16H33)4N+Br− in methanol. As shown in , the spectrum is dominated by (C16H33)4N+, which is also the most mobile ion present. The practical difficulty, however, is in forming an electrospray in the common setup in which the high voltage is applied to the solution. Two factors are at play here: the high electrical resistance of the capillary line and the precipitation of the sparingly soluble salt at the capillary tip. First, the electrical current must reach the cone tip by conduction through the liquid within the capillary tube, which, when the liquid has very low electrical conductivity, requires either unconventionally high voltages or wide capillary bores. Second, wide capillary tips are generally undesirable because liquid flow rates are typically small, hence solvent evaporation from the meniscus is substantial. This is particularly inconvenient in the case of modestly soluble solutes, which tend to precipitate at the tip, complicating the spraying process, and eventually clogging the capillary. There are two remedial solutions to the conductivity problem of narrow bore capillaries. It is possible to add other salts to the solution, but at the cost of forming a more complex pattern of ions. Alternatively, one may use a conducting capillary and apply the high voltage directly at its tip. Unfortunately metallic capillaries with tip diameters as small as those obtainable with silica (∼20 μm) are not commercially available. Finally, the tip of a capillary of silica or glass may be made conductive by depositing a metallic or semiconducting oxide layer. But this adds complexity to the already nontrivial process of obtaining a good spraying tip.
FIG. 1. Mobility spectrum for a spray of tetrahexadecylammonium bromide, showing on its right boundary the sharp peak associated to (C16H33)4N+.
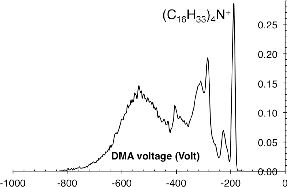
Here we spray (C16H33)4N+ and (C18H37)4N+ salts by following an approach suggested by so-called nanoelectrospray (Wilm and Mann Citation1996). NanoES relies on glass or silica capillaries with a wide bore, whose tip is pulled under a torch down to a narrow bore (<20 μm). The resulting narrowing region of the capillary is then short enough to produce a modest resistance to conduction through the liquid, even with de-ionized water or high purity alcohols. The approach is beneficial also in the case of reasonably soluble salts such as (C7H15)4N+Br−, as it enables spraying them at relatively low concentrations.
2. EXPERIMENTAL
2.1. Chemicals
(C16H33)4N+Br− and (C7H15)4N+Br− were both obtained from Fluka. (C18H37)4N+Br− was from Alfa Aesar. One or several grains about 0.1 mm in diameter of either (C16H33)4N+Br− or (C18H37)4N+Br− were dropped in a ½ cm3 vial, then filled with methanol (JT Baker, HPLC grade) or ethanol (200 proof, Decon labs Inc). No visible reduction of the grain size was observed in methanol after shaking the solution for 5 min in an ultrasonic bath. This saturated solution did not electrospray stably (even when applying 6 kV to the solution) with a silica capillary 40 μm in diameter, but sprayed readily at ∼1 kV via a pulled capillary with initial ID of 170 μm. However, salt deposits formed at the tip when spraying over periods of a few minutes, which eventually made it hard to maintain a steady current over practical times required for mobility analysis. Accordingly, the saturated solution was diluted by a factor between 4 and 10 in either methanol or ethanol. The methanol-diluted solution provided a sufficiently steady spray for acquiring mobility spectra, though some salt deposition still took place at the capillary tip and had to be washed periodically (every 10–15 min) with pure methanol. Dilution of the original solution with ethanol reduced further the precipitation problem. In subsequent experiments, the salt grain was initially dropped in ethanol (rather than methanol), leading to an apparently smaller tendency to precipitate at the tip.
2.2. Capillaries
We relied on commercial silica capillaries, 360 μm OD, 170–70 μm ID (Polymicro), which may be conveniently connected to various components via commercial liquid chromatography connectors (Upchurch). One such connector was attached to each end of the capillary, which was suspended vertically by tying the upper end to a rigid structure, and the lower end to an approximately axisymmetric weight (∼0.5 lb), such that the capillary would be under dominantly axial tension. A propane torch was then directed to the lowest end of the capillary, as close to the connector as possible without burning it. When appropriately hot the capillary would be pulled by the weight and divided into two pieces. The lowest one was separated from the weight and discarded. The longer piece was typically pulled by the above process to a diameter smaller than desired, or even completely closed. It was accordingly examined under a microscope and cut (with the sharp edge of an alumina plate) at the appropriate location, so as to achieve the desired tip diameter. No final mechanical sharpening is necessary for small tip diameters (<10 μm), which are in any case too delicate for this operation. Wider tips (>20 μm) having a visible wall thickness were sharpened by contact between the capillary tip (rotated manually) against a flat alumina surface supported on a rotating table. Thicker wall capillaries with 70 μm ID are more likely to survive this sharpening than thinner wall capillaries with 170 μm ID. These pulled conical tips are mechanically delicate because the original protective polymer layer is destroyed in the flame. Furthermore, the conical bore tends to clog by trapping any particles smaller than the tip ID accidentally introduced in the liquid through the wide end of the capillary. This incidence may, however, be repaired in the case of organic particles (which tend to be deformable and are particularly effective in eventually obstructing the flow) by introducing the tip in a moderately hot flame while pushing a water flow through the capillary from the cold end. It is preferable to use a cigarette lighter than a torch, as the thin tip tends to be curved when heated either for too long or to too high a temperature. For a pulled ID of ∼20 μm, as soon as the tip is unclogged, a moderate pressure of water leads to the formation of a long jet visible to the naked eye. Spraying from these capillaries (unclogged) requires only a small pressure drive, the regime of the spray being monitored via the sprayed current, and controlled primarily through the voltage applied to the solution. Pulled capillaries are also commercially available from New Objective in a variety of inner and outer diameters, lengths and drawn tip diameters (i.e., FS360-100-30-N-5-C20). We have tested one of these commercial Picotips having 100 μm ID and 30 μm pullet tip, with results comparable or superior to those of the home-made capillaries.
2.3. Mobility Analysis
The sprays produced in positive polarity were probed with a previously described differential mobility analyzer with electrode radii R1 = 4 mm, R2 = 7 mm, and axial distance between slits L = 20 mm (Fernández de la Mora and Kozlowski Citation2013). This DMA was modified to incorporate an internal semiconducting outlet tube bringing the monomobile particles collected at high negative voltage in the inner electrode into the grounded sample outlet tube of the DMA. The purpose of this antistatic tube is to increase the sample transmission of the DMA. A second change was meant to suppress an instability arising at high enough sheath gas flow rates, due to the growing boundary layer in this relatively long DMA. This problem was overcome by substituting the original cylindrical inner electrode with one converging at 1o. These two changes will be more thoroughly described in a forthcoming publication. The sheath gas was filtered laboratory air, at its ambient (uncontrolled) humidity. Nonetheless, the high hydrophobicity of (CnH2n+1)4N+ ions with n ≥ 7 greatly reduces their tendency to be solvated with water vapor (Thomson and Iribarne Citation1979). The mobility spectra to be reported in the form of ion signal versus DMA voltage were obtained by fixing the gas flow rates and the electrospray, while scanning over the voltage difference between the two DMA electrodes. The signal of monomobile aerosol is the classified current, obtained by passing the sample flow through a Faraday cage electrometer.
3. RESULTS
shows a mobility spectrum for an ethanol solution of (C18H37)4N+Br− (open circles), compared with a spectrum from a solution of (C7H15)4N+ (filled circles). The dominant ion in the C18 spectrum is the most mobile one, appearing at a voltage 1.619 times larger than that for the dominant (and most mobile) ion in the spectrum of (C7H15)4N+. This ratio differs 1.3% from the corresponding ratio reported by May et al. (Citation2014), a modest disagreement given that their drift gas was dry N2 at 300 K (versus lab air at ∼295 K in our measurements). A less mobile ion of substantial intensity can be seen in the C18 spectrum, but its right and left tails reveal that it is not a pure species.
FIG. 2. Mobility spectra from sprays of (C18H37)4N+Br− (open circles) and (C7H15)4N+Br− ions (filled circles) taken under identical conditions of the DMA. The dominant and most mobile peak in the spray of (C18H37)4N+Br− is (C18H37)4N+.
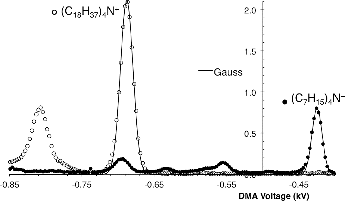
shows four mobility spectra, three of them associated with sprays from the salts (CnH2n+1)4N+Br with n = 7, 16, 18, and a fourth one, labeled Blank, with no electrospray at all. In this figure, prior to their analysis in the DMA, the electrospray ions were introduced into a charge reduction chamber (containing a radioactive beta emitter Ni-63, 10 mCi), where multiply charged ions were largely eliminated. The spectra for the C18 and C16 ions include the main ion, as well as the broader lower mobility peaks seen in and . These features therefore correspond to unidentified singly charged ions. In this case, the ratios of main peak voltages to that for tetraheptylammonium in air at about 295 K are 1.621 and 1.534 (compared to 1.641 and 1.552 in dry N2 at 300 K, given by May et al. Citation2014). Therefore, both in naturally charged as well as in charge-reduced sprays of (C18H37)4N+Br− and (C16H33)4N+Br−, the easily discernible dominant and most mobile peaks are (C18H37)4N+ and (C16H33)4N+. The electrical mobilities of these two ions are collected in , where the mobility ratios just quoted are turned into actual mobilities based on the (C7H15)4N+ standard previously reported by Ude and Fernández de la Mora (Citation2005).
Table 1 Measured electrical mobilities Z in laboratory air based on the (C7H15)4N+ ion standard
FIG. 3. Mobility spectra after passing the electrospray ions through a charge-reduction chamber containing radioactive Ni-63.
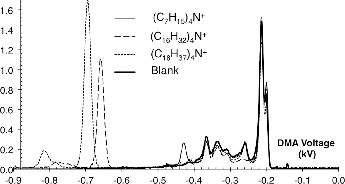
In view of the fact that radioactive sources are relatively common in aerosol laboratories, the use of a neutralizer illustrated in deserves some remarks. While the electrosprays of tetraalkylammonium salts give an easily recognizable most mobile and most intense peak in the spectrum, this peak tends be accompanied on its low mobility end by singly and multiply charged salt clusters. In the case of (C7H15)4N+Br−, this contamination is not problematic at sufficient DMA resolution because the first and second major peaks (monomer and dimer) differ in mobility by a ∼1.5 factor. Still, many DMAs conventionally used in aerosol laboratories cannot be run at high enough sheath gas flow rates to adequately separate these two ions. A method to clean up the low mobility portion of the spectrum is therefore of interest. Notice in this respect that the (C7H15)4N+ peak in does not show any contamination to its left, other than the background associated to neutralizer ions. This interesting situation is achieved by using relatively small quantities of (C7H15)4N+Br− in the solution, so that the dimer and trimer contributions become minimal. Such conditions do not generally suffice to clean up the low mobility end of the spectrum, due to the formation of multiply charged ions (). However, combining a low salt concentration (∼1 μM) with a neutralizer removes singly charged multimers (due to the low salt concentration) as well as multiply charged clusters (due to the neutralizer). The price to be paid for this clearing of the low mobility region is the appearance of numerous high mobility ions produced in the neutralizer. In , this neutralizer background extends to unusually low mobilities, even beyond that of (C7H15)4N+. The undesirable neutralizer peaks having the smallest mobilities result from relatively large ionized neutral vapor contaminants. By cleaning the neutralizer and the lines from the gas supply to the working region of the DMA, and by using purer gases, these heavy contaminants can be largely eliminated, pushing the neutralizer ions to substantially higher mobilities. Furthermore, neutralizer ions are of lesser concern for the two larger salts investigated here. Note finally that a considerable reduction of the salt concentration is not necessarily damaging to the intensity of the main ionic peak. In fact, decreasing this concentration from 1 mM down to a few μM initially decreases the intensity of dimers and trimers, but increases considerably the intensity of the monomer. The key to this behavior is the known surface activity of these tetraalkylammonium salts. Most of the charge on the original drops is transferred into smaller daughter drops forming during the many stages of Coulombic explosions experienced by an evaporating drop. These secondary drops are produced by a Taylor cone forming on the surface of the primary drop, and carry preferentially surface material. As a result, surface active solutes are preferentially ionized over other solutes. One would therefore expect that if the quantity of tetraalkylammonium salt initially in one electrosprayed drop is sufficient to consume most of the initial drop charge, it will do so. A larger salt concentration would be counterproductive due to competition from salt clusters for the limited available stock of charge.
4. CONCLUSIONS
A simple procedure to produce DMA-resolvable isolated peaks of relatively low mobility by electrospraying (CnH2n+1)4N+Br− salts has been extended to n = 18, widening the range of sizes of molecular ions of this homologous composition that may be conveniently used as pure nanoparticle standards.
CONFLICT OF INTEREST STATEMENT
Following Yale rules, the author declares his personal interest in the companies SEADM and NanoEngineering Corporation, both commercializing the DMA used in this work.
ACKNOWLEDGMENTS
I am grateful to Professor Michel Attoui (University of Paris, Est Creteil) for many improvements on the DMA setup used, particularly the electrometer and the high transmission semiconductor tubing in the monodisperse aerosol outlet. I am also much indebted to colleagues at SEADM for their gift of the pump running the DMA sheath gas, its precise velocity control system, and for the development of the data acquisition system. Many contributions of Yale students Juan Fernández-García, Matthew Paragano, and Aaron Madden are also gratefully acknowledged.
REFERENCES
- Attoui, M., Paragano, M., Cuevas, J., and Fernández de la Mora, J. (2013). Tandem DMA Generation of Strictly Monomobile 1-3.5 nm Particle Standards. Aerosol Sci. & Tech., 47(5):499–511.
- Fernández de la Mora, J. (2011a). Sub-3 nm Aerosol Measurement with DMAs and CNCs, Chapter 32, pp. 697-721 of Aerosol Measurement: Principles, Techniques, and Applications, 3rd ed. P. Kulkarni, P. A. Baron, and K. Willeke, eds., John Wiley & Sons, Inc.
- Fernández de la Mora, J. (2011b). Heterogeneous Nucleation with Finite Activation Energy and Perfect Wetting: Capillary Theory versus Experiments with Nanometer Particles, and Extrapolations on the Smallest Detectable Nucleus. Aerosol Sci. & Tech., 45(4):543–554
- Fernández de la Mora, J., and Kozlowski, K. (2013). Hand-Held Differential Mobility Analyzers of High Resolution for 1-30 nm Particles: Design and Fabrication Considerations. J. Aerosol Sci., (57):45–53.
- Jiang, J., Attoui, M., Heim, M., Brunelli, N. A., McMurry, P. H., Kasper, G., et al. (2011b). Transfer Functions and Penetrations of Five Differential Mobility Analyzers for Sub-2 nm Particle Classification. Aerosol Sci. & Tech., 45(4):480–492
- Jiang, J., Chen, M., Kuang, C., Attoui, M., and McMurry, P. H. (2011a). Electrical Mobility Spectrometer Using a Diethylene Glycol, Condensation Particle Counter for Measurement of Aerosol Size Distributions Down to 1 nm. Aerosol Sci. & Tech., 45:510–521
- Kangasluoma, J., Junninen, H., Lehtipalo, K., Mikkila, J., Vanhanen, J., Attoui, M., et al. (2013). Remarks on Ion Generation for CPC Detection Efficiency Studies in Sub-3-nm Size Range. Aerosol Sci. & Tech., 47:556–563
- Knutson, E. O., and Whitby, K. T. (1975). Aerosol classification by electric mobility: apparatus, theory and applications. J. Aerosol Sci., 6:443–451.
- May, J. C., Goodwin, C. R., Lareau, N. M., Leaptrot, K. L., Morris, C. B., Kurulugama, R. T., et al. (2014). Conformational Ordering of Biomolecules in the Gas Phase: Nitrogen Collision Cross Sections Measured on a Prototype High Resolution Drift Tube Ion Mobility-Mass Spectrometer. Anal. Chem., 86:2107–2116.
- Rosser, S., and Fernández de la Mora, J. (2005). Vienna-Type DMA of High Resolution and High Flow Rate. Aerosol Sci. & Tech., 39(12):1191–1200
- Steiner, G., Attoui, M., Wimmer, D., and Reischl, G. P. (2010). A Medium Flow, High-Resolution Vienna DMA Running in Recirculating Mode. Aerosol Sci. & Tech., 44(4):308–315.
- Thomson, B. A., and lribarne, J. V. (1979). Field-Induced Ion Evaporation from Liquid Surfaces at Atmospheric Pressure. J. Chem. Phys., 71(11):4451–4463
- Ude, S., and Fernández de la Mora, J. (2005). Molecular Monodisperse Mobility and Mass Standards from Electrosprays of Tetra-Alkyl ammonium Halides. J. Aerosol Sci., 36:1224–1237.
- Viidanoja, J., Sysoev, A., Adamov, A., and Kotiaho, T. (2005). Tetraalkylammonium Halides as Chemical Standards for Positive Electrospray Ionization with Ion Mobility Spectrometry/Mass Spectrometry. Rapid Commun. Mass Spectrom., 19:3051–3055
- Wilm, M, and Mann, M. (1996). Analytical Properties of the Nanoelectrospray Ion Source. Anal. Chem., 68(1):1–8
- Winkler, P. M., Vrtala, A., Steiner, G., Wimmer, D., Vehkamaki, H., Lehtinen, K. E. J., et al. (2012). Quantitative Characterization of Critical Nanoclusters Nucleated on Large Single Molecules. Phy. Rev. Lett., 108(8):085701